11 Hematology and Oncology
Hematology
Red Blood Cells
The red blood cell classically is described as a biconcave disk. Red cells evolve from immature forms that scarcely reflect the ultimate stage. Pronormoblasts begin as very large cells with dark blue cytoplasm and a large, centrally located nucleus. As the cell matures, the nucleus becomes pyknotic and is eventually extruded from the cell completely. As the cell begins to make hemoglobin, the cytoplasm undergoes a gradual transformation from dark blue to the eventual pink that is seen on peripheral smears.
A peripheral smear is made with a small droplet of blood on a glass slide. A second slide can be used to smear the blood in a manner that covers one half to two thirds the length of the slide. A well-made smear should not have lines or holes, nor should it reach either end of the slide completely. Commonly, Wright stain is applied after drying to give the cells their characteristic appearance (Fig. 11-1). In general, a normal red blood cell has approximately the same size as a lymphocyte nucleus. Red cells all should be the same relative shape and size, with an area of central pallor that is approximately one third the diameter of the cell (Fig. 11-2). A variety of terms are available to describe the physical properties of mature red blood cells. Polychromasia refers to variability in cell color, with hypochromia specifically indicating an increased area of central pallor. Anisocytosis indicates variability in cell size. Microcytosis describes a small cell size, whereas macrocytosis refers to large cells. Poikilocytosis represents variability in cell shape. The overall number of red blood cells in the circulation cannot be described reliably by the peripheral smear. Laboratory values can also aid in assessing red blood cell morphology. Two of these have wide usage. The mean corpuscular volume (MCV) is a laboratory measure of cell size, and red cell distribution width (RDW) estimates this difference.
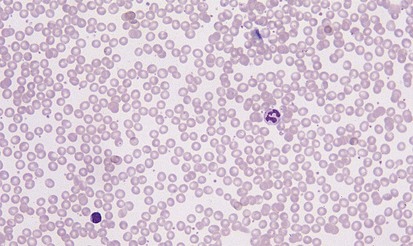
Figure 11-1 Peripheral blood smear: the appearance of a normal peripheral blood smear when viewed under low magnification (×100).
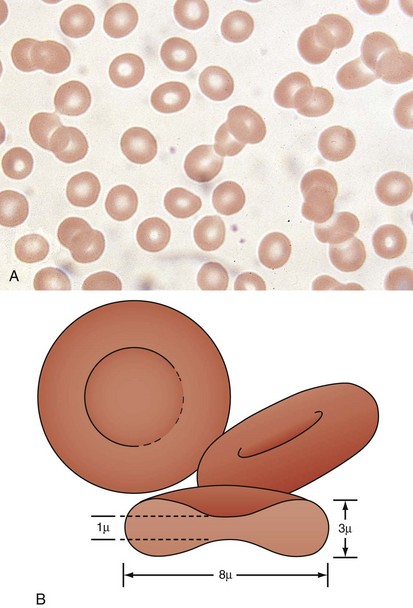
Figure 11-2 Peripheral blood smear: the appearance of a normal peripheral blood smear when viewed under high power (×400). A, Biconcave structure of the red blood cell (RBC). B, Schematic drawing of an RBC in two views, demonstrating the features of the normal biconcave disk.
The primary function of the red blood cell is to deliver oxygen to the tissues. The effective red cell number commonly is represented by the hemoglobin concentration, in grams per deciliter (g/dL). The oxygen-binding sites of hemoglobin are completely saturated by passage of the RBC through the lungs. As the cells circulate through the systemic capillaries, the hemoglobin releases 25% of its bound oxygen to the tissues. However, the amount of oxygen released in the tissue may be significantly increased under certain conditions (fever, acidosis, level of 2,3-diphosphoglycerate), allowing some compensation for a decrease in hemoglobin. Nonetheless, a progressive decrease in the hemoglobin level eventually leads to tissue hypoxia, which triggers a release of erythropoietin. This induces an increase in RBC production, which brings RBC mass back toward normal values.
Different stages of life and disease states impart different oxygen demands, and therefore hemoglobin levels can vary greatly. In general, full-term newborns are born with relatively high levels of hemoglobin that then fall over the first few months of life. This low point, the physiologic nadir, occurs during the transition in production from fetal to adult hemoglobin. Premature infants may have an earlier, more severe, and more prolonged nadir depending on gestational age. After this time, the average hemoglobin value increases until preadolescence, and by puberty, reaches adult levels. Similarly, the MCV is at a low point after the nadir of hemoglobin, and slowly increases into early adolescence. An estimate of the lower limit of normal for the MCV is 70 femtoliters (fL) plus two times the age in years, from ages 1 to 10 years.
