Fig. 2.1
Visual pathway. The visual pathway comprises the retina, optic nerve, optic chiasm, optic tract, lateral geniculate nucleus, optic radiation, and neurons in visual cortex
2.1.2 Prenatal Visual Development
In the prenatal (embryonic) period, the general structure of the visual system has taken shape. The generation of vision-related neurons and their functional localization, production of neuron axons and their projection onto the targeted neurons at the next level, and decussation of nerve fibers at the optic chiasma and functional ocular columns in the visual cortex have been formed. Prenatal visual development is mainly regulated by genetic information and molecular and endogenous electrophysiological stimulation. It is the developmental stage of the topographic projections that is independent of visual experience. Based on the regulating factors and the formation of neural circuits, prenatal visual development can be divided into two stages, the stimulus-independent and the endogenous stimulus-dependent. At the stimulus-independent stage, relatively disordered connections form among neuron synapses, which depend on genetic factors. At the endogenous stimulus-dependent stage, early visual neural circuits develop after stimulus from spontaneous neuron activities, which is primarily influenced by a series of regular action potentials spontaneously generated by the ganglion cells.
2.1.2.1 Stimulus-Independent Stage of Visual Development
Mediated by inherent genetic signals instead of electrophysiological activity and visual stimulus, it is the first stage of visual development, including cell division, differentiation, migration, and early adjustment, as well as early axon growth. At this stage, early normal structures and initial synaptic connections are formed [1]. Distribution and localization of rods, cones, and bipolar cells of the retina are determined by genetic information and eye development, neither of which is dependent on electrophysiological activity and visual stimulus.
The retinal ganglion cells, neurons in the lateral geniculate nuclei and in the primary visual cortex, are far apart during early embryo development. The axons of ganglion cells and neurons in the lateral geniculate nucleus grow to their target cells under the guidance of guiding molecules to form early synaptic connections. In animal experiments, it was found that the layered structures of lateral geniculate nucleus came into being prior to the formation of photoreceptor cells. Extending out to the lateral geniculate nucleus from the retinal ganglion cells, some early axons form imprecise synaptic connections with neurons in the lateral geniculate nucleus. Then the axons of neurons in the lateral geniculate nucleus reach the visual cortex.
2.1.2.2 Endogenous Stimulus-Dependent Stage of Visual Development
Early synaptic connections based on genetic factors are imprecise. They must be refined by spontaneous endogenous stimuli. Some researchers believe that endogenous stimuli originate mainly from retinal waves [2], the spontaneous cellular action potentials in the course of retinal development found in many vertebrates. The retinal waves are formed by retinal ganglion cells spontaneously generating a series of regular action potentials at random locations, which rapidly form synchronized stimulus waves spreading to both the inner and outer retina. With stimulation by retinal waves, the neurons in the lateral geniculate nucleus migrate to their six-layered structures, respectively, and form layered and topological projection of the axons of binocular ganglion cells in the lateral geniculate nucleus. Meanwhile, similar endogenous neural activity is found in the lateral geniculate nucleus and the visual cortex, and the topological projection of the lateral geniculate nucleus onto the visual cortex has developed [3]. Stimulated by these neural activities, the early topological projection of the axons takes shape, which prepares for greater refinement induced by postnatal visual stimuli. Under the joint effects of such mechanisms as direct stimulus, stimulus competition, and neurofeedback, the development of the ocular dominance columns and orientation columns in the visual cortex becomes more refined. Furthermore, some studies put forward an argument that, in addition to retinal waves, some eye-specific molecular signals are also involved [4].
Due to inadequate research on the visual development of human embryos, most of the knowledge about human visual development is derived from studies of mammals and primates. In fact, the process of human visual development bears a striking similarity to that of such animals, particularly primates. Their differences lie mainly in functional localization, duration of visual development, and advanced functionality. Hence, although these theories of visual development are applicable to humans, these theories cannot explain accurately the development of the human visual system.
Early morphological studies investigated the spatial-temporal distribution of the development of the human embryonic visual system [5]. Soon afterward, researchers learned about the morphological features and the dynamic developmental process of the retina, lateral geniculate body, and axon projections in the visual cortex by means of applying the axon tracing technique of DiI labeling to the human embryo [6]. In the human embryo, the nerve projection of the retina reaches the lateral geniculate nucleus at 7-week gestational age, whereas synaptic connections between the nerve fibers and the cells of the lateral geniculate nucleus form between 13 and 14 weeks of gestation. The axon tracing experiment conducted by Hevner demonstrated that the formation of the layered structures of lateral geniculate nucleus was preceded by separation of the retina, lateral geniculate nucleus, and nerve fiber terminal, and the latter is supposed to occur between 12 and 20 weeks of gestation, while the former remained hidden at 22-week gestational age. Early in fetal life, the lateral geniculate nucleus is merely a cell mass, and two big cellular layers are present at 22-week gestational age. Between 25 and 27 weeks of gestation, the six-layered structure becomes more obvious. Moreover, axons in the optic nerve reach its maximum density between 16 and 17 weeks of gestation and then gradually decrease, closing in on adult level at 29-week gestational age. The decrease of axons is probably associated with the refinement process of their projections.
