Fig. 5.1
(a) Intercalary reconstruction of the tibia by using an allograft after resection of an osteosarcoma. Note the holes for patellar tendon reattachment. (b) In this case, preservation of the epiphysis allowed the patient to do sporting activities such as climbing
The growth plate has traditionally been considered to block the spread of a tumor, but this barrier is not impenetrable. In limb salvage procedures, although preserving the joint near the tumor enables better functional results, it is not possible to preserve the epiphysis in all cases. Articular resections pose the most complex problems of reconstruction in oncological surgery [15].
Magnetic resonance imaging (MRI) is an important tool in limb salvage procedures. Plain radiographs only indirectly show growth problems caused by physeal abnormalities and they do so long after damage has occurred. MRI, however, provides the resolution necessary to define many of the prenatal and postnatal stages of epiphyseal development in vivo. Differentiation between the different layers of the cartilage is well achieved with gadolinium enhancement that also identifies cartilage vascular canals. With MRI it is possible to differentiate epiphyseal cartilage, physeal cartilage, the zones of provisional calcification, physis of the secondary center of ossification, the perichondrium, vascularity of the cartilage canals, and hematopoietic and fatty marrow [6, 33, 50].
5.2 Morphology of Growth Plate
Physeal cartilage is the growth organ of the long bones. The cells of growth cartilage are chondrocytes, which direct the whole process of enchondral ossification and are responsible for controlling the extent of growth in the long bones during postnatal development. Endochondral ossification is a process involving chondrogenesis, chondrocyte hypertrophy, matrix mineralization, and vascularization followed by bone formation [26]. Morphologically, the growth plate is divided into zones of germinal cells, columnar cells, hypertrophic cells, and metaphyseal cells. In terms of function, we can refer to (1) the germinal zone; (2) the columnar zone with two well defined areas: the upper proliferating zone and the lower maturation zone; (3) the hypertrophic cell zone, with the upper 4/5 with a non-mineralized matrix and the lower 1/5 with a mineralized matrix; and (4) the outer reaches of the metaphysis [26].
Although the two most important states of a physeal chondrocyte are proliferation and hypertrophy, which includes the mineralization of the matrix before bone reabsorption during vascular invasion, a chondrocyte actually goes through several phases, each of which is characterized by a predominant functional activity. These phases occur synchronically: the youngest chondrocytes are proliferating cells, whilst the oldest are to be found in regions of vascular invasion. The upper hypertrophic zone is characterized by cells that have enlarged five- to tenfold implying a reduction in matrix volume relative to total tissue volume. The cells in this zone synthesize type X collagen [4], and the mineralized chondrocytes undergo apoptotic cell death [18]. The chondrocytes presumably trans-differentiate to osteocytes (although there is no evidence of in vivo one-step trans-differentiation) and then endothelial cells from the bone collar invade the terminal layer of apoptotic chondrocytes to form vascular channels [7]. These changes require a molecular interaction between vascular structures and cartilage; the two types of molecules involved in this process are proteases and growth factors [38, 48, 55]. In addition, the transition from cartilage to bone is highly regulated by transcriptional factors and local growth factors (Indian hedgehog (Ihh), Sox9, BMPs, and PTHrP) [29, 34, 36, 51, 58].
The mechanical properties of growth cartilage depend on the extracellular matrix, which is produced and maintained by the chondrocytes. In the case of many bone types, epiphyseal plates form, in some individuals, the greatest possible angle with the corresponding planes of shear strain. The space between the two plate surfaces is filled by growth cartilage, which, seen from the front, has a “V” shape. This arrangement minimizes the risk of shear strain separating the plates. Changes in tension in different areas of the periosteum may be a factor that leads to increased growth in certain parts of the physis [37].
In the event of injury that affects only the metaphyseal part of the growth cartilage, increased growth may be a temporary process which results in broadening of the physis. Good metaphyseal vascularization ensures rapid regeneration [39]. The epiphyseal part of the physis comprising the germinal layer is much more sensitive to trauma because such trauma not only disturbs the functional balance of the physis but can also bring about destruction of growth cartilage.
