Figure 4.1 Arterial phase CT showing a peripherally arterialised tumour in the right lobe of the liver in an infant. There is evidence of centripetal enhancement and a hyperplastic feeding artery, which are characteristic features of a haemangioendothelioma.
4.2.5 Magnetic resonance imaging
MRI uses nonionising radiation (radiofrequency) within a strong magnetic field. The absorbed energy is released from the cell nuclei in a specific manner that is proportional to proton density, allowing differentiation of soft tissues. This results in a change in the magnetic field that is translated into a ‘signal’ of varying intensity which is plotted to produce a representation of a slice through the body. These signals can be presented in any plane, and may therefore be imaged in the axial, coronal or sagittal plane without mathematical reconstruction of the dataset. There are no known harmful biological effects, and repeat imaging is therefore acceptable. As with CT, fast techniques lead to a reduction in misregistration artefacts, resulting in enhanced resolution of the liver. The terminology of MRI is often complex, but a simple principle is to regard T1-weighted images as defining the anatomy and contrast-enhanced vascular characteristics of a lesion, and T2-weighted images as defining its inherent water content.
Haemangiomas, with their slow-flowing blood, and simple cysts therefore give a uniform high signal on the T2-weighted acquisition. On this sequence, solid tumours are isointense or of nonuniform, marginally higher signal than the surrounding liver.
Extended MRI techniques include vascular imaging of MR arteriography and MR venography (MRA and MRV) (Figure 4.2) and MR cholangiopancreatography (MRCP), which allow noninvasive imaging of the hepatic vasculature, biliary and pancreatic ducts. These techniques rely on the enhancement and ‘water’ properties of the structures. High-signal (‘bright bile’) techniques are utilised in MRCP and commonly use T2-weighted, breath-hold sequences with repeated or single acquisition. Image manipulation allows rotation of the summated image and display of the cholangiogram to best advantage (Figure 4.3). The diagnostic sensitivity of the techniques varies according to pathology and in particular whether the disease results in dilatation of the biliary system. For example, disorders such as choledochal malformations or choledocholithiasis that result in bile duct dilatation will be more reliably shown than the subtle duct changes of sclerosing cholangitis. The latter may only be present at the third- and fourth-order intrahepatic duct levels. Chemical shift techniques allow differentiation of focal fat from other causes of focal pathology.
Figure 4.2 Gadolinium-enhanced MR angiography and venography defining the arterial, portal venous and caval anatomy in a transplant recipient. A tight suprahepatic venous stenosis has been defined which was successfully treated by balloon dilatation.
Figure 4.3 MRCP demonstrating calculi within a dilated common duct.
Although tissue-specific agents using the paramagnetic properties of iron and manganese have been developed and utilised in MR practice, they no longer form an integral part of the investigation. This is now confined to gadolinium-based agents, some of which have hepatocyte specificity.
4.2.6 Arteriography
Although noninvasive mapping of the hepatic vascular anatomy (arterial, portal and hepatic venous) can be achieved with MRI, CT and US, there remains a requirement for invasive vascular techniques now generally as a prelude to intervention. The morbidity of angiography, which should not be overstated, has been significantly reduced by the use of nonionic low-osmolality contrast media, which carry a lower risk of hypersensitivity, tissue toxicity and renal failure. This has been coupled with improved registration and resolution using digital techniques. Arterial dissection and thrombosis are still potential complications, but are reduced by the use of high-flow, small-calibre catheters and heparinisation during the procedure. Any invasive procedure should be preceded by a clinical risk–benefit analysis, but with careful technique and an experienced operator, they carry a low morbidity.
