CHAPTER 10 Hormones
their action and measurement in gynaecological practice
Introduction
The term ‘hormone’, derived from the Greek word hormaein meaning to arouse or excite, was first used in 1905 by Sir Ernest Starling in his Croonian Lecture to the Royal College of Physicians. It is this ability to excite and modulate the behaviour of cells that is the fundamental defining characteristic of hormones.
Hormones are one of the important means by which cells communicate with each other. They ensure that the body’s physiological systems are coordinated appropriately, and if this is impaired, as occurs with hormone deficiency or excess, function is compromised. Classically, hormones are synthesized by specialized cells, which may exist as distinct endocrine glands or be located as single cells within other organs, and secreted into the blood stream and transported through the circulation to a distant site of action (endocrine effect). However, autocrine or paracrine actions may also be prevalent and indeed modulate the function of the original endocrine cell type (Figure 10.1).
Hormones: Types and Action
There are three major groups of hormones according to biochemical structure and method of synthesis:
Peptide hormones
The majority of hormones are peptides and range in size from very small (thyrotrophin-releasing hormone has just three amino acids) to small [thyroid-stimulating hormone (TSH) has over 200 amino acids]. Some peptide hormones are secreted directly, but most are stored in granules, the release of which becomes a major control mechanism regulated potentially by another hormone as part of a cascade or by innervation.
Some peptide hormones have complex tertiary structures; for example, glycoproteins are composed of more than one peptide chain. Oxytocin and arginine vasopressin, two of the posterior pituitary hormones, have ring structures linked by disulphide bridges. Despite being remarkably similar in structure, they have very different physiological roles. The gonadotrophins, follicle-stimulating hormone (FSH) and luteinizing hormone (LH), TSH and human chorionic gonadotrophin (hCG) also have two chains (Figure 10.2). However, these α- and β-subunits are synthesized quite separately from separate genes. The α-subunit of each is shared; it is the distinctive β-subunit of each that confers biological specificity.
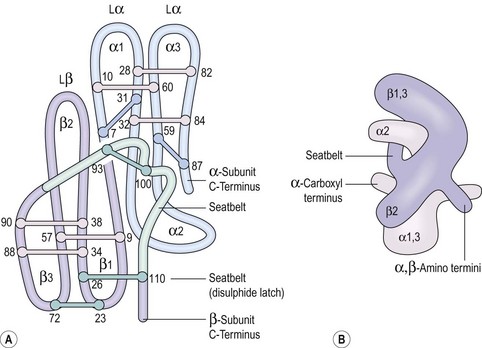
Figure 10.2 (A) Assembly of α and β subunits to form human chorionic gonadotrophin (hCG) dimer. Thick blue line, hCGα polypeptide chains; thick purple line, hCGβ polypeptide chains; numbers, amino acid numbers; Lα, loops of hCGα; Lβ, loops of hCGβ; pink bars, cystine knots on α and β subunits; thick green line of hCGβ, seatbelt region; thick green lines, seatbelt disulphide bonds; thin green line, small loop disulphide bond of β-seatbelt; blue lines, other disulphide bonds. (B) Spatial representation of α:β assembly in which the hCGα carboxyl terminal extension penetrates the hCGβ and is locked by the β-seatbelt portion.
(A) From Xing Y, Williams C, Campbell RK, Cook S, Knoppers M, Addona T, Altarocca V, Moyle WR. Threading of a glycosylated protein loop through a protein hole: implications for combination of human chorionic gonadotropin subunits. Protein Sci (2001) 10:226–235, Wiley. (B) From de Medeiros SF, Norman RJ. Human choriogonadotrophin protein core and sugar branches heterogeneity: basic and clinical insights. Hum Reprod Update. 2009 Jan-Feb;15(1):69–95. Epub 2008 Oct 22.
Amino acid derivatives
These hormones are small water-soluble compounds. Melatonin is derived from tryptophan, whereas the tyrosine derivatives include the thyroid hormones, the catecholamines, and dopamine from the hypothalamic–anterior pituitary unit and the central nervous system. The catecholamines of the adrenal medulla, ephedrine and norepinephrine, are identical to the sympathetic neurotransmitters and, like peptide hormones, they are stored in secretory granules prior to release.