The three main sources of vascular supply to the metaphysis are (1) the feeder artery, which brings blood to the central metaphyseal area and has peripheral branches with small vessels that are distributed along the inside of the spongy bone and contributes up to 80 % of the blood; (2) the arteries penetrating the metaphysis; and (3) the arteries between the bone and the perichondrium. The central longitudinal branches reach the growth cartilage, resulting in a tree-like vascular pattern. A considerable number of metaphyseal vessels deriving from the articular arteries also contribute to the vascular supply of long bone metaphysis and growth cartilage. All these vessels end in vascular knots or clusters of capillaries just beneath the last intact transverse septum at the base of the cartilaginous part of the growth cartilage [9, 39].
The epiphysis receives its vascular supply from one or more vessels which penetrate the cartilage and branch out inside it. The epiphyseal vascular supply to growth cartilage is characterized by small arterial branches coming from the intra-epiphyseal arteries, which ramify to irrigate the proliferative layer of growth cartilage. However, arterial branches of the epiphyseal vessels do not extend into the cartilaginous part of the physis [26], and so the hypertrophic layer of growth cartilage is free of vascularization. The two most important circulatory systems, constituted respectively by epiphyseal and metaphyseal vessels, are separated both functionally and morphologically [13] (Fig. 5.2).
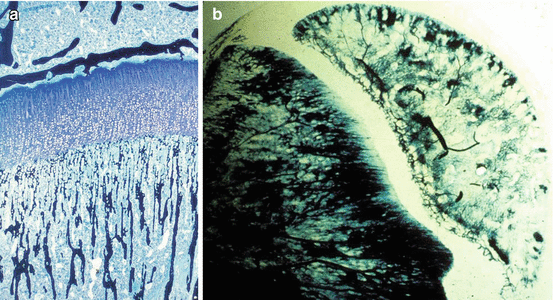
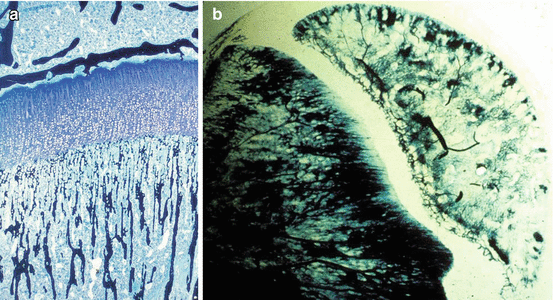
Fig. 5.2
(a) Indian ink injection and (b) Spaltenholz technique showing no vascular anastomoses between metaphyseal and epiphyseal vessels
Vascular anastomoses between the epiphyseal and metaphyseal vessels across the growth plate occur physiologically only at the time of growth plate fusion at skeletal maturity [57].
Vascular invasion in general leads to the removal of cartilage, formation of bone marrow, and new bone formation and growth.
5.3 Physeal Distraction in Orthopedic Surgery
Progressive lengthening is currently a common therapeutic method. Its history can be traced back to pioneering work in 1905 by Codivilla [16], whose technique was successively modified to make it simpler and more convenient for the patient, reducing the duration of treatment and the incidence of complications.
Distraction of the growth plate, physeal distraction or chondrodiastasis is a technique that has been used for bone lengthening and correction of angular deformity [11, 12, 30, 47] (Fig. 5.3). In animal models, growth plate distraction at a slow rate increases length by hyperplasia without causing epiphysiolysis; there is an increase in physeal cell activity demonstrable in the proliferating cell layer [3, 8, 17, 19, 35, 46, 52, 53]; Arriola et al. [5] found an increase in extension of the hypertrophic cell layer; and it has been suggested that the increase in physeal height may be caused by an increase of the metaphyseal blood supply [1, 2, 5, 17, 19, 21, 52]. Interruption of the metaphyseal vascular supply results in inhibited resorption in addition to a shortage of calcium in the hypertrophic chondrocytes.
