Fig. 1
Molecular mechanism of glucocorticoid action. GR glucocorticoid receptor, GC glucocorticoid, HSP heat-shock protein, FKBP FK506-binding protein, NF-kB nuclear factor-kB
Genetic and Epigenetic Predictors of GC Response
Given the high incidence of suboptimal response, associated with a significant number of side effects, the identification of subjects who are most likely to respond poorly to these agents seems extremely important. However, the mechanisms of steroid resistance and/or dependence are scarcely understood and there is presently no means to predict the response in advance.
Demographic and/or clinical markers [10, 66, 67] have been examined in correlation with GC response, but results have not been consistently replicated and could not be translated into clinical practice. Genetic markers are likely to complement clinical and demographic predictors. Phenotypes resulting from genetic changes, such as single nucleotide polymorphisms (SNPs), deletions, insertions, and duplications in genes involved in the complex GC mechanism of action can markedly influence drug pharmacokinetics or alter efficacy and/or toxicity profiles; in particular, genetic variants in the GR receptor heterocomplex, in the proinflammatory mediators in the downstream signaling pathway of the GC–GR complex, and in proteins involved in the extrusion (P-glycoprotein) and metabolism of GCs have been evaluated in the literature.
In addition, new genetic biomarkers have been studied: microRNAs (miRNAs), small noncoding RNA molecules that suppress the expression of genes involved in drug molecular mechanisms, have emerged as a promising field of pharmacogenomic research. Here we focus our attention on GR, which has a central role in GC molecular mechanisms, considering polymorphisms in its gene and the expression of miRNAs involved in its regulation.
GR Gene Polymorphisms
The human GR gene, NR3C1 (nuclear receptor subfamily 3, group C, member 1; Nuclear Receptor Nomenclature Committee, 1999), is located on chromosome 5q31.3 and includes nine exons [32]. Polymorphisms of this gene may impair the formation of the GC–GR complex and subsequently alter transactivation and/or transrepression processes that have been related to increased [68] or decreased [69] sensitivity to endogenous cortisol.
The TthIIII (rs10052957), ER22/23EK (rs6189/rs6190), GR-9β (rs6198), N363S (rs6195), and BclI (rs41423247) polymorphisms have been the most studied and have been associated with differences in metabolic parameters and body composition as well as with autoimmune and cardiovascular disease. These genetic variants have also been related to changes in GC sensitivity [70] and may therefore account for the variability in the response to GC therapy. Although very few studies deal with the mechanisms, it is usually assumed that NR3C1 polymorphisms lead to a modified GR transcript.
TthIIII (rs10052957) is a restriction fragment length polymorphism (RFLP) caused by a C > T change in the GR gene promoter region; it is located in a large intron of approximately 27 kb, 3,807 bp upstream of the GR start site [71]. This polymorphism has been associated with elevated diurnal cortisol levels and with a reduced cortisol response to 1 mg dexamethasone (DEX), as well as lower insulin and cholesterol levels [68]. Other studies suggest that this polymorphism has a biological role mainly in association with other GR polymorphisms, forming haplotypes [72], but to date it has not been correlated with clinical response to GCs in patients with IBD, neither alone nor in haplotype.
The ER22/23EK polymorphisms (rs6189 + rs6190) are located in the N-terminal transactivation domain of the GR and involve two nucleotide changes in codons 22 and 23 of exon 2 (GAG AGG to GAA AAG), which change the amino acid sequence from glutamic acid–arginine (E-R) to glutamic acid–lysine (E-K). Since the polymorphism is located in the transactivation domain, the amino acid change might affect the receptor’s tertiary structure, influencing the transactivational and/or transrepressional activity on target genes [73]. An association with higher post-DEX cortisol levels and less cortisol suppression after a 1 mg DEX suppression test in ER22/23EK carriers has been shown. In addition, the polymorphism is associated with a better metabolic and cardiovascular health profile and an increased survival [68, 69]. In a study considering the role of ER22/23EK in the variability of clinical response to GCs in IBD, no association was found between the ER22/23EK polymorphism and GC response in 119 pediatric patients [74]. These polymorphisms have been also studied in adult patients with IBD, but no correlation has been observed with GC-resistant phenotype, even when dividing patients into UC and CD groups [75].