Steroids
Steroid hormones are lipid-soluble molecules derived from modification of the four-carbon ring structure of cholesterol (Figure 10.3). Steroids can be classified according to the number of carbon atoms they possess: C-21 (progestogens, cortisol, aldosterone), C-19 (androgens) and C-18 (oestrogens). The common names used for steroids also adhere to a loose convention. The suffix -ol indicates an important hydroxyl group, as in cholesterol or cortisol, whereas the suffix -one indicates an important ketone (e.g. testosterone). The extra presence of -di, as in diol (e.g. oestradiol) or dione (e.g. androstenedione), reflects two of these groups, respectively; -ene (androstenedione) within the name indicates a significant double bond in the steroid nucleus.
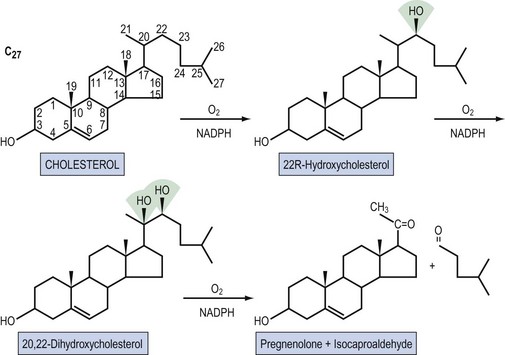
Figure 10.3 Enzymatic reaction catalysed by CYP11A; the first step in steroid hormone synthesis. CYP11A catalyses three sequential oxidation reactions followed by cleavage of the six carbon side chains to form pregnenolone. Each oxidation reaction requires one molecule of oxygen and one molecule of NADPH, and uses the mitochondrial electron transfer system.
Source: Reproduced from Payne and Hales; Overview of Steroidogenic Enzymes in the Pathway from Cholesterol to Active Steroid Hormones, (2004) Endocrine Reviews 25(6):947–970.
Regulation of Hormone Secretion and Action
Regulation and control of hormones is essential, and underpins not only the function of many endocrine systems but also their clinical investigation (Figure 10.4). Hormones utilize a variety of differential regulatory mechanisms.
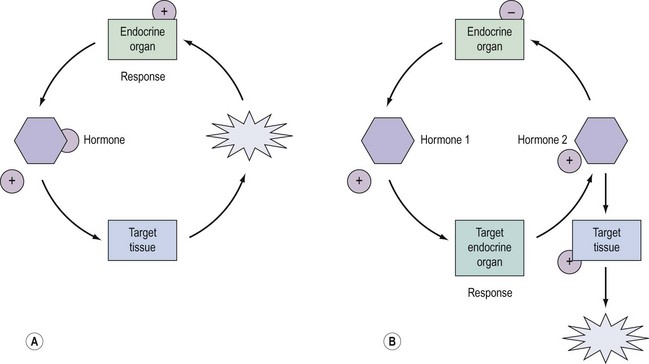
Figure 10.4 Two simplified models of hormone synthesis, action and feedback. (A) The endocrine organ releases a hormone which acts on the target tissue to stimulate (+) or inhibit (−) a response. The response may feedback either to inhibit or stimulate the endocrine organ to decrease or increase the supply of the hormone, respectively. (B) The endocrine organ produces hormone 1, which acts on a second endocrine gland to release hormone 2. In turn, hormone 2 acts dually on the target tissue to induce a response and feeds back negatively on to the original endocrine organ to regulate release of hormone 1. This model is illustrative of the axes between the anterior pituitary and its peripheral endocrine targets.
Simple control
An elementary control system is one in which the signal itself is limited, either in magnitude or duration, so as to induce only a transient response. Refinement allows discrimination of a positive signal from background ‘noise’ to ensure that the target cell cannot or does not respond below a certain threshold level. An example of this is the pulsatile release of gonadotrophin-releasing hormone (GnRH) from the hypothalamus. Similarly, although uterine epithelial cells have receptors for oestrogen, androgen, glucocorticoid and progestogen, there is no progestational response because progesterone is not produced in the first half of the menstrual cycle.