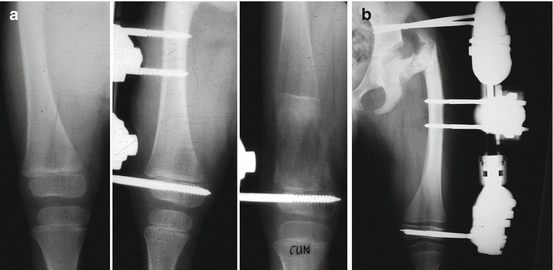
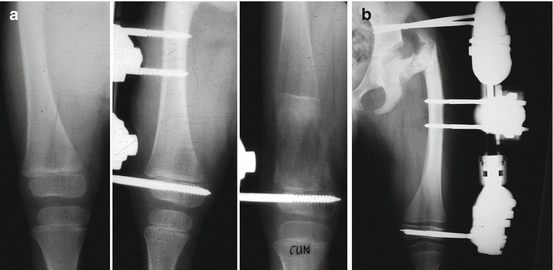
Fig 5.3
Physeal distraction has been widely used in orthopedics (a, b) as a bone lengthening procedure
When forces are applied in traction, epiphysiolysis (of type I according to Salter and Harris’s classification) is always achieved. When diaphyseal-epiphyseal distraction is performed in a growing bone, breakage occurs in the zone of least resistance, that is, in the physis (Fig. 5.4). The fracture makes it possible to lengthen the bone in a single surgical operation and achieve rapid consolidation without the need for osteotomy or even section of the skin.
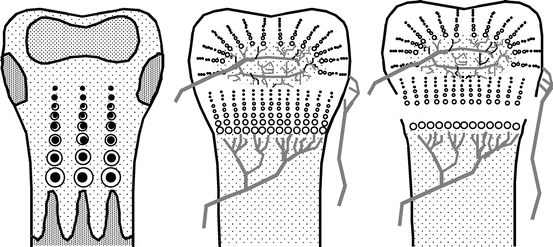
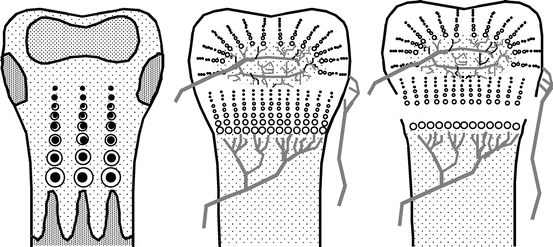
Fig. 5.4
Diagram showing the rupture in the metaphyseal part of a growth plate on application of the physeal distraction technique
Fjeld and Steen [24] established that daily distraction of 0.25 % of bone length, over a period of between 5 and 9 days, brings about epiphyseal separations in animal models. In all experimental studies on physeal distraction, separation of the growth cartilage from the metaphysis has been observed [31, 32, 45, 49].
In the course of the separation process, the periosteum breaks where the perichondrial ring inserts. This break produces a hematoma which fills the gap [23, 31, 32] and which is later replaced by fibrous tissue [40–42]. Newly formed bone deposition proceeds from the intact periosteum as well as from the epiphyseal and metaphyseal portions of the bone [40–42, 49, 54]. The characteristic pattern of grooves in the lengthening area, which can be seen by radiology, reflects the mineralization of the collagen fibers, which are arranged lengthways. Occasionally, a radiolucent area can be observed in the lengthening callus.
De Pablos and Cañadell [20, 21] demonstrated that distraction always produces epiphysiolysis, irrespective of the daily distraction rate. Their research established a relationship between morphological variations in the growth cartilage and the rate of distraction used. In sheep femora subject to lengthening at 0.5 mm/day, the cartilage remained normal (Fig. 5.5). However, femora lengthened by 1 mm/day, and particularly those lengthened by 2 mm/day, were found to have obvious injuries 45 days and 4 months later. Sledge and Noble [52] found that stimulation of the growth cartilage by applying distractional forces of 1–2 kg on the distal femoral physis in rabbits resulted in hyperplasia of the physis, with an increase in cell mitosis and a rise in polysaccharide sulfate synthesis. Spriggins et al. [53] used fixators equipped with instrumentation to study the forces of physeal distraction in rabbits. They detected two patterns of behavior. In one group of cases, the forces increased to maximum values of 20–32 N and then decreased until the next distraction. This indicates breakage of the growth cartilage, with the associated hyperplasia. In the other group of cases, lower forces with maximum values of 6–18 N were observed at the end of the distraction period. This indicates hyperplasia without bone fracture. Tercedor et al. [56] observed that in all physeal distractions there is an initial hyperplasic reaction in all the cell layers of the growth cartilage, and that this is followed by atrophy.
