Figure 33.1 Alternative options for fashioning of the recipient hepatic veins for implantation. Either all three hepatic veins are used as a common patch, the RHV is closed or the LHV and MHV are fashioned into a common orifice with a transverse caval extension.
Figure 33.2 Graphical representation of cavoplasty that facilitates piggyback liver transplant and potentially reduces the risk of venous outflow complications.
• SHVC anastomosis – This is the first step in the standard liver (caval) replacement and is performed with a continuous 3/0 or 4/0 polypropylene suture. In the infant where the IVC is narrow, the anterior wall should be closed with interrupted sutures.
• IHVC anastomosis – This is performed next, leaving the anterior wall incomplete in order to flush the liver with 500 mL of normal saline or albumin via the portal vein to wash out hyperkalaemic preservation solution. Complete the IHVC anastomosis at the end of the flush, leaving a growth factor of about one-fourth the diameter of the vessel. Again, in smaller children the anterior layer of the anastomosis should be completed with interrupted sutures.
• Portal vein anastomosis – This is performed taking care to get the length right to avoid kinking or stretching. The anastomosis is performed with a continuous polypropylene suture with a growth factor of about one-fourth to one-third the diameter of the vessel, as described by Starzl et al. [13]. This technique is important in preventing stenosis, but again, in smaller children an alternative is to use interrupted sutures for the anterior layer. The vein should be flushed to ensure that there are no clots within just before completing the anterior layer. Vascular clamps are then removed sequentially from the SHVC, IHVC and portal vein, and the liver is revascularised with portal blood. A search is made for any bleeding points before arterial reconstruction. Care should be taken to see that the ‘growth factors’ of both the IHVC and the portal venous anastomosis are taken up as the vessels expand with the restoration of flow.
Figure 33.3 Intraoperative photograph demonstrating the vertical cavostomy that has been fashioned on the recipient cava in preparation for caval anastomosis in a cavo-cavoplasty piggyback transplant.
The gallbladder is removed retrogradely using diathermy at this time, taking care to identify any anomalous biliary anatomy, such as a low insertion of the right posterior hepatic duct.
• Hepatic artery anastomosis – Flush the donor hepatic artery with heparin saline to remove air and blood clots and apply a bulldog clamp to prevent retrograde flow of blood from the donor liver that may interfere with the anastomosis. An effort is usually made to anastomose the hepatic artery using branch patches [14,15]. The anastomosis is performed under magnification (2.5× to 4.5×) or using an operating microscope with interrupted double-ended 7/0 or 8/0 polypropylene sutures. Care must be taken in preparing the artery for anastomosis by dissecting adventitial tissue away from the edge of the cut margins. Anastomosis of the posterior wall is performed starting with two adjacent sutures in the middle of the back row and working around to the sides where stay sutures are utilised. Following completion of the anterior layer, the stay sutures are tied and the artery reperfused. There is no clear-cut evidence to show that microvascular techniques improve patency rates for hepatic arterial anastomosis, but dedicated microvascular surgeons are used by some units with excellent outcomes. On completion of the anastomoses, the vasculature needs to be assessed intraoperatively with Doppler ultrasound prior to abdominal closure. The best indicator for widely patent hepatic veins is triphasic flow pattern on Doppler examination. Good portal venous flow should be confirmed by the absence of high-velocity jet and turbulence. The hepatic artery waveform should show a good ‘upstroke’, with the absence of a parvus tardus waveform* intrahepatically and a high-velocity jet of >2 m/s extrahepatically [16].
• Biliary anastomosis – Perform a duct-to-duct anastomosis with interrupted 6/0 or 7/0 polydioxanone (PDSTM) for whole liver replacement and a Roux-en-Y hepaticojejunostomy in cases of biliary atresia and sclerosing cholangitis, or if there is gross disparity of donor and recipient ducts, and with partial liver grafts. Important determinants of biliary complications are vascularity of the cut edges, tension-free anastomosis and accurate apposition. For larger ducts, a continuous suture may be performed. Stenting of biliary anastomoses has not been shown to significantly decrease the incidence of complications.