2.1.3 Postnatal Visual Development
Formation of visual perception is dependent on sufficient visual stimulation, an accurate optical system, and construction of normal physiological and neural pathways. The optical and visual pathways in neonates are not well developed, and thus their visual perception is not refined. Visual development after birth involves the development of structure, physiology, and function of the visual system. Receiving visual stimuli and pattern stimuli from retinal ganglion cells, this stage is characterized with high plasticity and is equivalent to the exogenous stimulus-dependent stage after eye opening in infants. Visual environment and visual experience are of vital importance to visual development in this stage, especially during the critical period of visual development. Since the 1960s, Hubel and Wiese have made tremendous contributions to the research on visual development, with pioneering studies of the role of visual experience in visual development laid a foundation for subsequent studies [7, 8]. New techniques and equipment developed particularly in the last 20 years have provided the research on visual development in children with more conveniences.
2.1.3.1 Anatomical and Physiological Changes in Postnatal Visual System
At birth, the structure, physiology, and function of the optic nerve have begun to take shape, but in the visual system, the retina, the lateral geniculate nucleus, and the visual cortex, in particular, are not fully developed, and the connections among them remain to be refined. Subtle alterations will occur in both the organization and neural circuits of the postnatal visual system.
Retina Development
The fastest retina development in normal term infants is in the 6 months after birth, and the retina becomes fully developed in the following 1–4 years. At birth, the structure and function of the peripheral retina is almost fully developed, and the changes after birth occur mainly at the posterior pole, especially at the macula. The retinal ganglion cell layer, inner nuclear layer, and rod cells in the macula move to the periphery to form a pit (foveola), whereas cone cells keep migrating from the periphery to the central macula, resulting in a contracting foveola. The fovea is immature at birth and becomes similar to that of adults only at 15–45 months, which is not associated with visual stimuli. Both inner and outer segments of cones keep growing slimmer and longer after birth, with the inner segments comparable to the adult shape at 15 months but the outer segments less than half the length of those in adults. At 45 months, the inner segments reach the adult size, while the outer segments achieve 50–70 % of the adult length. It is a crucial factor in the rapid enhancement of visual sensitivity after birth that the density of cones in the macula increases rapidly by central migration and the cones growing longer and thinner. Both the density and length of cones in the foveola will increase from birth to adulthood.
The Development of the Lateral Geniculate Nucleus
Projection areas of retinal neurons in each layer of the lateral geniculate nucleus continue to develop after birth. Immediately after birth, the neurons in the lateral geniculate nucleus are identifiable but not fully developed. There are numerous dendrites and dendritic spines that reach the maximum in 4-month-old infants. They are comparable to that in adults around the second year, showing that they are fully grown [9]. The anatomical and histological observations in primates revealed that the differentiation of six layers of neurons in the lateral geniculate nucleus has been completed before birth. The ventral layers, layer I and layer II, consist of magnocellular neurons, while layers III, IV, V, and VI are composed of parvocellular neurons. After birth, parvocellular layers develop faster than magnocellular layers, reaching the adult level at the age of 1 year or so, while the development of magnocellular layers is not comparable with that of an adult until around 2 years of age. In infancy and early childhood, the spatial resolution of the lateral geniculate nucleus receiving the foveal fibers is extremely low, which can be comparable with that of an adult until 30 weeks.
The Development of the Visual Cortex
With the development of the visual function, the quantity, structure, and function of neurons in the visual cortex, as well as their synapses, may alter with circumstances, and they possess experience-dependent plasticity. There is a rapid increase of synaptic density in these neurons after birth, reaching the maximum peak at 8 months, twice as great as that at birth. With a slow decrease subsequently, the synaptic density in the visual cortex is comparable to that of an adult at 4 years. Advanced cortices develop to the adult level as late as the age of 11 [9, 10]. Increasing rapidly after birth, horizontal connections in the visual cortex form a homogeneous bundle at 7 weeks, becoming adult-type irregular projections after 8 weeks, and reach maturity by 15 months [11]. Nevertheless, a growing number of studies suggest that the function and structure in the visual cortex change through adolescence and adulthood [12–14].