GR-9β (rs6198) is an A to G nucleotide substitution located in the 3′-UTR of exon 9β, the terminal exon of the mRNA of the β isoform (nucleotide 3669 in X03348; rs 6198). The A to G nucleotide substitution is located in an ATTTA motif (changing it to GTTTA). This ATTTA motif is known to destabilize mRNA and decrease receptor protein expression in vitro [76, 77]. GR-β, generated through an alternative splicing [78], is unable to bind ligand, is transcriptionally inactive, and exerts a dominant negative effect on transactivation by interfering with the binding of GR-α to the DNA [79, 80]. Honda et al. [81] reported GR-β specific mRNA expression in lymphocytes of 83 % of patients with steroid-resistant UC compared with only 9 % in responsive subjects and 10 % in healthy controls and patients with chronic active CD. This observation was confirmed in colonic biopsies of patients with UC: Significantly more GR-β-positive cells were seen in the resistant group than in the GC-sensitive and control group [82]. However, in IBD, GR-β is expressed 100–1,000 times less than GR-α, and this challenges its role in the genesis of steroid resistance in this disease. The role of TthIIII, ER22/23EK, and GR-9β has been investigated in association with the response to exogenous GCs. The combinations of the three polymorphisms were studied in 646 patients with multiple sclerosis treated with GCs. In this study, the haplotype consisting of TthIIII, ER22/23EK, and 9β-G was associated with GC resistance and with a more rapid disease progression. However, this seemed to result from the presence of ER22/23EK and not from the other two polymorphisms [83].
Two single nucleotide polymorphisms in the NR3C1 gene, the N363S and BclI polymorphisms, have been, on the other hand, associated with an increased sensitivity to GCs. The N363S polymorphism, originally rs6195, currently listed in dbSNP as rs56149945, results in an asparagine (N) to serine (S) change in amino acid in codon 363. The N363S polymorphism may influence the interaction of the GR with coactivators and/or corepressors, one of the known functions of the N-terminal domain of this receptor [76]. Only few reports have studied the role of this polymorphism in the response to exogenous GCs. In 102 patients who underwent photorefractive keratectomy and received topical steroids as part of postoperative therapy, a significant correlation was found between N363S heterozygosity and ocular hypertension [84]. Furthermore, in 48 patients with Duchenne muscular dystrophy treated with prednisolone or deflazacort, the N363S carriers showed a trend toward a later age at loss of ambulation in comparison with noncarrier patients [85]. Only two studies to date have evaluated the role of this polymorphism in GC clinical response in IBD, but no relation was observed between the presence of this SNP and response to GCs both in pediatric and in adult patients [74, 75].
The BclI polymorphism (rs41423247) was initially described as a polymorphic restriction site inside intron 2, the nucleotide alteration was subsequently identified as a C > G substitution, 646 nucleotides downstream from exon 2 [86]. The molecular mechanism through which the BclI polymorphism exerts its effect is unknown. This polymorphism is associated with a clinical phenotype consistent with increased GC sensitivity in both heterozygous and homozygous carriers of the G allele. An association with unfavorable metabolic characteristics, such as increased body mass index and insulin resistance, has been also described [87]. The BclI SNP has been studied in 119 pediatric patients with IBD (64 with CD, 55 with UC). Patients were divided into two groups based on their response to GC treatment: GC dependence (45 patients) was defined by an initial response to prednisone with relapse on dose reduction, not allowing for steroid discontinuation, and GC responsiveness (67 patients) was defined as GC withdrawal without the need for steroids for at least 1 year. A significantly higher frequency of the BclI-mutated genotype was observed in the GC-responsive patients than in the GC-dependent group [74]. These results have been subsequently confirmed in a larger cohort of young patients with IBD [88] (Table 1).