Anemia
Classifications and Symptoms
Increased RBC loss or decreased RBC production can result in an overall decrease in RBC mass below a critical level, leading to anemia. This is the most common abnormality of RBCs, and it is identified as a decreased hemoglobin level. At any given age, anemia is defined practically as a value greater than 2 standard deviations below the mean (Table 11-1). However, physiologically, anemia is best defined as a hemoglobin level that is too low to meet tissue oxygen demands. Conceptually, anemia occurs as a result of one of the following: decreased bone marrow production of red blood cells (RBCs) and reticulocytes (Fig. 11-3), increased destruction of mature RBCs peripherally or as precursors in the bone marrow, or hemorrhage. In addition, a combination of these factors can occur.
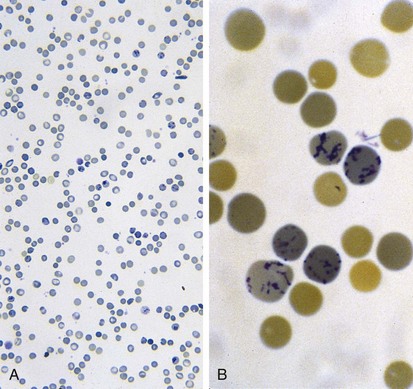
Figure 11-3 A, The reticulocyte: a reticulin-stained peripheral blood smear in a patient with a high (18%) reticulocyte count. The darkly stained cells are reticulocytes seen in the peripheral blood of a patient with hemolytic anemia. B, High magnification of reticulocytes.
Recognition that a child’s hemoglobin level is abnormal requires knowledge of age, gender, and race-related normal values. Severe anemia from any cause may elicit symptoms of fatigue; decreased appetite; headache; and, in extreme cases, shock, congestive heart failure, or even stroke. Physical examination of the anemic child may reveal pallor, although in the fair-skinned or dark-skinned child this may be easily missed, unless palmar creases or conjunctivae are also examined thoroughly (Fig. 11-4). Vital signs may be normal, but with severe anemia, tachycardia may be present. In practice, it is common for anemias to be classified on the basis of their cellular morphology, especially size and coloration. The most common scenario encountered in pediatrics is a microcytic anemia due to iron deficiency.
Iron Deficiency
A lack of iron leads to failure of hemoglobin production. Affected children may manifest a peculiar physical finding termed koilonychia, or “spooning” of fingernails and toenails (Fig. 11-5). Glossitis may be observed in iron deficiency and other nutritional deficiencies. Iron deficiency, however, is only a laboratory finding, not a diagnosis. The causes of iron deficiency must be elucidated through a careful history and physical examination. Although poor nutrition is the most common cause, other causes such as hemorrhage or malabsorption must also be considered. Iron deficiency anemia occurs in infants whose rapidly increasing RBC mass outstrips the dietary iron intake. Because the normal full-term infant has adequate iron reserve to accommodate the increasing RBC mass through the first 5 months of life, iron deficiency is usually not seen until the second half of the first year of life. It is detected most often in the 10- to 18-month-old child who ingests large volumes of cow’s milk and little else. Whole cow’s milk not only is deficient in dietary iron but often leads to an enteropathic condition with occult gastrointestinal blood loss, exacerbating the child’s iron-deficient status. A second peak of iron deficiency anemia is seen during adolescence in girls.
Figure 11-6 shows a peripheral blood smear of a child with iron deficiency anemia. The RBCs are microcytic and hypochromic. The number of platelets often is increased (particularly when an enteropathic condition is present), although it may be normal or even decreased. In severe iron deficiency anemia, anisocytosis and poikilocytosis may be prominent. Nonspecific abnormalities of RBC morphology, such as the presence of micro-ovalocytes (see Fig. 11-6) or basophilic stippling (see Fig. 11-8), may also occur. Bizarre RBC shapes are due to a three-dimensional change in structure when viewed in a two-dimensional plane of the light microscope. When viewed in two dimensions, the cells appear ovoid.
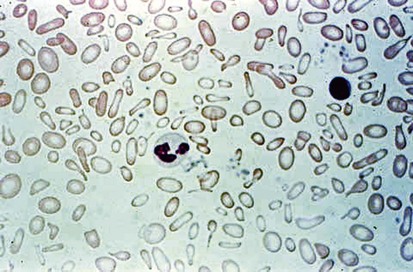
Figure 11-6 Iron deficiency results in a hypochromic, microcytic anemia. This 16-month-old patient had a history of excessive milk intake. Note the marked central pallor of the red blood cells (RBCs) with their small rim of hemoglobin, as well as their small size in comparison with that of the adjacent lymphocyte nucleus. Frequent “cigar cells” can be seen in addition to the hypochromic microcytic RBCs.
The history and physical examination, blood count, and review of the peripheral blood smear may be sufficient for diagnosis in many instances of hypochromic, microcytic anemia. If the evaluation before obtaining a more specific test strongly suggests iron deficiency, a therapeutic trial of iron may be a reasonable approach. However, additional laboratory studies are often indicated. The coexistence of iron deficiency and lead intoxication is well documented, and partial response to iron therapy may indicate concurrent lead intoxication. Securing long-term follow-up of the patient is also imperative. Supplementation with oral iron must continue for approximately 2 months after return of the hemoglobin value to normal to allow for the repletion of body iron stores. The etiology of the iron deficiency anemia also must be addressed to prevent recurrence.