In children with primary liver tumours, arteriography provides detailed information about their circulation, although it is seldom used nowadays. Characteristically, malignant tumours exhibit a neovascular circulation with new vessel formation, arterial or portal venous encasement and arteriovenous shunting (Figure 4.4). Arteriography also defines the level and volume of intrahepatic shunting of the primary vascular disorders of the liver, such as infantile epithelioid haemangioendothelioma and developmental arterioportal shunts (see Chapter 25). Furthermore, anatomical variants that may influence the surgical approach to liver transplantation or surgical resection are delineated. The most common of these include the replaced right hepatic artery as the first division of the superior mesenteric artery and the left hepatic artery arising from the left gastric artery.
Arterial catheterisation is also a prelude to embolisation as a therapy for liver tumours, a method of abating haemorrhage following blunt or penetrating hepatic trauma and a technique for the closure of developmental intrahepatic vascular shunts. Useful occlusive agents include tissue glues, thrombin, polyvinyl alcohol and metallic coils, the choice of agent being dependent on the size and level of shunt and whether it involves an end or anastomotic artery.
Figure 4.4 Hepatic arteriography showing the neovascular arterial characteristics of a hepatocellular carcinoma in the right lobe of the liver.
4.2.7 Portal venography
The portal venous system can be mapped by direct or indirect portography. The latter depends on imaging the venous return following splenic or superior mesenteric artery injection using digital subtraction techniques. Shunt therapy in cirrhosis or presinusoidal venous occlusion is directed by the extent of involvement of the portal venous system. The key issue is whether there is a segment of portal, superior mesenteric or splenic vein which will allow portosystemic shunting, either surgical or via transjugular intrahepatic portosystemic shunt (TIPS) placement that will completely decompress both the portomesenteric and splenoportal venous system.
Various patterns have been described, including the following:
1. Occlusion of the main portal vein and first-order intrahepatic divisions
2. Occlusion of the main portal vein and all intrahepatic divisions
3. Total occlusion of the portal venous system
Characteristically, venous collaterals termed cavernous transformation replace the occluded portal vein (Figure 4.5) (see Chapters 22 and 23).
The two main techniques of direct portography are splenic and transhepatic venography. Splenic venography is performed by injecting contrast through a 23-gauge Chiba* needle that is inserted into the splenic tissue via an intercostal route, and sequential digital image registration. The procedure demands careful technique and interpretation. A collateral circulation will be demonstrated in the presence of extrahepatic portal vein occlusion, but the superior mesenteric vein will not be shown unless there is retrograde flow. The portogram will reflect the pattern of variceal flow, and the portal vein may not opacify if there is severe portal hypertension with hepatofugal flow into retroperitoneal splenoportal shunts and short gastric varices.
Figure 4.5 Indirect portography showing cavernous transformation of the portal vein with bridging portal venous collaterals reconstituting intrahepatic portal venous radicals.
An alternative method depends on the percutaneous transhepatic cannulation of the intrahepatic portal venous system. This manoeuvre is also a prelude to portal vein dilatation or stenting in cases of segmental portal occlusion or stenosis that may occur as complications of liver transplantation.
4.2.8 Portal pressure measurements
In addition to defining the anatomy of the portal venous system, a key part of the investigation of portal hypertension is the measurement of portal pressure. Direct pressure measurements may be taken during splenic or transhepatic portal venography.
A pressure of
• 7–14 mmHg represents mild portal hypertension
• 15–30 mmHg represents moderate portal hypertension
• >30 mmHg represents severe portal hypertension
Alternatively, a quantification of the ‘bleeding risk’ can be deduced from the hepatic venous pressure gradient (HVPG), which represents the difference between the free and wedged hepatic venous pressure. This is measured using the technique of transvenous catheterisation of the hepatic veins, and if it is >12 mmHg, the patient is placed in a ‘high-risk bleeding’ category.
4.2.9 Cavography and hepatic venography
This technique is often coupled with pressure measurements as the method of diagnosing the cause and level in hepatic venous outflow obstruction which may complicate prothrombotic disorders, chemotherapy or liver transplantation. The diagnosis of Budd–Chiari syndrome is made by demonstrating the replacement of the main hepatic veins with a ‘spider’s web’ of hepatic venous collaterals (Figure 4.6). If a haemodynamically significant stenosis is demonstrated either at a hepatic vein orifice or within the suprahepatic inferior vena cava, then balloon dilatation or stent placement is often curative.
