Chapter 24: Hemodynamically based management of circulatory compromise in the newborn
Nicholas Evans
Key points
- • There is little outcome-based evidence to guide circulatory support in the newborn.
- • In clinical situations of high risk for circulatory compromise, there are a range of hemodynamics.
- • A “one size fits all” approach to neonatal circulatory support is neither logical nor likely to be successful.
- • It is logical to define the individual hemodynamic in a baby and apply therapy on the basis of those individual findings.
Introduction
Shock refers to a circulatory state where the delivery of oxygen to the organs and tissues of the body is inadequate to meet demand. In neonatology, the terms hypotension and shock have tended to be used synonymously. This is erroneous, and while many shocked babies will be hypotensive, not all hypotensive babies are shocked and not all shocked babies are hypotensive. While true shock is uncommon in neonatology, borderline states of circulatory incompetence are common in sick and very premature babies.
Pathophysiology
Circulatory competence depends on adequate systemic blood flow, which is dependent on cardiac output. Cardiac output is the product of ventricular stroke volume and heart rate. Ventricular stroke volume is determined by preload (positively), myocardial performance (positively), and afterload (negatively, above an individually variable threshold). Thus cardiac output can be increased by increasing stroke volume, heart rate, or both. Blood pressure is the product of cardiac output and systemic vascular resistance (SVR) (related to afterload), so blood pressure can be improved by increasing cardiac output or SVR, or both.
Neonatal circulatory compromise can result from four basic mechanisms: reduced intravascular volume (low preload), intrinsic or extrinsic compromise of myocardial performance, obstruction in the circulation (high afterload), and loss of vascular tone or distributive disorders of the peripheral circulation. In the first three processes the main hemodynamic feature is low systemic blood flow, which results in tissue hypo-perfusion and hypoxia. In contrast, in vasodilatory shock, as long as myocardial performance keeps up with the increased workload, there is a normal or high systemic blood flow. In vasodilatory shock abnormal distribution of the circulation or microcirculatory alterations may play an important role in the development of tissue hypoxia. Microcirculatory shock is the condition in which the microcirculation fails to support tissue oxygenation in the face of normal systemic hemodynamics.1
This complexity is magnified many times in the newborn because we are dealing with a circulation in transition. The fetal channels may not close, leading to blood either bypassing the lungs by shunting right to left or recirculating through them by shunting left to right. This depends on the pressure differentials in the pulmonary and systemic circulation. If the fetal channels do close (as they often do in near-term or term babies) and the pulmonary vascular resistance remains high, then the circulation may be compromised because the blood cannot get through the lungs to reach the systemic circulation.
Hemodynamics may vary between different clinical situations and even between babies in the same clinical situation, so to determine the logical approach to managing shock in an individual baby, there is a need to understand the mechanism of circulatory compromise in that baby and to apply therapy logically on the basis of our understanding of the actions of the pharmacological agents that are available.
This is not an area where a “one size fits all” evidence-based approach is likely to be effective. There is too much variation between babies and in the same baby with time. In this chapter, the author will summarize the important information needed to derive an individual hemodynamic, list the common patterns of abnormal hemodynamics and examine the evidence in relation to available circulatory support interventions in the newborn. Then a logical approach to utilizing these interventions based on hemodynamic scenarios that are commonly found in the newborn in clinical situations of high circulatory risk is suggested.
Relevant hemodynamic information
How to best define an individual hemodynamic is controversial. Many neonatologists still use vital signs, mainly blood pressure, and this will reflect the reality for many with limited access to technologies such as Doppler ultrasound and/or NIRS. But, to define a hemodynamic, one really needs both a measure of pressure and a measure of flow so that resistance can be estimated. From that, it is possible to derive whether it is flow or resistance or both that you need to increase to support pressure.
How best to define flow is also controversial, particularly whether that should be a measure of total systemic flow or a marker of individual organ blood flow, usually the brain. Both are clearly important, and the ideal situation would be to be able to measure systemic and organ blood flow as well as blood pressure. Indeed, the future may be comprehensive, real-time hemodynamic monitoring, as described in Chapter 14. One would also need a measure of preload or volume status, but this marker remains elusive except in cases of extreme hypovolemia. The author would argue that it is difficult to define an individualized approach to the circulation without any information about what is happening in the organ that drives the circulation and, indeed, the organ you are trying to manipulate with your interventions, the heart. Without this knowledge, you are flying blind.
