Junction stabilization
Small GTPase
Effectors
Molecular function
GEF
Rap1 a
Epac a (cAMP-dependent)
PDZ-GEF 1 a (cAMP-independent)
C3G b
Stabilization of VE-cadherin at junctions
Rac
Tiam (Rac specific)
Polimerization of cortical actin
Cdc42
R-RAS c
Inhibition of VE-cadherin internalization
GAP
Rho
P190Rho-GAP d
Inhibition of Rho
Junction destabilization
Rho
ROCK activation, phosphorylation of myosin light chain, actomyosin
GEF
Rac
Vav2
VE-cadherin endocytosis
How can the information developed through such molecular interactions at junctions be transmitted inside the cell to modulate its behavior? As anticipated above, this is primarily achieved through the recruitment at the cytoplasmic domain of VE-cadherin of molecules that possess either adaptor or enzymatic functions (β-catenin, cerebral cavernous malformation [CCM] proteins [see below], Rap1, and many others; see Lampugnani [46] for a more extensive list). VE-cadherin can also enroll transmembrane molecules at junctions, among which are both kinase receptors and phosphatase receptors [46]. In addition, post-transcriptional modifications of VE-cadherin can modulate such associations, as well as permanence at junctions of VE-cadherin itself (see below).
6.2.1 β-Catenin: The Canonical Cytoplasmic Partner of Vascular Endothelial (VE)-Cadherin
The classical cytoplasmic partner of VE-cadherin is β-catenin, a very versatile adaptor molecule that binds and recruits several regulatory and scaffold molecules to cell junctions (for a provisional list of molecules engaged to adherens junctions through β-catenin see Lampugnani [46]). Therefore, β-catenin provides a fundamental contribution to the molecular architecture and function of endothelial junctions. Indeed, truncated VE-cadherin, lacking the carboxy-terminal half of the cytoplasmic domain and unable to bind β-catenin, induces defects in vivo and in vitro superimposable to those observed after null mutation of VE-cadherin [11, 70].
It has been proposed that the phosphorylation of tyrosine residues of β-catenin can decrease its binding affinity for VE-cadherin. This has been reported in response to vascular endothelial growth factor (VEGF) [67]. In addition, focal adhesion kinase (FAK) could contribute to the weakening of endothelial junctions in response to VEGF, phosphorylating the tyrosine residue 142 of β-catenin and decreasing its association with VE-cadherin in vivo [13].
However, other reports are in contrast to the model in which VE-cadherin/β-catenin complex is modulated by tyrosine phosphorylation of β-catenin (or VE-cadherin itself; see below). Tyrosine phosphorylation of β-catenin could contribute to loss of barrier function, without requiring detachment from VE-cadherin. Indeed, Timmerman et al. [102] report tyrosine phosphorylation of VE-cadherin-associated β-catenin in response to thrombin, and no dissociation of the complex. Nonetheless, tyrosine phosphorylation of β-catenin is critical to sustain the permeability increase in response to thrombin. Indeed, depletion of the phosphatase SHP2, which dephosphorylates β-catenin, inhibits recovery of the barrier after thrombin challenge [102]. This may suggest that tyrosine phosphorylated β-catenin could change its association with partners other than VE-cadherin, and in this way could convey different signals. In summary, no general agreement on this issue has so far been reached. The specific mode of modulation of the VE-cadherin/β-catenin complex appears dependent on the stimulus and, possibly, on the origin of the endothelium. These variables could determine phosphorylation of specific tyrosine residues of β-catenin [56], resulting in apparently contrasting results (for example, see the opposite results obtained either with human umbilical vein endothelial cells [HUVEC] cultured on fibronectin, or human pulmonary microvascular endothelial cells cultured on collagen, reported by Timmerman et al. [102] and Monaghan-Benson and Burridge [67], respectively).
In some conditions of severely and constantly disorganized junctions, β-catenin can partially dissociate from VE-cadherin. For example, this has been reported in CCM1-silenced endothelial cells [31], and we have observed a similar event in CCM3-knockout endothelial cells [8]. A reasonable hypothesis is that, in endothelial cells, even a limited decrease in the association between VE-cadherin and β-catenin is sufficient to create subtle local heterogeneity in the molecular composition of cell-to-cell junctions that weaken the continuity of the monolayer and impair its barrier function.