33.3.3 Haemostasis and closurea
Complete haemostasis has to be secured before closure. Often, bleeding at this time is secondary to a coagulopathy, and thromboelastography (TEG) will help guide management of blood products. The abdomen is closed with mass closure and a subcuticular suture (e.g. 4/0 polydioxanone) to the skin over a single abdominal drain placed adjacent to the bile duct anastomosis. Closure of the abdomen should only be performed if there is no risk of graft compression. Otherwise, simple skin closure alone or a temporary silastic mesh should be used. The use of cell salvage has led to bloodless surgery becoming a practical proposition and should be routinely employed.
33.4 PARTIAL LIVER GRAFTS (SEE CHAPTER 36)
The use of partial grafts was the solution to both organ shortage and size restriction in transplanting young children. Liver reduction techniques were developed to increase the donor pool available for children and were made possible by the introduction of UW solution, which allows for up to 20 h of cold preservation time [17]. Reduction techniques were based on the segmental anatomy of the liver and produce three grafts of varying size. The left lateral segment (LLS) (segments 2 and 3) comprises 25%–30% of the whole liver and allows a size reduction from donor to recipient of 10:1. Use of a left lobe (segments 1–4) provides a size reduction of 3:1, and the right lobe (segments 5–8) of 1.5:1 (Figure 33.4).
Liver reduction is performed as a bench procedure in the operating theatre. The liver is kept in cold (4°C) UW solution throughout the procedure. The extent of reduction is determined by visual comparison between the donor liver and the recipient hepatic fossa. When using a right or left liver lobe, the transection plane is through the principal plane slightly to the left or right of the MHV, respectively. The biliary and vascular radicles are carefully ligated with fine ties. The structures of the portal triad can be transected within the parenchyma. The donor vena cava is left with the lobe to be used, and the residual liver is discarded and offered for hepatocyte isolation. After the transection is completed, the cut surface is tested for leaks by perfusing the portal vein with UW and any identified vessels are oversewn. Right or left lobes are transplanted in a similar fashion to a whole graft replacement. Familiarity with the techniques of liver reduction led to the development of split and living donor liver transplantation (see Chapters 36 and 37).
Figure 33.4 Segmental anatomy for splitting of the liver to produce the three grafts of the (1) LLS (segments 2 and 3), (2) left lobe (segments 1–4) and (3) right lobe (segments 5–8). A represents the plane of split for a LLS graft. B represents the plane of split of the right or left lobe, with the line of split being adjusted to Cantile’s line according to hemiliver type.
In Europe, more than 10,000 liver transplants have been performed in recipients under 16 years old. of these, approximately 40% have been performed with whole organs. Partial grafts account for 80% and 52% of all liver transplants performed among patients aged 0–2 and 2–15 years old, respectively. Early experience of reduced-size grafts, either left lobe or LLS, subsequently led to split and living donor liver transplants, with both techniques being incorporated into routine clinical practice from 1991 onwards. Roberts et al. analysed data from 6467 liver transplants performed in patients under 30 years from the Scientific Registry of Transplant Recipients–Organ Procurement and Transplantation Network (SRTR-OPTN) database [18]. It was noted that patient and graft survival during the first year after transplant for each donor graft type varied according to the recipient age group. For children of 2 years and under, living donor grafts had a 51% and 30% lower relative risk (RR) of graft failure than deceased donor split (DD-S) and deceased donor full (DD-F), respectively. A similar difference in mortality risk in the same age group favoured recipients of living donor grafts over DD-S (RR = 0.71, p = 0.08). Recipients in the group aged 0–2 years old had higher risk of mortality and graft failure with DD-S livers than DD-F livers (RR = 1.31, p = 0.04 for mortality; RR = 1.42, p < 0.001 for graft failure). For patients aged 2–10 years, the relative risks of mortality and graft loss were higher after DD-F (RR = 1.78, p = 0.02 for mortality; RR = 1.53, p = 0.02 for graft loss) but not after DD-S. In the age group of 11–16 years, a significantly higher relative risk of graft failure was observed after living donor than after DD-F transplant (RR = 3.63, p = 0.0001) or DD-S transplant (RR = 2.87, p = 0.02), although mortality risks were similar for all three donor graft types [18]. Subsequent publications have confirmed the excellent outcomes with living donor liver transplantation (LDLT) in young children, but published data regarding outcomes of children more than 12 years of age remains limited.