Cardiac ultrasound assessment of an individual hemodynamic is complex, and the measurements will be covered in more detail in the relevant chapters of this book. It is clearly important to document structural normality, fetal channel shunts, volume status, myocardial function, and estimated pulmonary pressure but the essentials for circulatory support are a measure of blood pressure, a measure of systemic blood flow, and in some babies an estimate of pulmonary blood flow. The author uses superior vena cava flow and/or right ventricular output as markers of systemic blood flow and left pulmonary artery velocity as an estimate of pulmonary blood flow. Ideally, in addition, information on blood flow distribution to the organs needs to be available to fully understand the cardiovascular status of the neonate.
Patterns of abnormal neonatal hemodynamics
Neonatal hemodynamics exist on a continuum, not in the discrete categories that are described in the following sections. Notwithstanding this, there are several patterns of abnormal hemodynamics seen in the newborn, and it is the author’s opinion that it is helpful to model your thinking around these categories. While these will be discussed separately below, it must be highlighted that there will be overlap between these hemodynamic patterns in individual babies. It is also important to emphasize the importance of integrating the cardiac ultrasound findings with the clinical history, respiratory/ventilatory status, and vital signs to define the likely diagnosis and individual therapeutic needs. There are essentially five patterns of abnormal hemodynamics found in the newborn. These are summarized in Table 24.1. These patterns are given as follows:
Low systemic blood flow (SBF): RV output <150 mL/kg/min and/or SVC flow <50 mL/kg/min.
Low pulmonary blood flow (PBF): Left pulmonary artery (LPA) mean velocity <20 cm/s.
Minimally acceptable blood pressure: Controversial, MBP use above gestational age suggested.
BP, blood pressure; CHD, congenital heart disease; FBC, full blood count; FO, foramen ovale, iNO, inhaled nitric oxide; LPA, left pulmonary artery; LV, left ventricle, MBP, mean blood pressure; PAP, pulmonary artery pressure; PPHN, persistent pulmonary hypertension of the newborn; RV, right ventricle; SBF, systemic blood flow.
Hypovolemic hemodynamic (see also Chapter 21)
Absolute hypovolemia is rare in the newborn, but it is seen in the immediate postnatal period in babies that have suffered intrapartum fetal blood loss and, postnatally, with subgaleal hemorrhage and post-surgical blood loss. Such babies may have a perinatal or clinical history consistent with blood loss and, on examination, will be pale and invariably tachycardic. If they have progressed beyond the compensatory phase, they will have low blood pressure. The main cardiac ultrasound finding is dramatic poor biventricular filling, so the chambers look small and often have an appearance of poor contractility, reflecting low preload. The walls of the left ventricle may appear to touch each other in systole. The systemic veins will also be poorly filled and measures of systemic blood flow will be low or low normal depending on the degree of hypovolemia.
Hemoglobin falls quickly after blood loss and evidence of a low hemoglobin as measured on a hematocrit or emergency full blood count may assist in diagnosis. Also, most blood gas machines include a hemoglobin measurement, so look for that on your cord gas if one has been performed.
Case history 1
Preterm rupture of membranes at 34 weeks progressed to preterm labor at 36 weeks. Maternal group B streptococcus positive and given intrapartum antibiotics. Ventouse delivery for failure to progress and maternal temperature. No nuchal cord, no intrapartum hemorrhage. Apgars 2 at one minute, 6 at 5 minutes, and 8 at 10 minutes, still pale and unwell looking at 10 minutes.
Cord arterial gas was as follows: pH 7.28, base excess (BE) −7, and lactate 4 mmol/L. On examination, heart rate 190, mean BP 28 mmHg, and respiratory rate 40. Scalp was boggy on examination, with possible small subgaleal hemorrhage. Venous gas at 50 minutes: pH 7.02, BE −15, lactate 8.3 mmol/L, and hemoglobin 90 g/L. Given 20 mL/kg normal saline and cardiac ultrasound performed.
Neonatologist-performed cardiac ultrasound showed poor filling of both ventricles. Figure 24.1 shows the end-systolic frame with the walls of the left ventricle touching each other.
