121 Inborn Errors of Metabolism
Individually, IEM are rare, but they collectively make up a significant source of disease, particularly in infants and children. Most diseases are autosomal recessive, but autosomal dominant, X-linked, and mitochondrial inheritance patterns exist (see Chapter 115).
Clinical Presentation
To accomplish this, a thorough history and physical examination will narrow the differential diagnosis before diagnostic testing. A complete dietary history may reveal symptoms instigated by certain food types or fasting. Food preferences or aversions may also be instructive. Other important information includes the frequency of and severity of response to illness, pattern of developmental delays, presence of consanguinity, and distinct body fluid odors. Although there are many physical examination and laboratory findings characteristic of specific disorders (Figure 121-1), several findings help to indicate general categories. For example, Kussmaul’s respirations may be found with metabolic acidosis or hyperventilation with hyperammonemia or cerebral edema. Organomegaly may also be evident as the result of a storage defect or organ dysfunction. Several disorders, such as the peroxisomal disorder Zellweger’s syndrome, have characteristic patterns of dysmorphia.
Evaluation And Management
Diagnostic Testing
When an IEM is suspected, it is often important to initiate treatment measures while an investigative workup is underway because a delay in therapy may affect the clinical outcome. Blood, urine, and cerebrospinal fluid (CSF) can be useful in the laboratory evaluation, and several laboratory studies are particularly helpful when an IEM is suspected in an ill child (Box 121-1). Serum electrolytes and blood gas analysis evaluate the acid-base status and anion gap. Ketone levels in urine, and sometimes blood, should be determined. Serum ammonia, lactate, and pyruvate, which can be tested in most hospitals, can also be informative, particularly in ill newborns. Metabolic laboratory studies such as plasma amino acids, plasma acylcarnitines, and urine organic acids, which provide important diagnostic information, must often be sent to specialized centers. Finally, one should consider other disease-specific testing, such as complete blood counts, to evaluate for bone marrow suppression in some organic acidurias. Molecular DNA testing and enzyme assays may require biopsy of specific tissues.
Common Presentations
Hypoglycemia
In general, hypoglycemia can be induced by lack of adequate glucose intake, poor homeostatic controls, iatrogenic causes, infection, and inborn errors of glucose metabolism. The brain is particularly dependent on glucose for energy but can alternatively use ketone bodies. Symptoms of hypoglycemia include lethargy, sweating, pallor, seizures, and mental status changes. Central to determining a metabolic cause of hypoglycemia is its onset after a carbohydrate load. For example, whereas early postprandial symptoms are more likely to be related to hyperinsulinism and glycogenoses, defects in fatty acid oxidation, ketone body synthesis, gluconeogenesis, and other IEM tend to take longer to present with hypoglycemia (Figure 121-2). Diagnostic pathways to approach the metabolic differential diagnosis for hypoglycemia are useful (Figure 121-3). A focused evaluation for an IEM may include electrolytes, glucose level, urine ketones, urine-reducing substances, ammonia, lactate, urine organic acids, and plasma acylcarnitines. One should also consider evaluation for non-IEM causes of hypoglycemia such as infection, drugs or toxins, and abnormal counterregulatory hormone mechanisms.
Hyperammonemia
Ammonia is a product of protein metabolism that, in elevated levels, is toxic to the central nervous system (CNS), resulting in cerebral edema. Normally, ammonia is converted to urea in the liver via the urea cycle and is excreted in the urine. When the nitrogen load exceeds the clearance capacity of the liver, ammonia accumulates. The nitrogen load increases with dietary protein intake and endogenous protein breakdown, both of which can cause hyperammonemia in patients with primary or secondary urea cycle defects. Symptoms of hyperammonemia include vomiting, seizures, lethargy, coma, and hyperventilation associated with respiratory alkalosis. Nonspecific episodes of headache, vomiting, and mental status changes may be the only signs in later-onset cases. For extremely high ammonia levels, such as during neonatal presentations of urea cycle disorders (UCDs), dialysis to rapidly reduce the toxic levels may be necessary in addition to less invasive therapies. Nonmetabolic causes of hyperammonemia include sepsis; liver failure; use of medications such as valproate; and transient hyperammonemia of the newborn, a disorder associated with severe neonatal hyperammonemia that resolves perinatally, although residual neurologic sequelae may result. Classic metabolic causes of hyperammonemia include UCDs, organic acidurias, FAODs, hyperornithinemia-hyperammonemia-homocitrullinemia syndrome, pyruvate carboxylase deficiency, and hyperammonemia-hyperinsulinemia syndrome. Several of these disorders, including organic acidurias and FAODs, cause secondary urea cycle inhibition. Whereas hyperammonemia without metabolic acidosis and hypoglycemia is suggestive of UCD, an anion gap metabolic acidosis should arouse suspicion for an organic aciduria. Diagnostic pathways for biochemical causes of hyperammonemia are depicted in Figure 121-4.