Negative feedback
Negative feedback is the most common type of regulation used by biological systems. The hormone acts on the target cell to stimulate a response that inhibits the first hormone. An extension of this scenario exists for many of the hypothalamic–pituitary–end organ axes; for example, corticotrophin-releasing hormone from the hypothalamus stimulates the release of anterior pituitary adrenocorticotrophic hormone (ACTH) to increase peripheral cortisol production, which feeds back to the anterior pituitary and hypothalamus to reduce the original secretions.
Positive feedback
Under certain circumstances, hormones feedback to the pituitary to enhance, rather than inhibit, secretion of the primary hormone. For example, the action of oestrogen on the pituitary gland to induce the ovulatory surge of LH and FSH; or during childbirth, stretch receptors in the myometrium send neurological signals to the brain to stimulate oxytocin release. The role of oxytocin in the suckling–milk ejection reflex is similar; a positive feedback loop that is only broken by cessation of the baby’s feeding.
Inhibitory control
The secretion of some hormones is under inhibitory as well as stimulatory control. Dopamine prevents the secretion of prolactin, such that if dopamine secretion is diminished, prolactin secretion will increase. This complexity from dual inhibitory and secretory control allows more precise regulation of hormone secretion.
Endocrine rhythms
Many of the body’s activities show periodic or cyclical changes and may either be circadian (24-h cycle, e.g. cortisol), ultradian (<24-h cycle, e.g. GnRH) or infradian (>24-h cycle, e.g. menstrual cycle). Control of these rhythms commonly comes from the nervous system, and although some are independent of the environment, others are coordinated and entrained by external cues, such as the 24-h light/dark cycle that becomes temporarily disrupted in jetlag. Cortisol secretion is maximal between 0400 h and 0800 h as we awaken, and minimal as we go to bed. In contrast, growth hormone (GH) and prolactin are secreted maximally 1 h after going to sleep. Clinically, knowledge of endocrine rhythms is important as analyses of hormones must take daily variation into account.
Availability of the hormone to a cell
A hormone can be present in the blood stream but unavailable to the target cell. A hormone circulating in the blood stream, not attached to a binding protein, readily enters cells by simple diffusion. However, most of the steroid hormones circulate bound to protein carriers, such as sex-hormone-binding globulin (SHBG), because their cholesterol origin makes them hydrophobic (King 1988). Consequently, they are generally unavailable, and alterations in the amount of circulating binding globulins can modulate the biological activities of each of their respective hormones. For example, approximately 40% of circulating oestrogen is bound to SHBG, and the remainder is bound to albumin and membrane proteins (Hovarth 1992). As testosterone is largely (80%) bound to SHBG and 19% is loosely bound to albumin, its androgenicity is mainly dependent upon the unbound fraction and partly upon the fraction associated with albumin. SHBG production in the liver is decreased by androgens, hence the binding capacity in men is lower than in normal women. In a hirsute woman, the SHBG level is depressed by the excess androgen, and the percentage of free and therefore active testosterone is elevated (Nestler et al 1991).
Tissue distribution
The tissue distribution of hormone receptors also determines the scope of hormone action, and the presence or absence of a given receptor determines whether a cell will respond to a given class of steroids. For example, the TSH receptor is limited to the thyroid and therefore TSH action is restricted to the thyroid. A hormone can also modify its own and/or another steroid hormone’s activity by regulating the concentration of receptors in a cell. This has the biological effect of increasing tissue response to the hormone if the receptor number is increased, and vice versa if the receptor number is decreased. Oestrogen, for example, increases target tissue responsiveness to itself by increasing the concentration of FSH receptors in granulosa cells (Hillier 1994). This process is important in the selection and maintenance of the dominant ovarian follicle in the menstrual cycle. In order to respond to the ovulatory surge and become a ‘successful’ corpus luteum, the granulosa cells must acquire LH receptors. FSH induces LH receptor development on the granulosa cells of large antral follicles with oestrogen acting as chief coordinator. Progesterone, on the other hand, limits the tissue response to oestrogen by reducing, over time, the concentration of oestrogen receptors, hence its use in the prevention of endometrial hyperplasia and carcinoma (Studd and Wadsworth 2000).