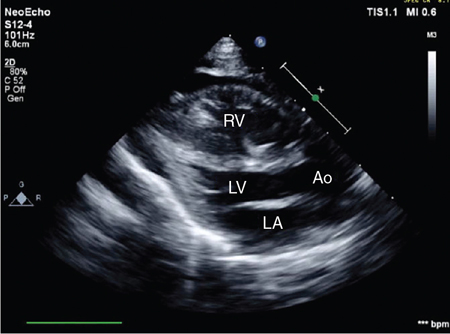
Interpretation: Diagnosis consistent with severe hypovolemia. Given 20 mL/kg of uncrossmatched O-negative blood over 20 minutes with marked clinical improvement. Cardiac ultrasound repeated, and Figure 24.2 shows the improved ventricular filling with the LV walls no longer touching in end-systole. A further 20 mL/kg of blood was given over an hour.
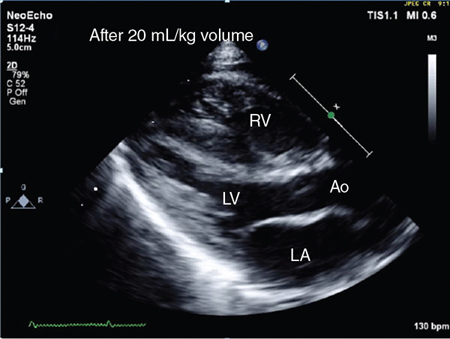
Outcome: Maternal Kleihauer test result consistent with 90 mL of fetal blood in the maternal circulation. Diagnosis of acute hypovolemia from combined effect of an acute fetomaternal hemorrhage and a subgaleal hemorrhage. Cardiac ultrasound and cord arterial gas allowed quick confirmation of diagnosis from the main differential, which would be perinatal asphyxia.
Vasodilatory hemodynamic
This loss of vascular resistance pattern is invariable in hypotensive preterm babies who are more than 24 hours old and can be seen during the first 24 hours, sometimes in combination with low systemic blood flow (see below). It is also seen on recovery from shock or severe asphyxia and is the invariable hemodynamic in late-onset sepsis (see Chapter 21).2 These babies will either be very preterm or have a clinical history consistent with sepsis or asphyxia. They will be hypotensive, often tachycardic, and the impact on other clinical signs and markers of shock such as lactate will depend on severity. The cardiac ultrasound findings will show well-filled ventricles with a good, sometimes hyperdynamic, appearance to the myocardial function, and measures of systemic blood flow will be high normal or high, reflecting the loss of resistance. If treatment is delayed, myocardial function will deteriorate as, after a certain period, myocardial oxygen delivery starts failing to meet tissue oxygen demand.
Case history 2
Preterm baby born at 29 weeks with good initial course, but sudden deterioration on day 7 with clinical sepsis. Full septic screen and commenced on vancomycin and gentamicin. Baby had pale shocked appearance with tachycardia, mean blood pressure of 18 mmHg, and a rising blood lactate. Hypotension, not responsive to both dobutamine and dopamine at 20 mcg/kg/min, was noted. Neonatologist-performed cardiac ultrasound done for investigation of unresponsive hypotension.
Cardiac ultrasound showed a hyperdynamic heart with a closed ductus arteriosus. LV output was very high at 600 mL/kg/min (Normal range [NR] 150–300 mL/kg/min). This can be seen in Figure 24.3, with high velocities in the ascending aorta (left) and middle cerebral artery (right). The normal average maximum velocities are shown by the dotted lines.
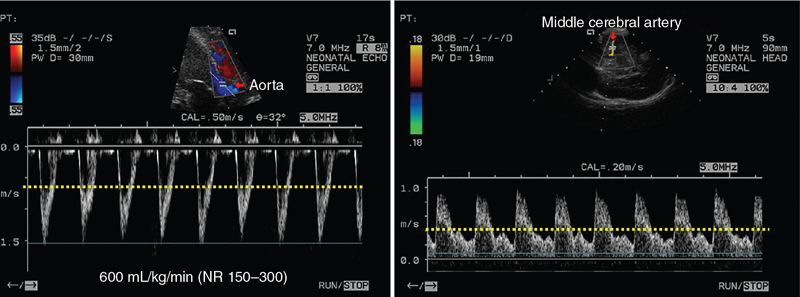
Interpretation: LV output is systemic blood flow when the ductus is closed. High systemic blood flow with low blood pressure is consistent with vasodilatory shock. The therapy was focused on pressors, so dobutamine was ceased. Norepinephrine and then vasopressin were commenced.