< div class='tao-gold-member'>
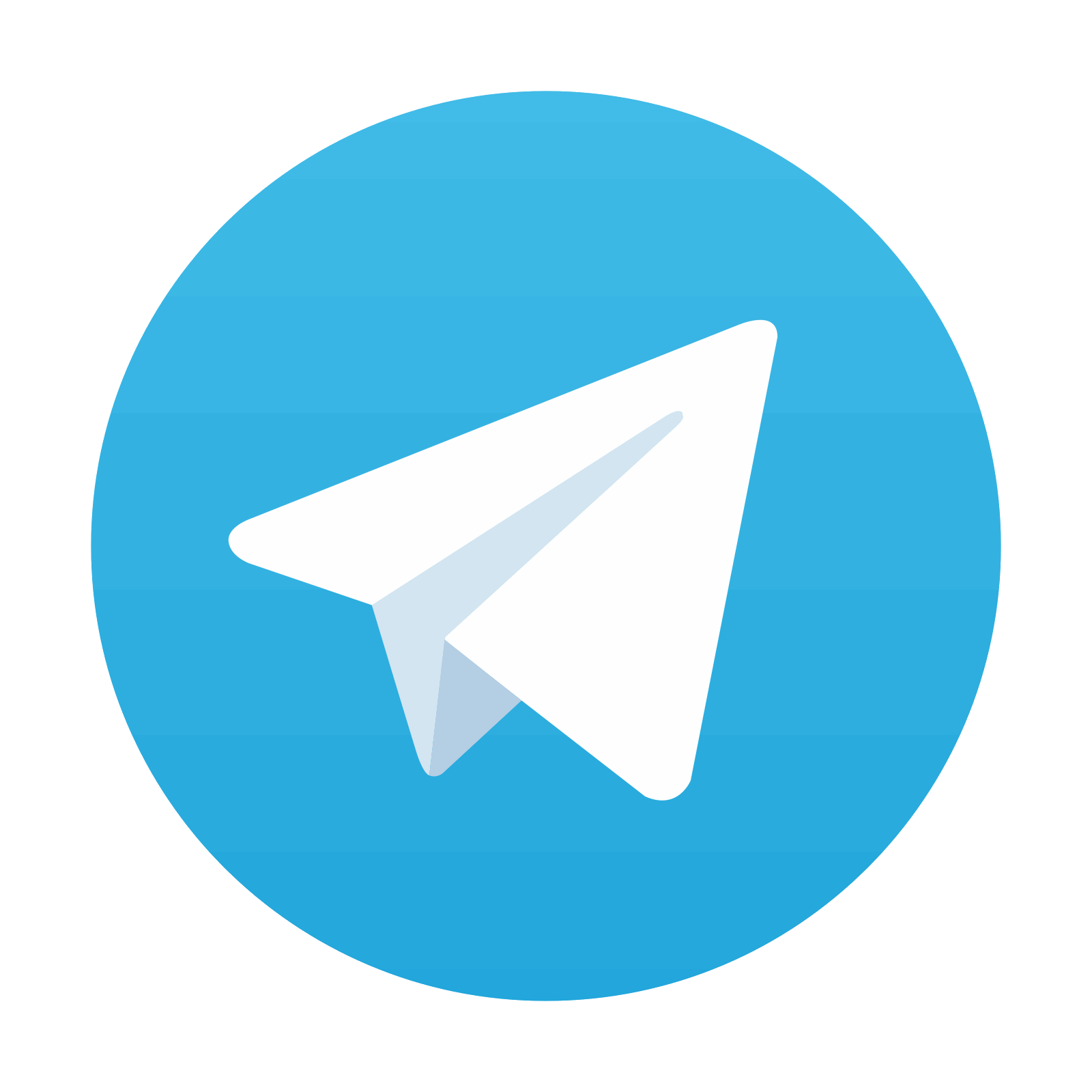
Stay updated, free articles. Join our Telegram channel

Full access? Get Clinical Tree
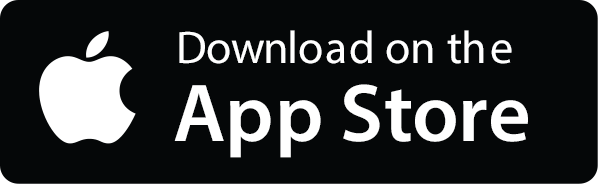
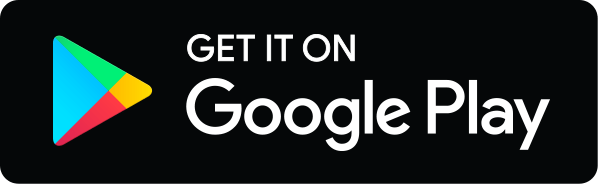