Publications of outcomes of LLS reduced-size liver transplant tend to be from the early 1990s, and there are few direct comparisons with those observed after split liver transplant (SLT) or LDLT. A recent study of 251 liver transplants, which included 138 reduced and 30 split, reported 1-year patient and graft survivals that were comparable at 73% and 67%, respectively [19]. In addition, no differences in vascular complications were observed. of note, biliary complications were significantly more common after split when compared with reduced-size grafts (21% vs. 4%, p < 0.0001). The most common biliary complication after SLT was late stricture, in contrast to reduced liver transplant (RLT), which was cut surface bile leak. Patients undergoing SLT had a significantly increased risk of biliary complications compared with those receiving a RLT, but this did not appear to impact on graft or patient survival [19]. A further series conducted by the University of California, Los Angeles (UCLA) compared the outcome of whole and partial grafts in both adult and paediatric recipients. of 442 liver transplants, 284 were whole, 109 were split liver left lateral segment (SL-LLS) and 49 were living donor left lateral segment (LD-LLS). The 10-year patient survival for children was similar for all graft types. Multivariate analysis confirmed that history of previous liver transplant and SL-LLS were independent predictors of reduced survival. Chronic rejection and hepatic artery thrombosis (HAT) were the most common reasons for graft loss [20]. The largest study from Diamond et al. of outcomes after partial liver grafting in children analysed data from the Studies of Paediatric Liver Transplantation (SPLIT) registry and compared the outcome of each variant with that of whole organ transplantation (1183 whole [WLT], 261 split [SLT], 388 reduced [RLT] and 360 live donor [LDLT]) [21]. There was a clear difference in outcomes at 1 year (WLT 93%, RLT 82%, SLT 87% and LDLT 89%) and 4 years (WLT 89%, RLT 79%, SLT 85% and LDLT 85%) after transplant. However, the groups were not strictly comparable in terms of era of transplant, recipient selection and centre. Children receiving a technical variant waited on average 2.3 months less than those receiving a whole liver and tended to be younger. Complications were significantly higher after partial grafts: at 24 months, the incidence of biliary complications were WLT 17.3%, SLT 28.5%, RLT 25.3% and LDLT 40.1%, and vascular complications were WLT 16.5%, SLT 23.8%, RLT 23.5% and LDLT 24.4% [21].
Overall survival after liver transplantation has improved progressively over the past 25 years, from 1-year survival in 1982 of 35% to 85% by 1991, and >90% in the 2000s [22–24] (Table 33.1 and 33.2). The majority of deaths occur within the first 6 weeks. In addition, the introduction of reduced, split and living donor grafts has led to a fall in the number of children dying while awaiting transplantation to less than 5% [25,26].
Early mortality is most commonly due to early graft dysfunction and sepsis [27,28]. Other important complications with an impact on first-year survival are discussed below. In contrast to the adult population, pretransplant nutritional status and morbidity appear to have little impact on survival after paediatric liver transplantation [23]. Age at transplantation appears to have no influence on survival, except in the very young (<3 months of age). Transplantation for acute liver failure is associated with 30%–40% mortality within the first 3 months of transplantation.
33.6 POSTOPERATIVE MANAGEMENT (SEE CHAPTER 40)
The patient is nursed in an intensive care unit and generally will require ventilation overnight to ensure that he or she remains stable during the first 24 h. All vital signs are continuously monitored. Blood loss from the drains is meticulously charted. Fluid replacement has to take account of fluid loss from the drains with like-for-like replacement in the first 48 h. Pressor support is sometimes required in the earlier recovery period, particularly in the unwell child. Serial blood tests to monitor lactate, base excess and pH and liver function (aspartate transaminase [AST]/alanine transferase (ALT) and international normalised ratio [INR]) allow for the assessment of early graft function Any sign of hepatic dysfunction should lead to a Doppler ultrasound to exclude major vascular complication. Nasogastric suction is used for the first day or two until bowel function returns. Feeding tubes may be kept in to ensure that enteral nutrition is reestablished as soon as possible, and if need be, parenteral nutrition can be used. Chest X-ray should be performed daily while in the intensive care unit or high dependency unit.