Hormone Receptors
The clinical effects of hormones are mediated through the interaction of a hormone with its receptors. There are two principal superfamilies of hormone receptors. This broad classification is based on their cellular location and their characteristic features (Figure 10.5).
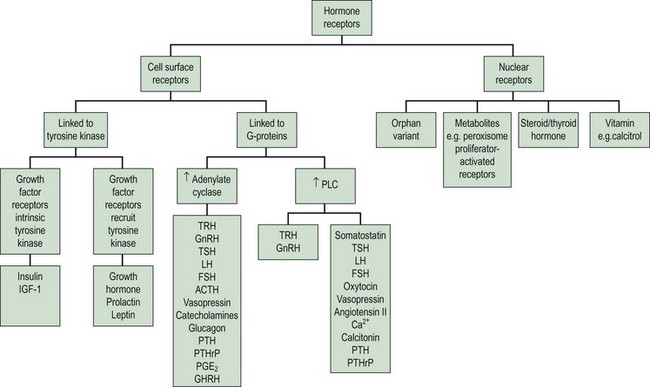
Figure 10.5 The different classes of hormone receptor. Some receptors can link to different G-proteins, which couple to either adenylate cyclase or phospholipase C (PLC). IGF-1, insulin-like growth factor-1; TRH, thyrotropin-releasing hormone; GnRH, gonadotrophin-releasing hormone; TSH, thyroid-stimulating hormone; LH, luteinizing hormone; FSH, follicle-stimulating hormone; ACTH, adrenocorticotrophic hormone; PTH, parathyroid hormone; PTHrP, parathyroid-hormone-related peptide; PGE2, prostaglandin E2; GHRH, growth-hormone-releasing hormone.
Adapted from Holt RIG, Hanley NA 2007. Essential Endocrinology and Diabetes, 5th edn. Blackwell Publishing, Massachusetts.
Cell surface receptors
Cell surface receptors are composed of three components, each with structural features, which reflect its location and function (Figure 10.6). The hormone acts as a ligand, with a ligand-binding pocket in the extracellular domain of the receptor being comparatively rich in cysteine residues that form internal disulphide bonds and repeated loops to ensure correct folding. This extracellular domain may also be cleaved for some hormones, and function as a binding protein in the circulation, thus limiting bioavailability (e.g. GH). The α-helical membrane-spanning domain is rich in the hydrophobic and uncharged amino acids, enabling it to be secured with the lipid bilayer. The C-terminal cytoplasmic domain either contains, or links to, separate catalytic systems which initiate the intracellular signals after hormone binding.
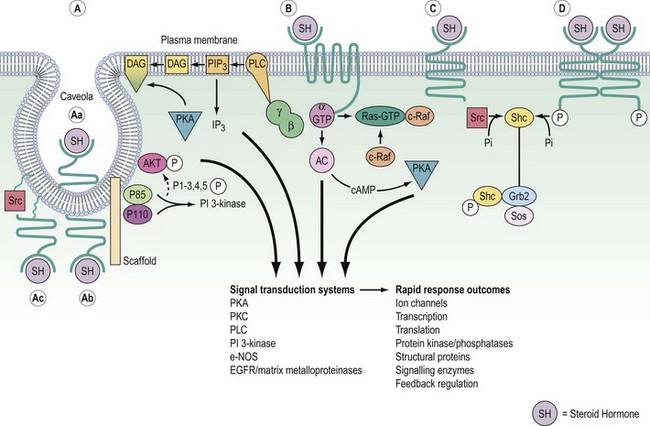
Figure 10.6 (A) Three classes of membrane receptor are shown, illustrating the classic nuclear steroid hormone receptor associated with a caveola. (Aa) The receptor is technically outside the cell and is associated with the outer surface of the plasma membrane in the flask of the caveola. (Ab) The receptor is tethered by a scaffolding protein to the plasma membrane on the inner surface of a caveola. (Ac) The receptor is tethered to the caveolae by a palmitic acid molecule that is esterified to a receptor Ser or Thr with the fatty acid side chain ‘inserted’ into the membrane (palmitoylation). (B) A G-protein coupled receptor with its ligand-binding domain on the outside of the cell and a seven-membrane-spanning peptide transition followed by an intracellular peptide domain that can bind G and proteins. (C) A single-membrane-spanning receptor with intrinsic kinase activity that might be functional as a monomer. (D) As for C except a homodimer. Caveolae are flask-shaped membrane invaginations present in the outer cell membrane of many cells; they are believed to serve as a ‘platform’ to accumulate or ‘dock’ signal-transduction-related molecules. The signal-transduction systems are listed as candidates for mediating rapid responses to steroid. The two ovals with Ras-GTP and c-Raf ‘touching’ are to suggest that c-Raf was recruited to the complex. AC, adenylyl cyclase; DAG, diacylglycerol; EGFR, epidermal growth factor receptor; e-NOS, endothelial nitric oxide synthase; IP3, inositol triphosphate; MAP, mitogen-activated protein; PI 3-kinase, phosphatidylinositol 3 kinase; PIP3, phosphatidylinositol triphosphate; PKA, protein kinase A; PKC, protein kinase C; PLC, phospholipase C.