In addition to its adaptor role at junction, β-catenin is a crucial regulator of transcription, particularly in association with transcription factors of the Tcf family [16]. This aspect is discussed below (see also comments in the legend for Fig. 6.1).


Fig. 6.1
Distribution of β-catenin to the nucleus inversely correlates to the stability of endothelial junctions. a In confluent endothelial layers in culture, β-catenin (red) is prevalently associated with cell-to-cell junctions where it co-distributes with VE-cadherin (green, co-distribution; yellow, arrows). b In migrating endothelial cells, which have dynamic adherens junctions in comparison to the stable monolayer, β-catenin can be observed in the nucleus (red, arrowheads), in addition to junctions, where it still co-localizes with VE-cadherin (green, co-distribution; yellow, arrow). Endothelial cells at the migrating front of a wounded monolayer are shown. c In VE-cadherin-null endothelial cells, β-catenin is also prevalently nuclear (red, arrowheads) in highly dense cultures. Endothelial cells in culture, and isolated from either wild-type or VE-cadherin-null mouse embryos, are shown. Active β-catenin (in the three panels), dephosphorylated on the N-terminal residues Ser37 or 41, is immune-stained with the mAb clone 8E7 (Merck Millipore, Darmstadt, Germany). Junctional β-catenin acts as a crucial scaffold for several signaling molecules (see Lampugnani [46] for a provisional list of such molecules). Nuclear β-catenin in the different situations shown is competent for regulation of transcription. This has been analyzed in detail for the transcription of claudin-5, a component of tight junctions with crucial activity in the control of permeability [100]. The mechanisms that direct β-catenin localization in the nucleus are not yet defined in molecular details. See the text for further discussion
A particular example of indirect control of transcription by β-catenin localized to junctions is the regulation of nuclear localization of the transcription factor FoxO1 (see below).
6.2.2 Other Cytoplasmic Associates of VE-Cadherin with a Crucial Role in Endothelial Physiology: Rap1 and the Cerebral Cavernous Malformations (CCMs)
Among the several molecules that associate with VE-cadherin at cell-to-cell junctions and control vascular permeability and vascular structure, we report here on the small GTPase Rap1 [32] and the CCM proteins (CCM1, 2, and 3) [85] as our laboratory also contributed to the definition of their function in the endothelium.
These molecules are discussed together as CCM1 has been demonstrated to recruit and localize Rap1 to cell-to-cell junctions [31]. CCM1 associates with adherens junctions, directly binding β-catenin through its FERM domain [31]. In addition, co-immunoprecipitation of CCM1 with the adherens junction components VE-cadherin , α-catenin, and afadin has been reported [31]. However, interaction with β-catenin appears to be nonexclusive for junctional localization of CCM1. Indeed, CCM1 can be recruited to cell junctions through interaction of its FERM domain (the groove between subdomain 1 and 3) with the C-terminal region of the transmembrane protein heart of glass (HEG1) [29]. In addition, CCM1 appears to sustain functions downstream of HEG1. Indeed HEG1, CCM1, or CCM2 murine and zebrafish mutants show convergent traits in their abnormal phenotypes [40].
The stabilizing activity of Rap1 on endothelial cell-to cell junctions has long been identified and analyzed in several models of endothelial cells cultured in vitro [32, 42]. The cyclic adenosine monophosphate (cAMP) analog 8-pCPT-2ʹ-Me-cAMP (007), a synthetic cell-permeable activator of Epac1 (an Rap1 GEF), is a potent stabilizer of VE-cadherin-based endothelial cell-to-cell junctions [32, 42]. In addition, Rap1b isoform knockout mice show hemorrhagic phenotype due to unstable blood vessels [14]. When ablated in mice, Rap1a, the other isoform of Rap1, does not determine a vascular phenotype. Similar effects of vessel fragility that result predominantly in cerebral hemorrhages have been observed in zebrafish larvae treated with Rap1b morpholino [34]. Local signaling activated by Rap1 would determine polymerization of cortical actin through the activation of Rac [6, 32]. Rap1 can recruit an Raf1/Rok-α complex to junctions for local modulation of actomyosin contraction [111], as discussed above). However, the molecular mechanism of junction stabilization in response to Rap1 activation still remains largely unknown. Association with junctions of GEFs and GTPase-activating proteins (GAPs) can regulate the localization and duration of Rap signaling [32]. PDZ-GEF1, an Rap1 GEF, can be found to be associated with VE-cadherin through β-catenin and MAGI1 [90, 32]. Another Rap1 GEF, Epac1, can associate with VE-cadherin through the phosphodiesterase PDE4D [83]. Afadin (AF6) [60], which can recruit both Rap-GTP and RapGAPs, can associate with adherens junctions [6, 32].