Myelination
Myelination of the optic nerve progresses from the distal end to the proximal end of cells. It mainly occurs in the first 3 months after birth, and it ceases when it reaches the cribriform plate at the age of 2 years [15]. Yet the myelination of some of the visual areas of extrastriate cortex and interneurons among the cortical layers requires a longer period.
The Development of the Neural Circuit of Retina, Lateral Geniculate Nucleus, and Primary Visual Cortex Neurons
The development and maturity of the visual system involves not only the structural development of the fovea, the lateral geniculate nucleus, and the visual cortex but also the refinement of axonal connections among the retina, the lateral geniculate nucleus, and the visual cortex, as well as the changes into the primary and secondary visual areas in the visual cortex. The synaptic projection from the retina onto the lateral geniculate nucleus is complete before birth, and it is further modified after birth under the regulation of visual stimulation. Ocular dominance columns, which pass through the visual cortex, will continue to develop, and orientation columns, in particular, are almost completely developed at this stage. Visual experience is essential to the completion of modification of the visual pathways from the retina to the visual cortex, and the different activities between binocular neurons induced by it also influence the formation of ocular dominance columns. In other words, visual neural circuits go through a more delicate modification in the presence of neuronal activities induced by visual experience stimulation, giving rise to topological synaptic connections at all levels of the visual pathways and finally becoming the fully developed visual system [2].
2.1.3.2 Development of Visual Function
Vision
Vision refers to visual acuity. Children’s visual acuity changes dynamically. It is generally believed that it is almost fully developed at 5 years and relatively stable at 6 years and is similar to that of adults.
It is quite difficult to measure visual acuity in neonates and infants. Different examinations, such as subjective psychophysical examination and objective electrophysiological testing, show great discrepancy in results. Behavioral research observed that neonates develop slowly in grating acuity, reaching adult level as late as 3–5 years [16]. The study of visual evoked potential (VEP) discovered that, when amplitude is used as a grating frequency or contrast indicator, infants’ vision becomes comparable to that of adults between 6 months and 1 year [17]. As for preschool children, some researchers performed assessment on the visual acuities of children aged 3–6 years with the Landolt C chart and the tumbling E game, respectively. They found that, among young children aged 3–4 years, the visual acuity measured by E chart is far better than that measured by Landolt C. But for children aged 5–6 years, there is no difference in visual acuities between the two tests, and the visual acuities are indistinguishable from those of adults [18]. Therefore, children’s visual acuity test results are age specific and closely associated with testing methods as well. So particular care is required for children’s visual acuity assessment.
Binocular Vision
An external object forms a single image at the binocular corresponding retinal points and induces nerve impulses that are transmitted to the cerebral visual cortex, where two separate images can be fused into one single full image. This function is termed binocular vision or binocular single vision. Binocular visual function is divided into three levels from the basic to the advanced: simultaneous vision, sensory fusion, and stereopsis. In 1959, Hubel and Wiesel studied the interrelation of binocular vision, pointing out that the visual information converged at a very early stage [19]. In 1967, the driving cells sensitive to binocular parallax in the cat’s visual cortex were first found to be at Brodmann areas 17 and 18 [20]. Banks and colleagues stated that the sensitive period of the development of binocular vision starts several months after birth, with the peak between 1 and 3 years [21]. Leguire and colleagues investigated binocular summation of visual evoked potential among normal and strabismic children aged 1–58 months and revealed that there was a rapid increase in binocular summation response between 1.5 and 3 months, with a gradual decrease subsequently at 3–58 months [22]. They believed that it was associated with the emergence of conjugate ocular movements, sensory fusion, and stereopsis [22]. Fox and colleagues measured stereoacuity by using dynamic random-dot stereogram (RDS), demonstrating that stereoacuity emerged at 3.5–6 months. The result is consistent with the rapid development of the visual system after birth [23]. In terms of the mature stage of children’s development of binocular vision, there is a lack of systematic study. Romano and colleagues investigated the stereoscopic acuity of 1.5 to 13-year-old children with normal visual functions by means of the Titmus stereotest. They found that stereoacuity has been improving until the age of 9, and 40 s of arc in stereoacuity is the normal average level for children aged 9 and above [24]. Simons studied the stereoacuities of 3 to 5-year-old children and adults using the Frisby stereotest, Randot stereotest, random-dot stereogram, and TNO stereotest. He found that children’s binocular visual function has not yet been fully developed by the age of 5 [25]. Walraven and colleagues tested a population of 4–18 years using TNO stereotest and random-dot stereogram. They believed that stereopsis became gradually mature under the long-term stimulation of normal visual stimuli after children’s binocular vision had been established [26].