4.2.10 Direct cholangiography
Direct visualisation of the bile ducts is achieved by either percutaneous (PTC) or endoscopic retrograde (ERC) cholangiography. The choice of technique is dependent on the pathology and on local expertise. The techniques are complementary, not competitive.
PTC is indicated if there has been previous surgery with disconnection of the bile duct and the formation of a biliary enteric anastomosis with, for example, a retrocolic Roux loop. This type of biliary drainage will be present after the correction of many congenital biliary abnormalities, including biliary atresia and choledochal malformations, and in most techniques of paediatric liver transplantation.
Figure 4.6 Hepatic venography demonstrating the characteristic spider’s web of venous collaterals replacing the main hepatic veins in Budd–Chiari syndrome.
Figure 4.7 Percutaneous cholangiogram demonstrating an inspissated bile plug obstructing the distal bile duct of an infant who presented with obstructive jaundice.
PTC can define the pattern of bile flow and the site of any biliary fistula. With the use of saline irrigation, it also allows the clearance of the bile duct of inspissated bile. As in adults, it is important to exclude coagulopathy and to administer antibiotic prophylaxis in order to avoid the potential complication of septic shock. General anaesthesia is almost always required in children. Direct puncture of the intrahepatic bile ducts is usually achieved, but occasionally delayed bile duct filling occurs indirectly by diffusion of contrast from a sinusoidal injection. Opacification can also be achieved by direct puncture of the gallbladder, but there may be incomplete demonstration of the intrahepatic bile ducts if there is rapid flow through the papilla, or if the obstruction is proximal to the cystic duct. Technically, it is important to map the biliary system fully, recognising the normal anatomical variants and the developmental patterns at the pancreaticobiliary junction that are a key diagnostic feature of choledochal anomalies (see Chapter 7). Failure to opacify the bile ducts and the visualisation of hyperplastic lymphatics are supportive diagnostic features of biliary atresia.
If obstructive bile duct pathology is confirmed, then it may be possible to intubate the bile ducts to facilitate balloon dilatation, stent insertion or clearance of calculi (Figure 4.7). With the development of the neonatal endoscope, endoscopic retrograde cholangiopancreatography (ERCP) is now possible in all age groups and across the whole range of biliary disorders. It is of particular value in the investigation of persistent conjugated neonatal jaundice, when the diagnosis of biliary atresia can be excluded if the biliary tree is visualised. This obviates the need for laparotomy and operative cholangiography in difficult cases in which biopsy and noninvasive imaging have failed to establish a diagnosis. ERCP in this age group can also be used for diagnosis of conditions such as biliary hypoplasia, sclerosing cholangitis or Caroli malformation. As in adults, the technique can be extended to allow therapeutic intervention, including sphincterotomy, extraction of calculi, balloon dilatation of strictures and stent insertion. Complications include pancreatitis, haemorrhage and sepsis.