While constitutive activation of Rap1 is required for maintaining stable junctions, and its activation further reinforces them, it is debated whether Rap1 deactivation or delocalization can destabilize established cell-to-cell junctions [32].
Interestingly, besides CCM1, which binds directly to Rap1 [94], CCM2 and CCM3, which can form a complex with CCM1 [97, 107, 114], also strongly contribute to the maintenance of stable endothelial cell-to-cell junctions in both in vitro and in vivo models [7, 8, 51, 58]. Indeed, as soon as they are ablated, adherens junctions (and, as a consequence, tight junctions; see below) become disorganized and permeability is increased.
Local activation of Rap1 at adherens junctions, as promoted by CCM1, is critical for stabilization of endothelial junctions. Indeed, stimulation of Epac1 with 8-pCPT-2ʹ-Me-cAMP (007) in CCM1-ablated cells does not restore either endothelial barrier or localization of Rap1 to cell-to-cell junctions [31]. Consistently, activation of Epac1 does not restore junction and permeability in Rap1-depleted endothelial cells [77]. While it is established that CCM2 binds directly to CCM1, and CCM3 binds to CCM2 [97], it is not known how the absence of either CCM2 or CCM3 could influence the localization or activity of Rap1 bound to CCM1. In addition to affecting Rap1 GTPase, ablation of CCM1, 2, or 3 stimulate the activity of Rho, with consequent phosphorylation of MLC2, actomyosin contraction, and disorganization of cell-to-cell junctions [99, 109] (for Rho activation after CCM1 and 2 ablation); [8] (for Rho activation after CCM3 ablation). Inhibition of Rho activity can restore the structure and function of junctions in CCM-ablated endothelial cells, and inhibit formation of vascular lesions in vivo (see below). Rap1 could mediate the downregulation of Rho by CCM proteins recruiting Rac and inhibiting Rho activation at cell-to-cell junctions [6, 99]. Figure 6.2 summarizes the molecular interaction at cell-to-cell junctions between VE-cadherin, β-catenin, CCM proteins, and small GTPases.


Fig. 6.2
Schematic representation of the molecular interactions at endothelial cell-to-cell junctions between VE-cadherin, cerebral cavernous malformation (CCM) proteins, small GTPases, and the polarity complex. CCM1 interacts with both β-catenin and Rap1 [31]. As a consequence, Rap1 is activated locally (GTP-bound) and stabilizes cell-to-cell junctions through a mechanism that is still poorly understood [32]. Active Rap1, possibly activating Rac and inhibiting Rho, induces stabilization of the cortical actin cytoskeleton.In addition, active Rap1, stabilizing VE-cadherin to cell-to-cell junctions [42], indirectly promotes localization to cell-to-cell contacts of the polarity complex, Par3/Par6/atypicalPKC (aPKC) together with Tiam [48]. This complex can associate with VE-cadherin through recognition, by the third PDZ domain of Par3, of the type II PDZ-domain-binding motif at the C-terminus of VE-cadherin [38], and directs apical-basal polarity and the formation of a regular and polarized lumen [51]. CCM2 and CCM3 can form a complex [107] that might be recruited to cell-to-cell contacts in association with CCM1 [97], contributing to junction stability. Although localization to junctions of such ternary complex has to be definitely proved, the absence of any of the CCM proteins profoundly affects endothelial cell-to-cell junctions. Indeed, active Rap1 is delocalized from junctions (Glading et al. 2008; [34], junctions are dismantled, apical-basal polarity is lost [7, 51, 58] and lumen is abnormal in CCM-ablated (any CCM gene) endothelial cells cultured in vitro (Glading et al. 2008 [7, 51, 58], in murine models of CCM pathology [7, 58] and in vascular lesions of CCM patients [7, 58]
As illustrated by the individual examples discussed above, signaling conveyed by stabilized junctions depends on the association of specific molecules with junctions, and on their interactions. This relies primarily on clustering of VE-cadherin to cell-to-cell contacts. Therefore, the presence of VE-cadherin at junctions is crucial and can be regulated as discussed in the following paragraph.