Outcome: Blood cultures grew Pseudomonas aeruginosa. The blood pressure was non-responsive to the added pressor therapy and the lactate continued to rise. The baby passed about 24 hours later with ischemic increased echogenicity changes evolving on the cerebral ultrasound. The case highlights that tissue ischemia can occur in the presence of normal or high systemic blood flow, pointing to the role of distributive or micro-circulatory shock.
Low systemic blood flow hemodynamic
This pattern usually reflects absolute or relative myocardial dysfunction with relative myocardial dysfunction, meaning that a myocardium is struggling against increased afterload or, in the case of the preterm myocardium, afterload for which it is not adapted. This is the most common pattern of abnormal hemodynamic seen in the very preterm baby in the first 12 hours after delivery (Chapter 20). It probably reflects maladaptation of the immature myocardium to the higher afterloads of extra-uterine life, possibly compounded by the negative circulatory effects of positive pressure ventilation as well as shunts out of the systemic circulation through the fetal channels.3 It is unusual to find this pattern in preterm babies after the first 24 hours, where the abnormal hemodynamic is invariably vasodilatory.2,3 Low systemic blood flow due to afterload compromise is the usual pathophysiology of the cardiorespiratory compromise seen after PDA ligation,4 and recipients of twin-twin transfusion syndrome seem particularly vulnerable to developing this hemodynamic. Low systemic blood flow is found in more mature babies with high ventilator and oxygen requirements, where primary myocardial dysfunction merges with the PPHN hemodynamic discussed below.5 Low systemic blood flow is also found in clinical situations associated with an injured myocardium, most commonly in the asphyxiated newborn but also in viral myocarditis or congenital cardiomyopathies. It is also important to remember that myocardial compromise can be external to the heart, as in cardiac tamponade.
The impact of low systemic blood flow on vital signs is variable, particularly in the preterm baby, where blood pressure may be normal. Because of this, recognition of low systemic blood flow often depends on proactive use of cardiac ultrasound in the high-risk clinical situations mentioned above. The findings on cardiac ultrasound will be low SVC flow (<50 mL/kg/min) and/or low RV output (<150 mL/kg/min) and, particularly in the more mature baby, poor myocardial function may well be subjectively apparent as well assessed by myocardial function measures. Cardiac tamponade will show as an echolucent surrounding to the myocardium compressing the chambers and impairing contractility.
Case history 3
Spontaneous onset of preterm labor in a 27-week monozygotic twin pregnancy. On delivery, undiagnosed twin polycythemia anemia sequence apparent. Twin 2 was the recipient twin with hemoglobin 257 g/L and hematocrit 72%. Intubated, ventilated, and given surfactant. Mean blood pressure at 4 hours was in the range of 45–50 mmHg. Neonatologist-performed cardiac ultrasound performed because of high-risk hemodynamic situation.
Cardiac ultrasound showed mild myocardial hypertrophy with a 2.0 mm PDA shunting left to right. Figure 24.4 shows the pulsed Doppler traces from which RV output and SVC flow were derived. Both show low velocities suggesting low systemic blood flow. RV output was 145 mL/kg/min (NR 150–300 mL/kg/min) and SVC flow was 41 mL/kg/min (NR 50–100 mL/kg/min).