Table 1
Polymorphisms in the glucocorticoid receptor (GR) gene associated with altered glucocorticoid (GC) response in patients with inflammatory bowel disease (IBD) and other diseases
GR gene polymorphisms | Correlations with GC response in IBD or other diseases | References |
---|---|---|
TthIIII (rs10052957) | Correlation with elevated diurnal cortisol levels and reduced cortisol response to 1 mg dexamethasone (DEX), as well as lower insulin and cholesterol levels | [68] |
No correlation in haplotype with clinical response to GC in IBD patients | [72] | |
ER22/23EK (rs6189/rs6190) | Association with higher post-DEX cortisol levels and less cortisol suppression after a 1 mg DEX suppression test | [69] |
Correlation with a better metabolic and cardiovascular health profile and an increased survival | [70] | |
In 119 pediatric patients with IBD, no association with GC response | [74] | |
In adult IBD patients, no correlation with GC-resistant phenotype even when dividing patients into UC and CD | [75] | |
GR-9β (rs6198) | Association of the haplotype consisting of TthIIII, ER22/23EK, and GR-9β-G with GC resistance, and with a more rapid disease progression | [83] |
N363S (rs6195) | Significant correlation with ocular hypertension in 102 patients who underwent photorefractive keratectomy and received topical steroids | [84] |
In 48 patients with Duchenne muscular dystrophy treated with prednisolone or deflazacort, correlation with a later age at loss of ambulation | [85] | |
No correlation with response to GCs in pediatric IBD patients | [74] | |
No correlation with response to GCs in adult IBD patients | [75] | |
BclI (rs41423247) | In 119 pediatric patients with IBD, association with GC response | [74] |
Unfavorable metabolic characteristics, such as increased body mass index and insulin resistance | [87] |
miRNAs Involved in GR Regulation and Their Potential Role
Recently, noncoding miRNAs have emerged as important gene expression regulatory elements; understanding of the complex gene regulation may shed light on the causes of the variable responses to these hormones in patients with GC-sensitive or GC-resistant inflammatory and autoimmune diseases [89].
miRNAs are small (18–24 nucleotides) noncoding RNAs, which bind the 3′UTRs and the coding exons of their target genes and inhibit gene expression [90] either by messenger RNA (mRNA) cleavage (most common in plants) or by translational repression (most common in metazoan) [91, 92]. A single miRNA can regulate approximately 200 mRNAs, and each mRNA can be regulated by multiple miRNAs [93, 94]; overall, it is predicted that protein production for at least 20 % of all human genes is regulated by miRNAs [95, 96]. miRNAs suppress gene expression at the posttranscriptional level, and are fine-tuning regulators of diverse biological processes, including the development and function of the immune system, apoptosis, metabolism, and inflammation. Emerging data have implicated the deregulated expression of certain miRNA networks in the pathogenesis of autoimmune and inflammatory diseases, such as IBD, and it has been suggested that these small noncoding RNAs represent an important player in the complex interactions that result in IBD clinical features [97–100]. A number of studies have identified a specific differential expression of miRNAs in IBD and unique miRNA expression profiles for the different subtypes of IBDs and for evolutionary stages of the disease in colonoscopic biopsies [97, 101]. In particular, Zahm and colleagues [102] identified a number of miRNAs significantly increased in the serum of patients with pediatric CD in comparison with healthy subjects. In addition, Wu and colleagues demonstrated for the first time that peripheral blood miRNAs can distinguish active IBD subtypes from each other and healthy controls. They identified 10 miRNAs significantly increased and 1 miRNA significantly decreased in the peripheral blood of patients with active UC as compared with patients with CD, 12 miRNAs significantly increased and 1 miRNA significantly decreased in the blood of patients with active UC compared with healthy controls, and 5 miRNAs significantly increased and 2 miRNAs significantly decreased in the blood of patients with active CD compared with healthy controls [103]. Paraskevi and colleagues recently identified 11 miRNAs significantly increased in CD and 6 miRNAs increased in UC blood samples compared with healthy controls [104]: These results confirmed previous studies of IBD miRNA expression obtained in blood and/or tissue samples [98, 105].
In this context, the study of a possible correlation between tissue or blood miRNA expression and variability in GC response in pediatric patients with IBD could be a promising field of research. The investigation of miRNA expression as a pathogenetic or pharmacological biomarker in plasma, serum, or peripheral mononuclear cells instead of colonic tissues represents a semi-invasive diagnostic approach, easier to translate into clinical practice, particularly in a pediatric population. Indeed, an ideal biomarker must be easily accessible using noninvasive procedures, and this is especially true when the patients are children.
An important field of investigation concerns the role of miRNAs in the regulation of target genes, such as NR3C1. Computational studies showed that the 3′ UTR of the GR gene is predicted to contain numerous seed regions recognized by a variety of miRNAs [106].
Vreugdenhil and collaborators investigated the possible interaction between miRNAs and NR3C1, and they found that miR-18 and miR-124a bind GR mRNA and decrease GR activity in neuronal tissues, using a combination of in silico prediction of miRNA binding sites, miRNA overexpression studies, and mutagenesis of the GR 3′ UTR [107]. The overexpression of these miRNAs reduced GR protein levels and impaired the activation of the GC-responsive gene glucocorticoid-induced leucine zipper (GILZ) in neuronal cell cultures. In addition, these authors demonstrated by miRNA reporter assay that miR-124a is able to bind to the predicted seed region in the GR 3′UTR.