Blood urea, serum creatinine, electrolytes, calcium, phosphate, magnesium, full blood count and liver function tests are determined daily for the first 2 weeks. An ultrasound should be performed daily for the first 5 days to check for hepatic artery patency. Immunosuppression is achieved with steroids and a CNI (see Chapter 9.30). Tacrolimus has displaced cyclosporine as the most widely used immunosuppressant after liver transplantation. Tacrolimus exposure is monitored by measuring 12-hour trough levels, aiming for a range between 8 and 10 ng/L. By 3 months, trough levels are reduced to 5–8 ng/L. Cyclosporine dosage is adjusted to maintain a whole blood level of between 100 and 300 ng/mL. Once the gastrointestinal function returns, the patient is commenced on oral cyclosporine 10–30 mg/kg/day. Since bile is needed for cyclosporine absorption, the drug may have to be administered both orally and intravenously for a few days in patients with poor bile production or diversion via a T-tube. Once-a-day CNI therapy has also become popular in the belief that it improves compliance, is popular with recipients and may be associated with a lower incidence of renal complications.
33.7 SURGICAL COMPLICATIONS (SEE CHAPTER 9.13)
33.7.1 Primary nonfunction
Primary nonfunction (PNF) is rare in children because of the quality of the grafts being used for transplantation [29]. A variety of factors contribute to nonfunction, including donor factors, such as haemodynamic instability, time on the intensive care unit prior to donation and problems at retrieval, and recipient factors, including technical or immunological complications. Early graft function can be assessed by the presence of recipient haemodynamic stability, early bile production, lactic acidosis and base excess falling to normal levels within 24 h of surgery, and the correction of perioperative coagulopathy.
Table 33.1 Patient and graft survival in paediatric liver transplantation using split deceased grafts
Note: Summary of the published literature on deceased SLT by author and year, according to graft type. Patient and graft survival expressed as a percentage for each year where available. Red, reduced; ERG, extended right graft; NS, not stated.
Table 33.2 Patient and graft survival in paediatric liver transplantation using reduced grafts
Note: Summary of the published literature on deceased RLT by author and year. Patient and graft survival expressed as a percentage for each given year where available.
Daily laboratory monitoring of the international normalised ratio (INR) and liver function tests, particularly AST levels and serum creatinine, is mandatory. Signs of poor graft function include haemodynamic instability, the need for pressor support, persistent or increasing acidosis and lactataemia and bleeding due to coagulopathy and persisting low platelet count. An INR of >4 and first-day AST levels of >2000 IU/L reflect major cell damage, but even levels of >5000 IU/L may settle with good long-term graft function. From 48 h post-transplant, serum transaminase levels should halve each day. Serum bilirubin may rise in the first few days, but thereafter it should settle in the absence of infection or rejection.
The only treatment for PNF is urgent retransplantation, and 1-year survival in this group is <50%.
33.7.2 Postoperative bleeding
Postoperative bleeding occurs in 5%–10% of children post-transplant. Risk factors include graft dysfunction, renal impairment, high intraoperative blood loss and low platelet count. Maintaining a satisfactory platelet count (>100 × 109/L) will help prevent bleeding in the first 48 h. Bleeding from the cut surface of a segmental graft may be a sign of venous outflow obstruction. Occasionally, a side branch of the artery opens up a few hours after surgery and may cause early acute blood loss and requires a return to theatre for suturing. However, in more than 50% of cases, no specific bleeding point is identified and coagulopathy is assumed to be responsible.