6.2.3 Phosphorylation and Ubiquitination of VE-Cadherin and the Regulation of Vascular Permeability
The permanence of clustered VE-cadherin at cell-to-cell junctions can be modulated by post-transcriptional modification of the cytoplasmic domain of VE-cadherin, particularly phosphorylation on tyrosine (Y658 and Y685 [75]) and serine (S665 [26]) residues. Such post-transcriptional modifications can regulate the internalization rate of VE-cadherin and, as a consequence, the stability, composition, and signaling activity of adherens junctions.
VE-cadherin posses several tyrosine residues (eight in the human protein and nine in the mouse protein) that could be potential targets of tyrosine kinases [81]. Of these residues, only tyrosine residues at positions 658 and 685 have, to date, been reported to be phosphorylated in vivo in the mouse 45, 75]. In a current model, tyrosine phosphorylation of VE-cadherin in response to angiogenic or inflammatory stimuli has been associated with weak and permeable cell-to-cell junctions [81, 103]. However, tyrosine phosphorylation of VE-cadherin may not be sufficient, and other signaling pathways possibly need to concur to determine the loss-of-barrier function [1]. This concept has been further reinforced by the work of Orsenigo et al. [75], who observed that VE-cadherin is indeed phosphorylated on tyrosine residues 658 and 685 in vivo, specifically in the veins under physiological conditions. Such phosphorylated VE-cadherin is regularly distributed to cell-to-cell junctions, and the rate of its internalization is not increased in comparison to the nonphosphorylated form. However, such phosphorylation sensitizes the cells to respond to permeability-increasing agents such as bradykinin and histamine, with consequent enhanced ubiquitination and internalization of VE-cadherin. Endothelial cells expressing the nonphosphorylatable form of VE-cadherin, such as Y658F or Y685F, with tyrosine (Y) mutated in phenylalanine (F), do not reduce their barrier function in response to either bradykinin or histamine, nor internalize the mutated VE-cadherin. Specific phosphorylation of VE-cadherin in veins is determined by the low shear stress characterizing veins and mediating the constitutive activation of Src that directly or indirectly targets VE-cadherin [75].
The serine residue 665 (S665) appears to be a critical target in the action of VEGF as an agent that decreases the endothelial barrier [26]. Although the effects of such phospho-residue have been studied in detail in in vitro models of cultured endothelial cells , its presence has been observed as a short-term response to VEGF also in vivo in VE-cadherin from mouse skin vessels [27]. Once more, a key factor would be Src, which, activated by VEGF receptor 2 (VEGFR2), upon VEGF binding, would phosphorylate Vav2. This GEF starts a chain of activating events: activation of the small-GTPase Rac, which stimulates its downstream target p21-activated-kinase (PAK) to phosphorylate the residue S665 in VE-cadherin. Β2-arrestin recognizes such phosphorylated residue and, upon binding to VE-cadherin, promotes clathrin-dependent internalization of VE-cadherin. The consequence of this process is weakening of cell-to-cell junctions. Interestingly, this sequence of events can be blocked by angiopoietin-1 (Ang1). Ang1, promoting the sequestering of Src through mDia, inhibits the phosphorylation of VE-cadherin on S665, its internalization, and the ensuing weakening of junctions.
Phosphatases are expected to modulate the phosphorylation of VE-cadherin. Until now, the tyrosine phosphatase SHP2, which is associated with VE-cadherin through β-catenin, has been reported to dephosphorylate β-catenin, γ-catenin, and p120 associated with VE-cadherin , but not VE-cadherin itself [102, 105].
The endothelial-specific vascular endothelial phosphatase (VE-PTP) is associated with VE-cadherin at junctions through interaction of the extracellular domains of the two proteins. VE-PTP can indeed dephosphorylate VE-cadherin, besides Tie2 and plakoglobin [24, 89]. It has recently been reported that VEGFR2, when associated with junctions, can also be a target of VE-PTP [35].
In addition, clathrin-dependent internalization of VE-cadherin can be inhibited by p120 catenin masking an endocytic signal [69].