The role of miR-124 has been investigated in the regulation of GR expression in human T cells of patients with critical illness-related corticosteroid insufficiency. It was found that miR-124 specifically downregulated GR-α, and a slight increase of miR-124 and a reduction of GR-α were observed in patient T cells compared with healthy controls [108]. In addition, Tessel and colleagues [109] identified and characterized miR-130b as an important downregulator of GR in a GC-resistant multiple myeloma cell line: The overexpression of this miRNA was also associated with a decreased regulation of GILZ, a downstream GC-controlled gene (Table 2).
Table 2
Role of miRNA deregulation in inflammatory bowel disease (IBD) considering glucocorticoid receptor (GR) as target
Sample analyzed | Correlations | References |
---|---|---|
Patient colonoscopic pinch biopsies | miRNA expression changes during tissue inflammation, and patterns of miRNAs are intestine region-specific | [101] |
Patient peripheral blood | miRNAs in peripheral blood can distinguish active IBD subtypes from each other and healthy controls | [103] |
Patient serum | miRNA profiles significantly increased in the serum of patients with pediatric CD in comparison with healthy subjects | [102] |
Patient blood samples | Pattern of 11 miRNAs significantly increased in CD and of 6 miRNAs in UC | [104] |
In vitro neuronal cell cultures | miR-18 and miR-124a bind GR mRNA and decrease GR activity | [107] |
Patient T cells | miR-124 in patients with critical illness-related corticosteroids insufficiency specifically downregulated GR-α | [108] |
GC-resistant multiple myeloma cell line | miR-130b is an important downregulator of GR | [109] |
Conclusions
GCs have been used in the treatment of active IBD since the 1950s, and are still used to induce remission in pediatric IBD, but interindividual differences in their efficacy and several side effects have been reported. The main goal for clinicians is therefore to improve the efficacy and safety of these agents and, when possible, to reduce steroid exposure and use a nonsteroid option. This is particularly important in patients who do not respond and will suffer considerable steroid-dependent morbidity without any clinical gain. The molecular mechanisms involved in the variability in GC response are still not completely known, but advances in pharmacogenomics could contribute to the optimization and personalization of therapy. Pharmacogenomic studies represent a promising field of research that could increase our understanding of the pharmacology of steroids in IBDs and possibly in other diseases.
In conclusion, the identification of pharmacological, genetic, and epigenetic determinants associated with GC response in pediatric patients with IBD and the consequent personalization of therapy based on this information will result in higher quality, less toxicity, and a more rational employment of national health service resources.
References
1.
Baldassano RN, Piccoli DA (1999) Inflammatory bowel disease in pediatric and adolescent patients. Gastroenterol Clin North Am 28(2):445–458PubMed
3.
Michail S, Ramsy M, Soliman E (2012) Advances in inflammatory bowel diseases in children. Minerva Pediatr 64(3):257–270PubMed
4.
Loftus EV Jr (2004) Clinical epidemiology of inflammatory bowel disease: incidence, prevalence, and environmental influences. Gastroenterology 126(6):1504–1517PubMed
5.
Longobardi T, Jacobs P, Bernstein CN (2004) Utilization of health care resources by individuals with inflammatory bowel disease in the United States: a profile of time since diagnosis. Am J Gastroenterol 99(4):650–655PubMed
6.
Carter MJ, Lobo AJ, Travis SP (2004) Guidelines for the management of inflammatory bowel disease in adults. Gut 53(Suppl 5):V1–V16PubMedCentralPubMed
7.
Ross SC, Strachan J, Russell RK, Wilson SL (2011) Psychosocial functioning and health-related quality of life in paediatric inflammatory bowel disease. J Pediatr Gastroenterol Nutr 53(5):480–488PubMed
8.