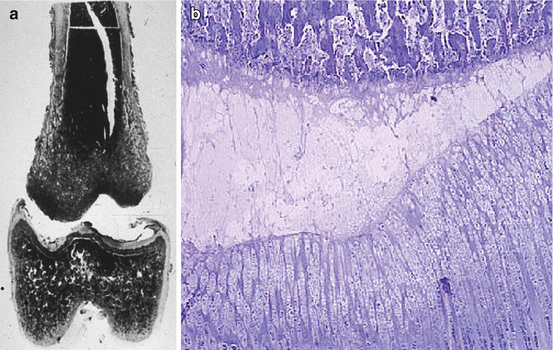
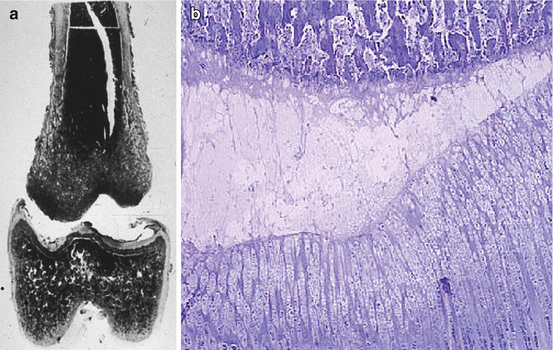
Fig. 5.5
These two histological images of an experimental epiphysiolysis in a lamb show the (a) disruption is produced through (b) the layer of degenerative cells of the growth plate. Most of the growth plate remains together with the epiphysis
The so-called Cañadell technique is applied in certain cases of metaphyseal bone tumor in which there has been no invasion of the physis by the tumor and concerns physeal distraction of the distal extreme of the femur or the proximal end of the tibia. Cañadell’s technique is indicated for pediatric bone sarcomas located in the metaphysis. The physis has to be open and the tumor must not have transgressed the physis [15]. If the tumor is in contact with part of the physis, physeal distraction can be tried, but because it is possible that tumor cells have crossed the physis, intraoperative histology is recommended [13]; if tumor cells are found in the physeal margin of the resection, surgical treatment is to be completed by transepiphyseal or epiphyseal resection. When the tumor has crossed the physis or if the tumor is in contact with all of the physis, the technique is contraindicated [15].
5.4 Technical Aspects of Physeal Distraction with a Unilateral External Fixator
The external fixator is of proven effectiveness in the treatment of fractures. As a consequence of direct experience, development and new designs, the indications for the external fixator have broadened far beyond the original basic indications. Nowadays, the external fixator is used by many departments in the treatment of limb fractures, as well as in cases of retarded consolidation, pseudoarthrosis, axial corrections and in limb lengthening.