6.2.4 VE-Cadherin can be Associated with, and Modulate the Activity of, Angiogenic Receptors
VE-cadherin clustered at junctions can also modulate the activity of receptors that act as crucial regulators of vessel organization, such as VEGFR2 [11, 50] and transforming growth factor (TGF)-βR2 [88]. Activated by VEGF, VEGFR2 becomes phosphorylated on several tyrosine residues [15]. These phosphorylated tyrosines become docking sites for different mediators that can activate locally-specific signaling pathways [15], depending on the stability of junctions. In5 particular, we have observed that when VE-cadherin is engaged in stable contacts, as in confluent endothelial monolayers, activated VEGFR2 associates with VE-cadherin through β-catenin and activates phosphatidylinositol3-kinase (PI3K) [also associated with VE-cadherin through β-catenin] to phosphorylate Akt and to signal cell survival and resistance to apoptosis [11, 100]. When VE-cadherin is engaged in dynamic junctions, as in cells undergoing angiogenic responses, VEGF-activated VEGFR2 preferentially stimulates the phospholipase C (PLC) and mitogen-activated protein kinase (MAPK) pathway to signal proliferation [49]. Such signaling can take place from endocytic compartments in which VEGFR2 is internalized in association with VE-cadherin through a clathrin-dependent process [50].
VE-cadherin clustered in stable contacts can modulate endothelial behavior, forming a complex with another regulator of the vascular phenotype, the TGFβ receptor TGFβR2 (as well as with Alk1 and Alk5 [88]). The functional consequence of such local regulation is the activation, by TGFβ, of antiproliferative and antimigratory responses. Such signaling would contribute to the stability of the mature endothelial layer. On the contrary, in endothelial cells with weakened junctions, as is the case after treatment with a VE-cadherin-blocking antibody [88] or with a reduced level of VE-cadherin [58], TGFβR2 is not associated, or is less associated, with VE-cadherin [88]. This deactivates the antiproliferative and antimigratory response elicited by TGFβ, and can even redirect TGFβ signaling towards dedifferentiation (endothelial-mesenchymal transition [EndMT]) of the endothelial cells, as described in more detail in the following paragraphs in CCM pathology.
VE-cadherin has also recently been shown to be associated with fibroblast growth factor receptor 1 (FGFR1) and to reduce its phosphorylation in response to FGF2 engaging the phosphatase Dep1 in a multiprotein complex [28].
6.3 Adherens Junctions and Nuclear Signaling: Mediators and Targets in the Establishment of Barrier Properties and Differentiated Phenotype of the Endothelium
In addition to regulating endothelial functions through the local organization of specific signaling complexes to adherens junctions, as described in the previous paragraphs, the ‘degree of tightness’ of VE-cadherin association with junctions appears to regulate the transcriptional profile of endothelial cells [100]. ‘Degree of tightness’ means distinct and specific molecular complexes recruited by VE-cadherin to adherens junctions as a function of the duration and stability of its engagement in an adhesive interaction. In general, the molecular details of the dynamic remodeling of such complexes are still poorly known. Some examples have been presented in the previous paragraphs. Among the interactors of VE-cadherin, β-catenin plays an important role in coordinating the state of the junction compartment to the quality and type of transcription in the nuclear compartment. Although such regulation is evident from a functional point of view, details of the underlying molecular mechanism are still virtually unknown.
Examples of loss and acquisition of differentiated phenotype through the cross-talk between adherens junctions and the nucleus mediated by β-catenin in endothelial cells will be discussed in more detail below; however, before then, we have to face the still unsolved problem of cell biology.
6.3.1 Nuclear β-Catenin: How Adherens Junctions Could Control It?
In addition to its scaffold role in adherens junctions, β-catenin is a crucial regulator of transcription in association with Tcf transcription factors [16]. At present, the relationship between the junctional and nuclear pool of β-catenin remains an unsolved general issue in cell biology, besides endothelial cells. It is not known whether β-catenin concentrated in the nucleus originates from junctions or whether it comes from a distinct pool. In Drosophila, the existence of two distinct pools, junctional and nuclear, of the β-catenin homolog armadillo, is ascertained [91], and is confirmed in Caenorhabditis elegans [43]. In contrast, in mammalian epithelial cells, photoactivatable GFP-tagged β-catenin has been observed to re-localize from E-cadherin to the nucleus upon dissociation of adherens junctions [39].