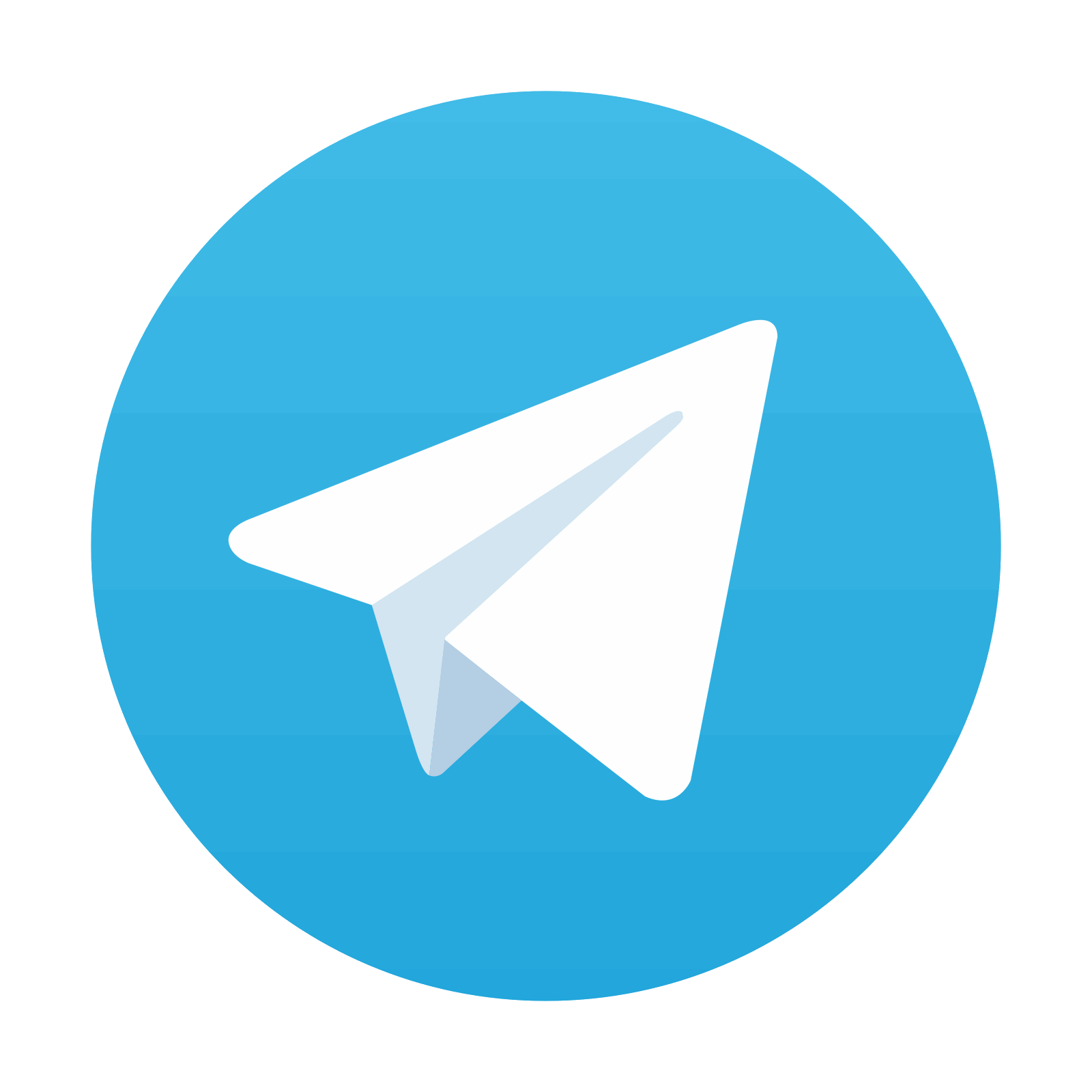
Stay updated, free articles. Join our Telegram channel

Full access? Get Clinical Tree
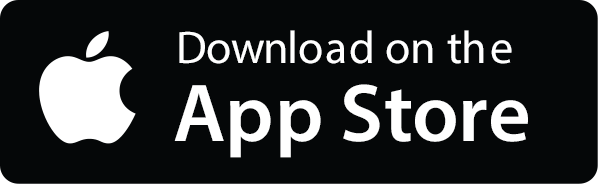
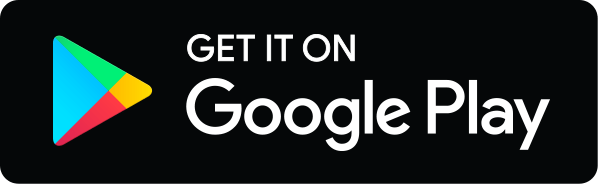