Dretzke J, Edlin R, Round J, Connock M, Hulme C, Czeczot J, Fry-Smith A, McCabe C, Meads C (2011) A systematic review and economic evaluation of the use of tumour necrosis factor-alpha (TNF-alpha) inhibitors, adalimumab and infliximab, for Crohn’s disease. Health Technol Assess (Winchester, England) 15(6):1–244
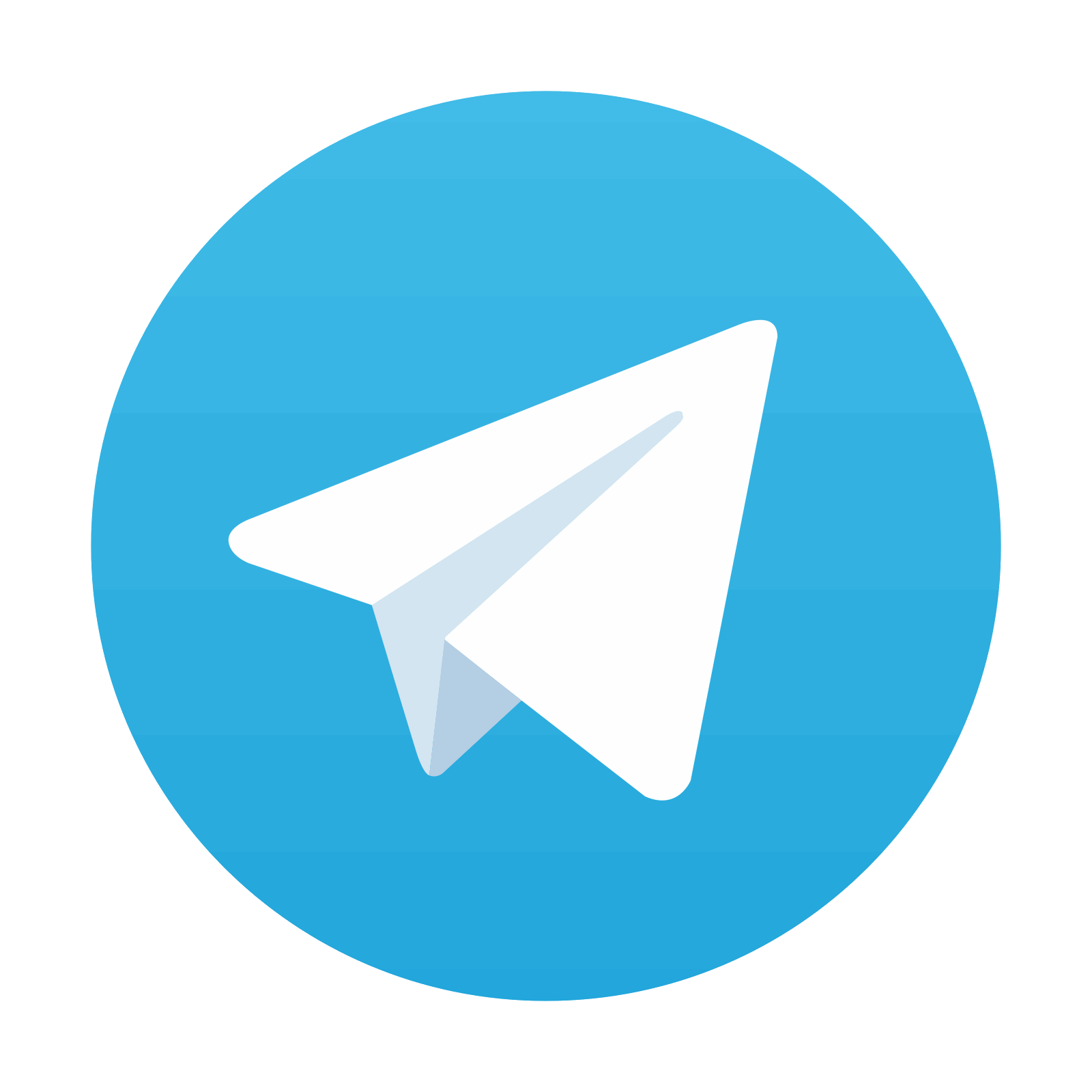
Stay updated, free articles. Join our Telegram channel

Full access? Get Clinical Tree
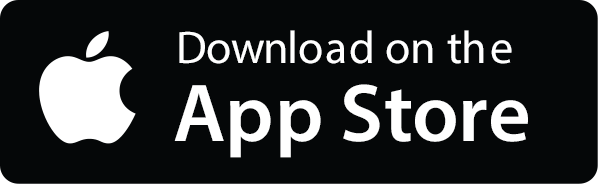
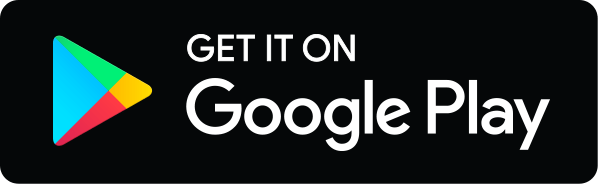