While it is reasonable to hypothesize that some communication exists between these two pools, the molecular details of such a connection are not defined. A possible mechanism through which cell-to-cell junctions could regulate the level of the cytoplasmic pool of β-catenin, and indirectly the level of nuclear β-catenin, has been reported in epithelial cells [59]. In these cells, crucial components of the β-catenin phosphodestruction complex, such as axin, adenomatous polyposis coli 2 (APC2), and glycogen synthase kinase (GSK)-3β, are localized to cell-to-cell contacts, and cadherins can promote N-terminal phosphorylation of β-catenin, which targets β-catenin to proteasomal degradation. As a consequence, when junctions are tightly organized, N-terminal phosphorylation of β-catenin and its turnover are enhanced. The reduced pool of cytoplasmic β-catenin would limit the nuclear distribution of β-catenin and its transcriptional activity. Whether the phosphodestruction complex acts on β-catenin released from cadherin or on an independent pool has not presently been clarified. N-terminal phosphorylated β-catenin can also be found at cell-to-cell junctions “in a complex which is molecularly distinct from the cadherin-catenin complex” [59]. However, such coordination between tightness of junctions and targeted degradation of β-catenin would regulate the cytoplasmic and nuclear amount of β-catenin and allow the cell to mount a transcriptional response mediated by β-catenin and appropriate to the state of junctions.
6.3.2 Nuclear β-Catenin in Endothelial Cells
In addition to the above general questions, endothelial cells present a peculiarity in respect to the classical model of a direct balance (proportion/ratio) between the cytoplasmic and nuclear level of β-catenin. In our experience, endothelial cells can concentrate β-catenin in the nucleus without apparently requiring accumulation of (stabilized) β-catenin in the cytoplasm, as generally observed after stimulation with Wnt in other cell types. Constant finding in endothelial cells is that when adherens junctions are poorly organized, β-catenin concentrates in the nucleus and regulates transcription. Examples of weak cell-to-cell contacts are: endothelial cells in nonconfluent layers as during angiogenic response; models of pathological conditions as after KD of the CCM1 [31] and knockout of the CCM3 gene [8]; and the extreme experimental situation of VE-cadherin-null endothelial cells [100]. Remarkably, in each of these circumstances, although the total level of β-catenin can decrease, even to an extremely low level, as in VE-cadherin-null endothelial cells, residual β-catenin concentrates into the nucleus where it is transcriptionally active. The mechanism of such nuclear localization of the low total level of β-catenin has not presently been defined. Notably, β-catenin lacks nuclear localization sequences [16, 23].
When junctions are poorly organized, β-catenin induces a transcriptional profile characterized by loss of differentiation markers and acquisition of EndMT markers [8, 58, 100], as discussed below.
On the other hand, endothelial cells can also use β-catenin to direct a program of differentiation. Indeed, endothelial cells of the central nervous system (CNS), which participate in the blood–brain barrier (BBB) when stimulated by either Wnt3a [55], Wnt7a, or Wnt7b [98] activate a canonical response and block proteasomal degradation of β-catenin that now concentrates into the nucleus where it induces a BBB transcriptional profile [55, 78]. In parallel, Wnt induces stabilization of adherens junctions, with increased localization to junctions of VE-cadherin and β-catenin, and tightening of tight junctions through upregulation of claudin transcription [55, 98] (see below). As far as adherens junctions are concerned, both VE-cadherin and β-catenin appear more regularly disposed to cell-to-cell contact in Wnt3a-treated endothelial cells from brain microvessels; however, this is not the consequence of transcriptional activation of these genes. The molecular mechanisms of strengthening of adherens junctions in response to Wnt is not yet defined in endothelial cells, although it might involve regulation of small GTPases [93].
All in all, the transcriptional responses elicited by nuclear β-catenin appear to be coordinated to the state of endothelial cell-to-cell adherens junctions. The molecular mechanisms that direct such alternative responses of endothelial cells are still mostly unknown (see also below).