Reproduced with permission from Norman et al.; Steroid-hormone rapid actions, membrane receptors and a conformational ensemble model, Nature Reviews Drug Discovery 3, 27–41 (January 2004).
Stage 1. Availability of the receptor (up- and downregulation)
The cell’s mechanism for sensing the low concentration of circulating trophic hormone is to have an extremely large number of receptors but to require only a very small percentage (as little as 1%) to be occupied by the hormone for its action to be evident (Sairam and Bhargavi 1985). Positive and negative modulation of receptor numbers by hormones is known as up- and downregulation. The mechanism of upregulation is unclear, but prolactin and GnRH, for example, can increase the concentration of their own receptors in the cell membrane (Katt et al 1985).
In downregulation, an excess concentration of a trophic hormone, such as LH or GnRH, results in a loss of receptors on the cell membranes and therefore a decrease in biological response. This process occurs by internalization of the receptors, and is the main biological mechanism by which the activity of polypeptide hormones is limited (Ron-El et al 2000). Thus the formation of the hormone–receptor complex on the cell surface initiates the cellular response, and the internalization of the complex (with eventual degradation of the hormone) terminates the response. It therefore appears that the principal reason for the pulsatile secretion of trophic hormones is to avoid downregulation and to maintain adequate receptor numbers. The pulse frequency, therefore, is a key factor in regulating receptor number.
It is believed that receptors are randomly inserted into the cell membrane after intracellular synthesis. They have two important sites — an external binding site, which is specific for a polypeptide hormone, and an internal site, which plays a role in the process of internalization (Kaplan 1981). When a hormone binds to the receptor and a high concentration of the hormone is present in the circulation, the hormone–receptor complex moves laterally in the cell membrane by a process called ‘lateral migration’ to a specialized area, the coated pit, the internal margin of which has a brush border (Goldstein et al 1979). Lateral migration, which takes minutes rather than seconds, thus concentrates hormone–receptor complexes in the coated pit, a process referred to as ‘clustering’ (Figure 10.7). When fully occupied, the coated pit invaginates, ‘pinches off’ and enters the cell as a vesicle. The coated vesicle is delivered to the lysosomes where it undergoes degradation, releasing the hormone receptors. The receptors may be recycled to the cell membranes and used again, or the receptor and hormone may be metabolized, thus decreasing the hormone’s biological activity. This process is called ‘receptor-mediated endocytosis’ (Goldstein et al 1979; Figure 10.8).