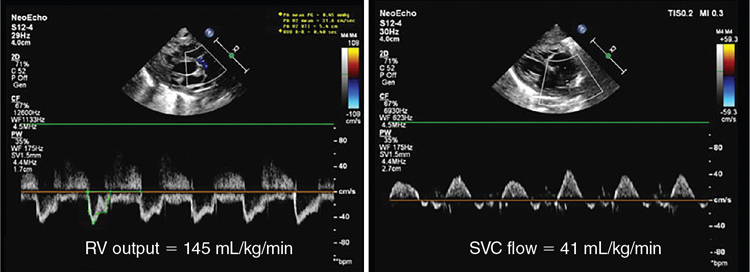
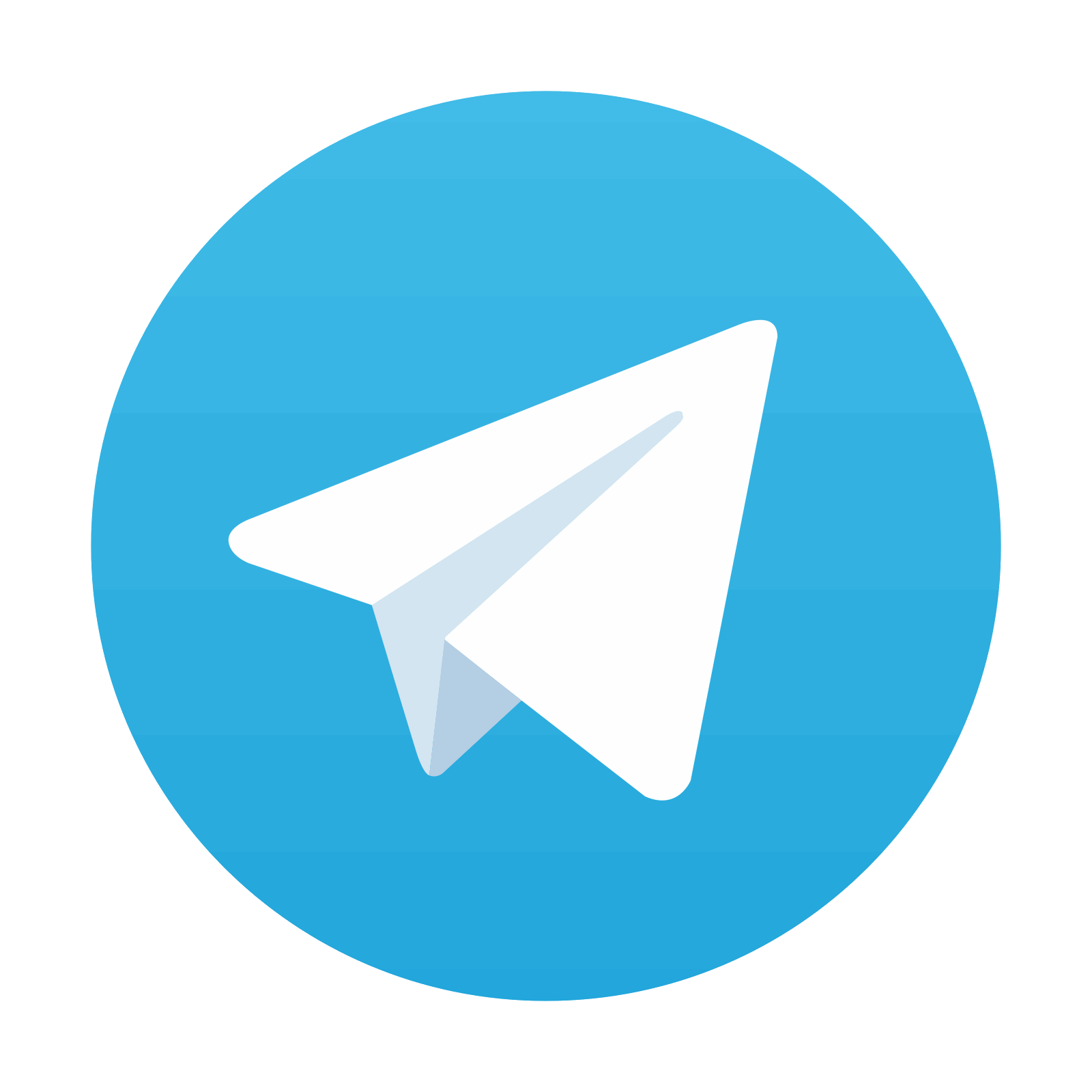
Stay updated, free articles. Join our Telegram channel

Full access? Get Clinical Tree
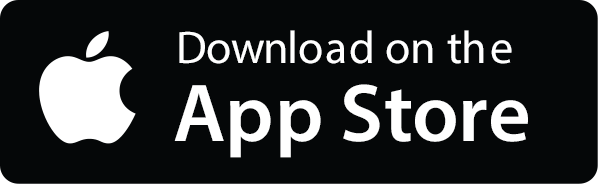
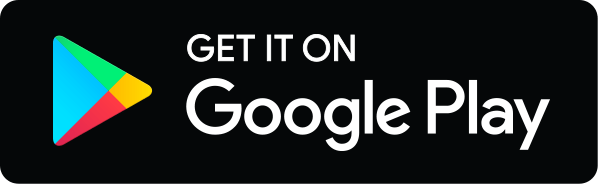