6.3.3 Adherens Junctions and Transcriptional Regulation of Permeability: The Case of Claudin-5 and Claudin-3
The extreme example of fragile endothelial junctions is that determined by the absence of VE-cadherin. Until now, this situation has mostly been studied in experimental models of in vitro cultured endothelial cells. Indeed, in the organism, constitutive inactivation of the VE-cadherin gene is embryonically lethal in the mouse [11] and in zebrafish [68], and VE-cadherin-inactivating antibody produces an acute lethal phenotype in the adult mouse [18, 19]. VE-cadherin can be dispensable for the early phase of sprouting angiogenesis when tip cells interact through filopodial contacts [52]. However, VE-cadherin is required for coordinating the following steps and allowing cells to properly recognize each other and mature the initial contacts, as observed in the zebrafish embryo [52].
In the absence of VE-cadherin, molecular determinants of the endothelial mature phenotype, such as claudin-5, VE-PTP, and von Willebrand factor, are transcriptionally downregulated [100] (unpublished results). In particular, claudin-5, an endothelial-selective constituent of tight junctions, is crucially involved in the control of endothelial permeability in the CNS [72]. The inhibition of claudin-5 transcription involves the formation of a nuclear complex between β-catenin and FoxO1, which acts as a transcriptional repressor of the Claudin-5 gene [100]. In addition, FoxO1 represents an interesting example of molecular cross-talk between junctional and nuclear compartments. Indeed, FoxO1 is phosphorylated through active Akt (phosphorylated on Thr308 and Ser473) localized to stable cell-to-cell contacts. Akt is activated locally at cell-to-cell junctions via PI3K, which associates with VE-cadherin through β-catenin only in stable contacts [11], as described above. Relevant for regulation of transcription is the fact that phosphorylation both promotes FoxO1 exclusion from the nucleus (phosphorylation on Thr24) and inhibits its binding to DNA (phosphorylation on Ser256) [9, 115]. As a consequence, transcription of claudin-5 (and other target genes such as VE-PTP and von Willebrand factor), is derepressed in endothelial cells with stable junctions, and the barrier properties are enhanced by the organization of claudin-based tight junctions.
An opposite example of transcriptional control of permeability by β-catenin is represented by the response of brain endothelial cells to Wnt3a and Wnt7a/b [55, 98]. As discussed above, in response to Wnt stimulus, β-catenin concentrates in the nucleus where it can activate a specific transcriptional program that drives differentiation of endothelial cells towards the CNS-specific phenotype (the BBB [21]). In particular, as far as control of permeability is concerned, the transcription of claudin-3 is upregulated both in vivo and in vitro [55, 98], and this has the functional effect of reinforcing the barrier properties of the endothelium [55, 98], as specifically needed at the BBB.
As discussed above, the molecular mechanism that determines very distinct β-catenin-driven transcriptional programs, in relation to different stability of adherens junctions, is still scarcely defined. As it has been shown for FoxO1, the cooperation between β-catenin and transcriptional co-regulators, the level or nuclear localization of which is regulated by the strength of adherens junctions, could be crucial. In addition, fine-tuning of the nuclear level of β-catenin could contribute to such distinct responses [33].
6.3.4 Adherens Junctions and Endothelial Differentiation: The Case of Endothelial-Mesenchymal Transition
EndMT represents a process of dedifferentiation driven by transcriptional reprogramming that also targets the composition and function of adherens junctions [64]. In the course of this process, the promigratory N-cadherin partially substitutes for VE-cadherin [28, 99]. The so-called cadherin switch, with N-cadherin substituting E-cadherin, is indeed a well-established trait of epithelial-mesenchymal transition (EMT) in epithelial cells [44]. Transcriptional repression of E-cadherin is well-defined in these cells, and similar mechanisms involving Twist, Snai1 and Snai2 also operate in endothelial cells [57]. However, reorganization of cell-to-cell junctions might also represent a priming event of EndMT. Although the precise relationship between junctional and nuclear β-catenin are poorly defined, as discussed above, β-catenin might represent one of the molecular link(s) in the reshaping of both junction and transcription profile that characterizes the EndMT. Indeed, β-catenin can drive the transcriptional upregulation of EndMT markers also cooperating with the TGFβ pathway [65, 116].