As well as downregulation of polypeptide hormone receptors, the process of internalization can be utilized for other cellular metabolic events, including the transfer of vital substances into the cell, such as iron and vitamins. Hence, cell membrane receptors can be separated into two classes. Class I receptors are distributed in the cell membrane and transmit information to modify cell behaviour for these receptors. Internalization is a method for downregulation and recycling is not usually a feature. Hormones which utilize this category of receptor include FSH, LH, hCG, GnRH, TSH and insulin (Kaplan 1981). Class II receptors are located in the coated pits. Binding leads to internalization, which provides the cell with the required ligands or removes noxious agents from the biological fluid bathing the cell. These receptors are spared from degradation and can be recycled. Examples of this category include low-density lipoproteins, which supply cholesterol to steroid-producing cells (Parinaud et al 1987), and transfer of immunoglobulins across the placenta to provide fetal immunity.
Stage 2. Binding of the hormone to the receptor
Notably hormone–receptor systems demonstrate standard kinetics and are saturable and reversible (Figure 10.9). Consequently, the dissociation rate of the hormone and its receptor is an important component of the biological response, as the biological activity of the hormone is only maintained while the hormone–receptor complex is maintained. Hormones that are structurally similar may have some overlap in biological activity. For example, the similarity in structure between GH and prolactin means that GH has a lactogenic action whilst prolactin has some growth-promoting activity and stimulates somatomedin production.
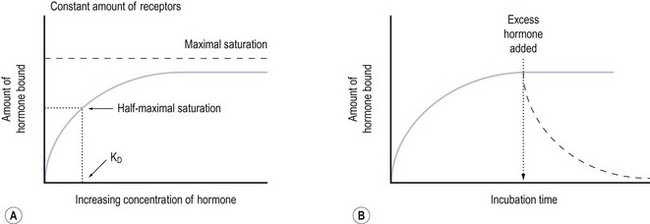
Figure 10.9 Pharmacokinetics of hormone receptors demonstrating that hormone–receptor systems are saturable and reversible. (A) Increasing amounts of hormone are incubated with a constant amount of receptor. The amount of bound hormone increases as more is added until the system becomes saturated. At this point, further addition of hormone fails to increase the amount bound to receptors. The concentration of hormone that is required for half-maximal saturation of the receptors is equal to the dissociation constant (KD) of the hormone–receptor interaction. (B) Constant amounts of hormone and receptors are incubated together for different times. The amount of hormone bound increases with time until it reaches a plateau, when the bound and free hormone have reached a dynamic equilibrium. By adding further excess hormone, there is competition for the binding sites and the amount of the first hormone bound decreases with time (dashed line).
Adapted from Holt RIG, Hanley NA 2007. Essential Endocrinology and Diabetes, 5th edn. Blackwell Publishing, Massachusetts.
The glycoprotein hormones (LH, FSH, TSH and hCG) share an identical α-chain and require another portion, the β-chain, to confer the specificity inherent in the relationship between hormones and their receptors. The β-subunits differ in both amino acid and carbohydrate content, and the chemical composition may be altered under certain conditions, thereby affecting the affinity of the hormone and its receptor (Willey 1999).
Stage 3. Signal transduction
On binding of a hormone to its cell surface receptor, protein phosphorylation and the generation of ‘second messengers’ mediates a cascade of cytoplasmic and cellular responses. This multistep cascade facilitates amplification of the cellular response to the hormone binding to a receptor, as many second messenger molecules are produced for each hormone–receptor complex. The cell surface receptors may be classified into two groups according to the second messenger and signalling cascades that are stimulated: (1) tyrosine kinase receptors and (2) G-protein coupled receptors (Figure 10.5).