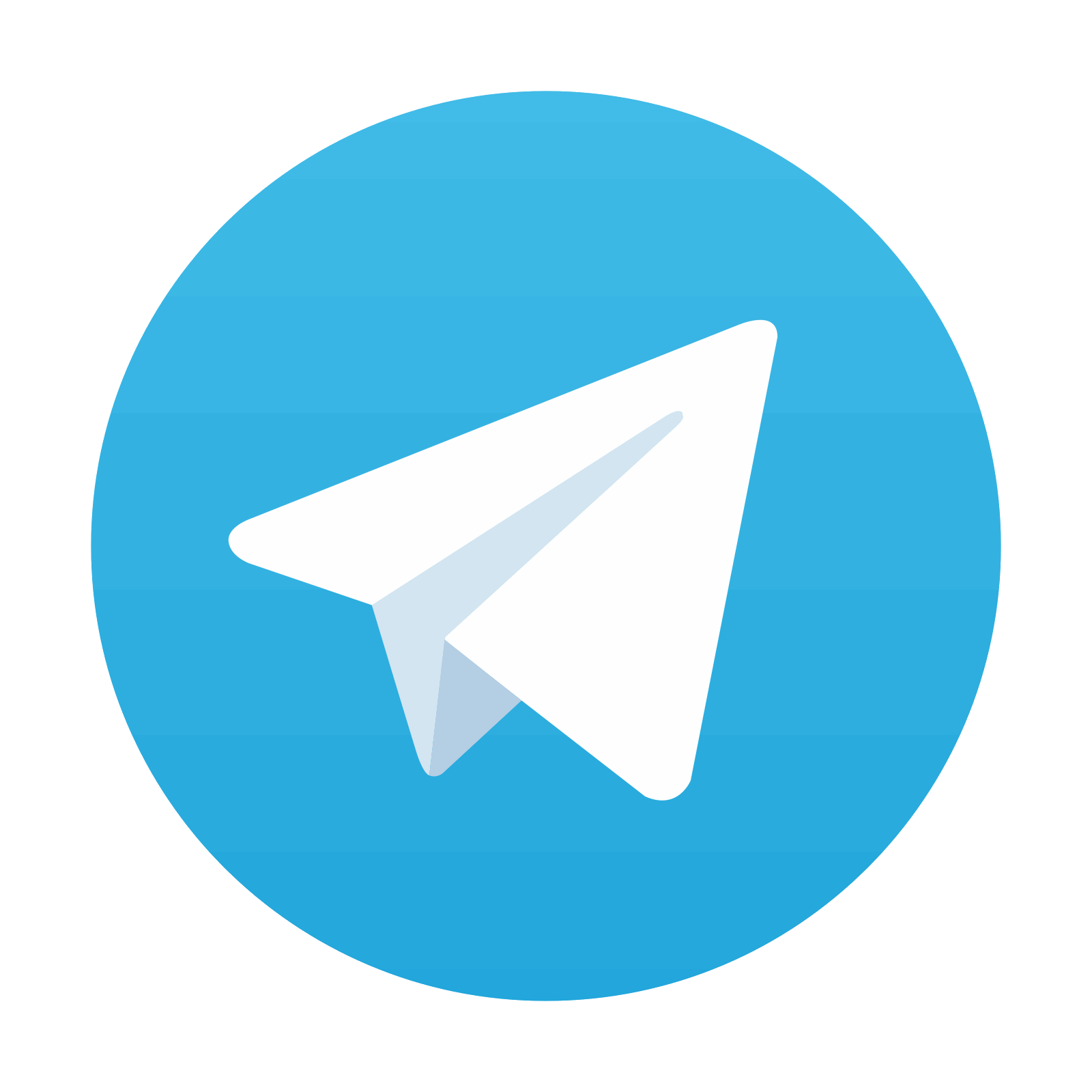
Stay updated, free articles. Join our Telegram channel

Full access? Get Clinical Tree
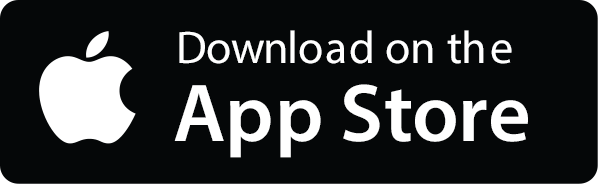
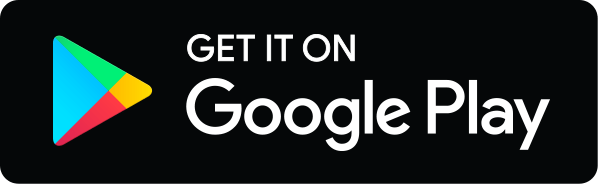