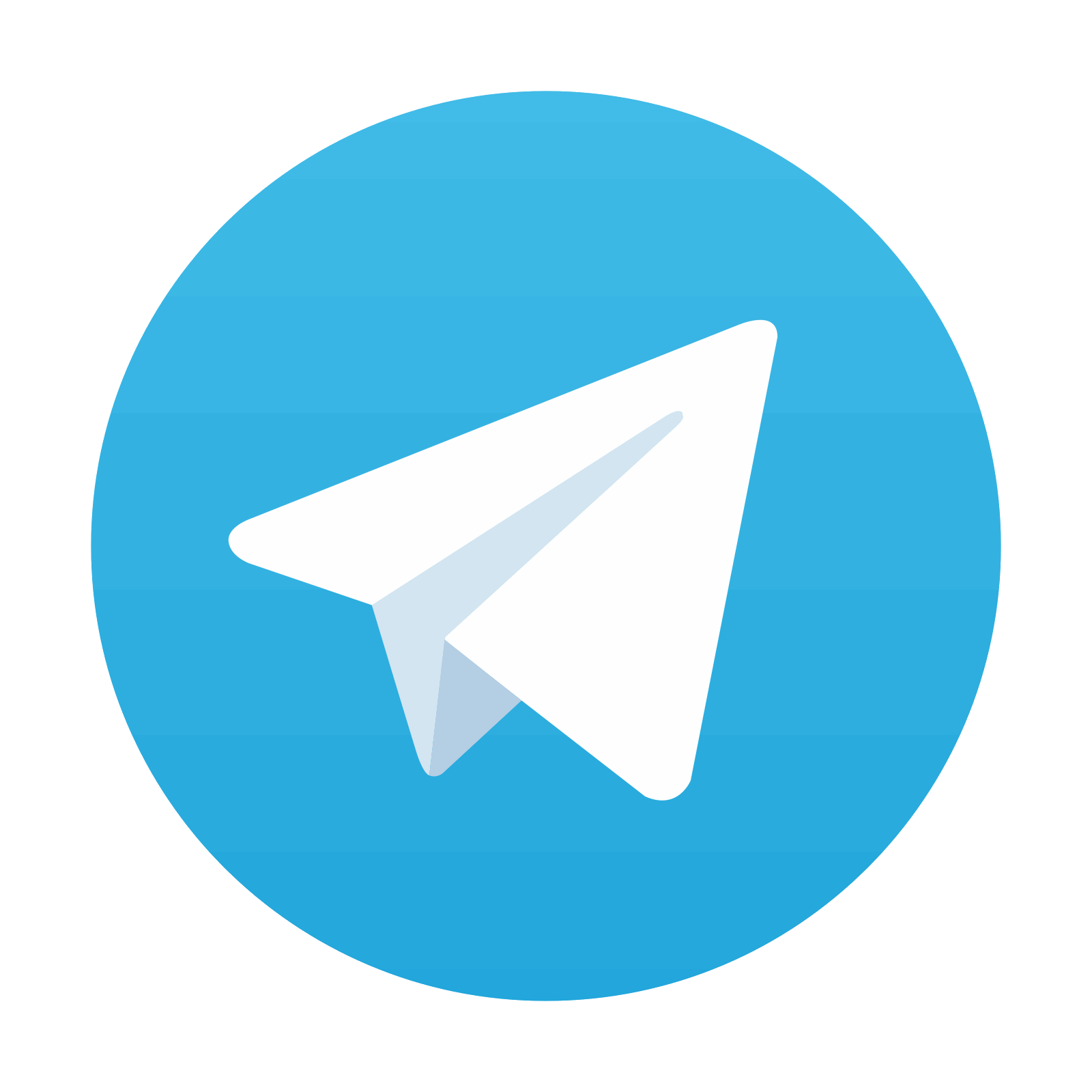
Stay updated, free articles. Join our Telegram channel

Full access? Get Clinical Tree
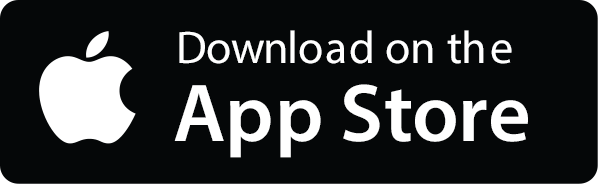
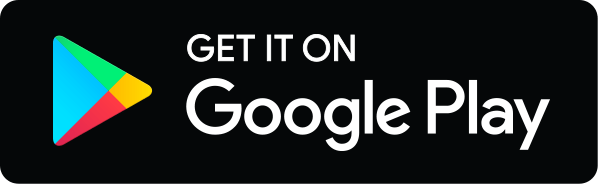