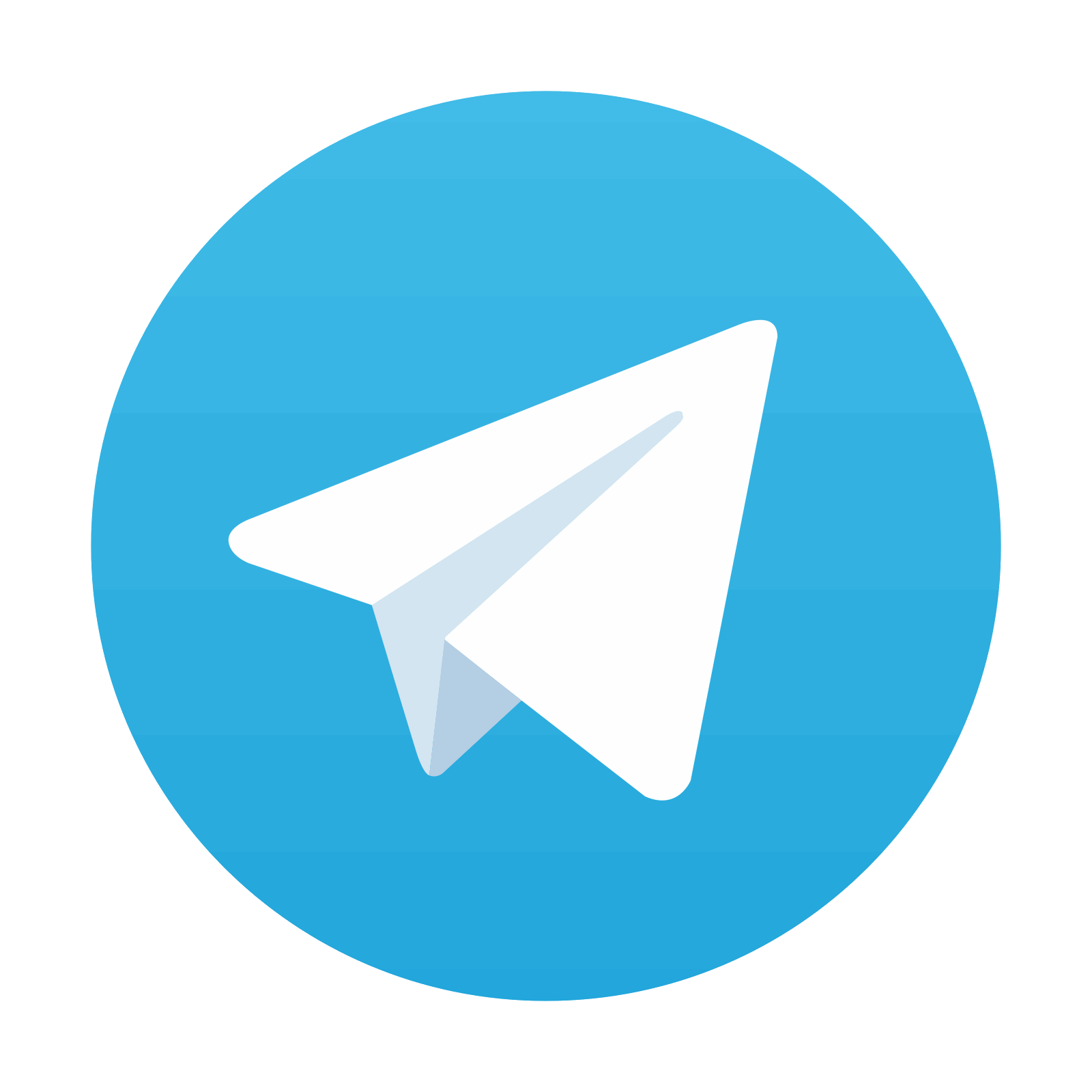
Stay updated, free articles. Join our Telegram channel

Full access? Get Clinical Tree
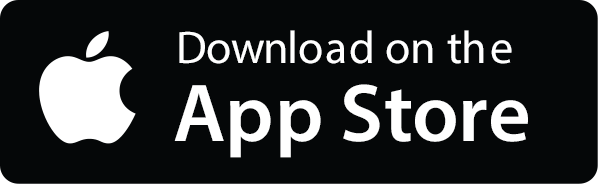
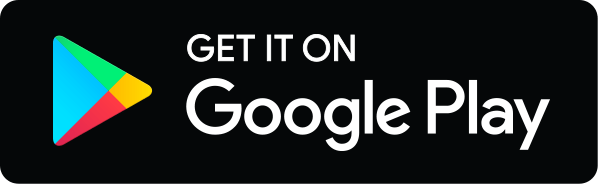