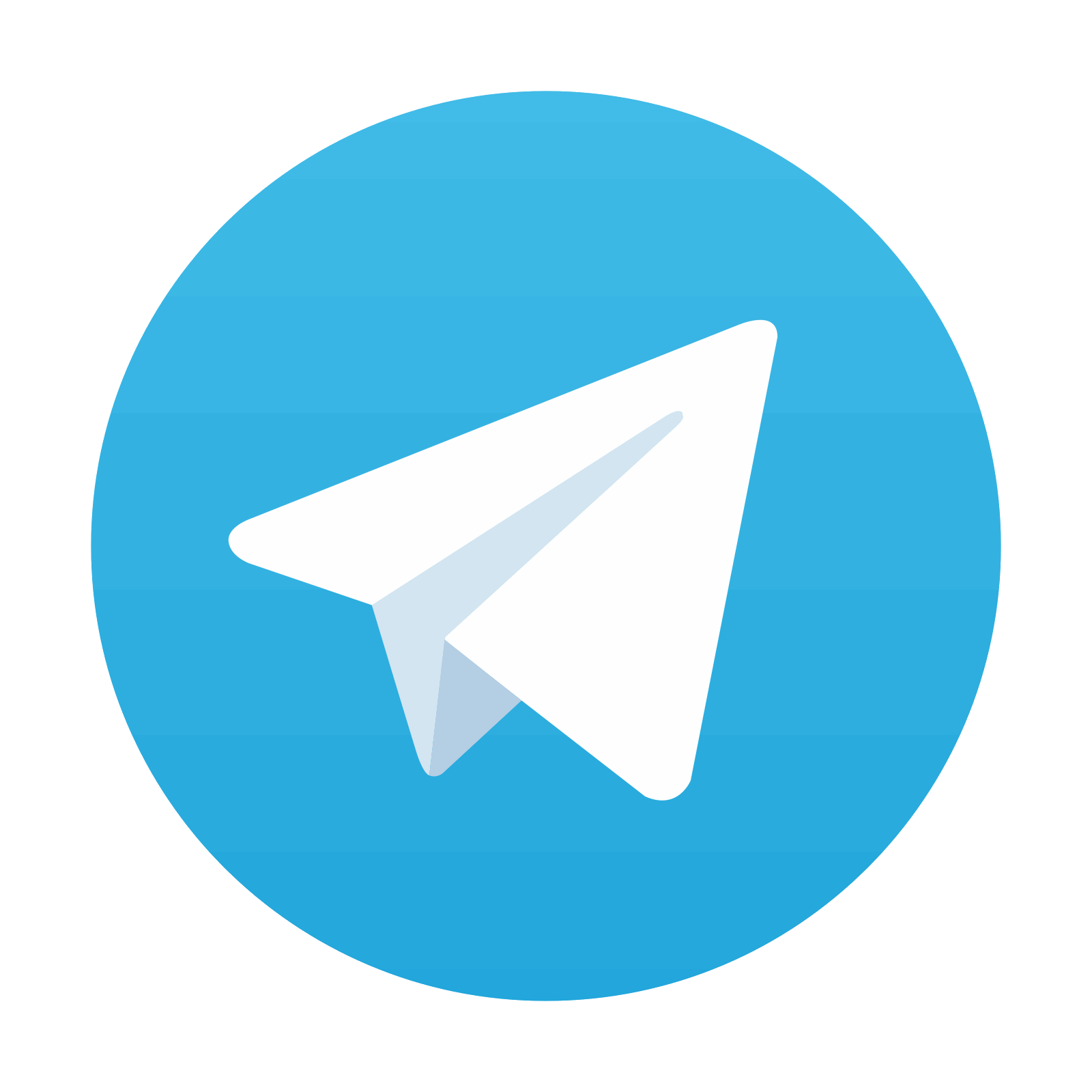
Stay updated, free articles. Join our Telegram channel

Full access? Get Clinical Tree
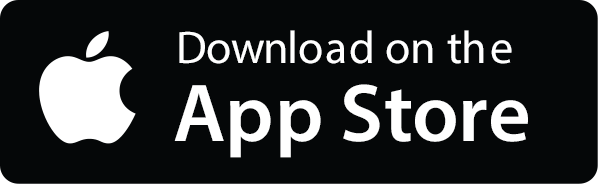
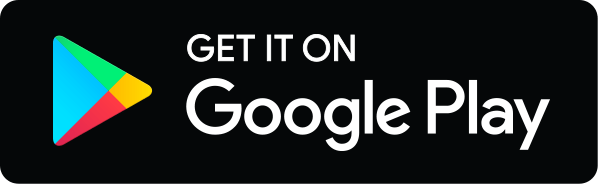