33.7.3 Vascular
33.7.3.1 HEPATIC ARTERY THROMBOSIS
HAT is the most serious technical complication due to the dependence of the graft on an intact arterial inflow. The historically reported incidence has been approximately 4% in adults and 10% in children [13
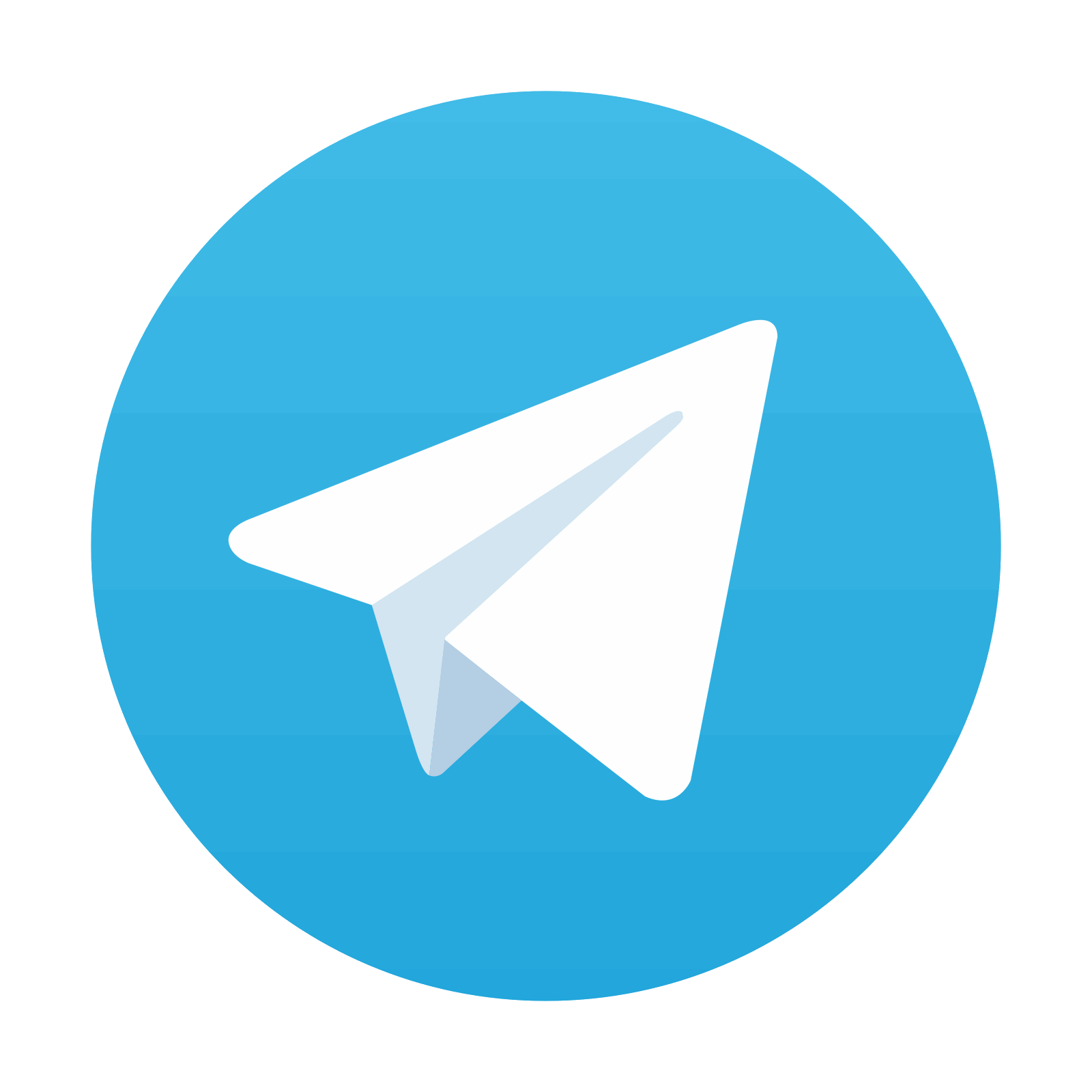
Stay updated, free articles. Join our Telegram channel

Full access? Get Clinical Tree
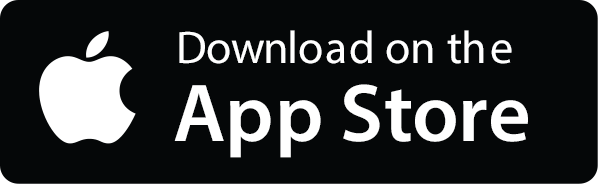
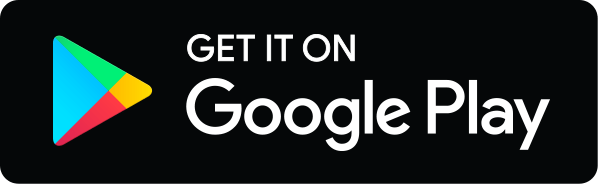