Choosing between specific studies such as serum iron levels and total iron-binding capacity (TIBC) or ferritin and free erythrocyte protoporphyrin (FEP) is often a matter of personal preference. In addition, when the degree of anemia is mild, the results of any combination of tests may be equivocal. Importantly, normal values of ferritin, serum iron, TIBC, and transferrin saturation demonstrate age-related variation. Ferritin is an acute-phase reactant and may be elevated into the low normal range in a child with a concurrent inflammatory process. However, if ferritin is low, it is diagnostic of iron deficiency. Low transferrin saturation and elevated FEP are also observed in the setting of iron deficiency.
Lead Poisoning
Lead intoxication also leads to microcytic anemia. However, nonhematologic manifestations of lead intoxication, particularly neurologic complications, often dominate the picture. The spectrum of clinical presentations of lead intoxication ranges from vague symptoms of abdominal pain, vomiting, malaise, and behavioral changes to acute encephalopathic conditions, with rapid progression to coma and death. Late physical findings may include papilledema. Significant radiographic changes are also seen in lead intoxication (Fig. 11-7).
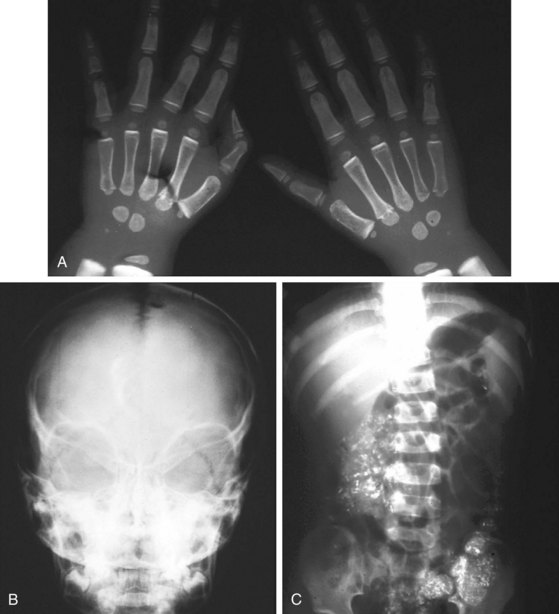
Figure 11-7 X-ray findings in lead intoxication. A, This hand radiograph of a child with lead intoxication reveals marked linear increases in the density of the metaphyses. These should not be confused with the growth arrest lines that may be seen after a variety of illnesses. B, A skull film is shown of a patient with lead intoxication and encephalopathy. Note the split of sutures indicative of increased intracranial pressure. C, An abdominal radiograph performed on a child with a history of pica and lead intoxication reveals radiodense, lead-containing paint chips scattered throughout the colon.
Lead intoxication occurs as a result of excessive environmental lead intake. Aerosolized and oral lead-containing environmental contaminants are major sources of lead intoxication. Although clearly a common mechanism in lead poisoning, pica associated with the intake of flaking lead paint represents neither the only nor the prevailing cause of lead intoxication. The finger-sucking behavior of children in homes where lead paint has become a part of house dust is perhaps a more important factor. A contaminated water supply resulting from old plumbing and deteriorating lead-containing soldered joints can cause lead intoxication in the infant (earlier than the usual at-risk age).
Hematologic abnormalities of lead intoxication are a direct result of the effect of lead on several cellular enzymes involved in heme production. Lead inhibits these enzyme systems, impairing iron use and globin synthesis. Thus despite normal intracellular levels, iron is unable to be incorporated into heme and hemoglobin production fails. Reduced hemoglobin production leads directly to hypochromic, microcytic anemia. Basophilic stippling (Fig. 11-8) is a secondary and inconsistent hematologic manifestation of lead intoxication that occurs as a result of inhibition of yet another RBC enzyme, pyrimidine 5′-nucleotidase. Although basophilic stippling is more prominent in lead intoxication, it is also present in thalassemia and treated iron deficiency. Its presence on the peripheral smear is nonspecific.
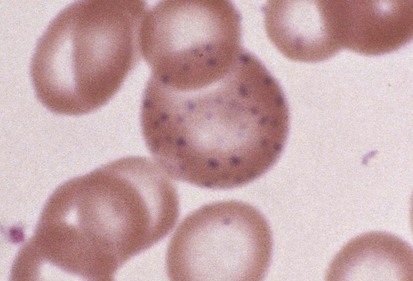
Figure 11-8 Lead intoxication leads to a hypochromic, microcytic anemia. Prominent basophilic stippling is often seen in cases of severe lead intoxication. However, this finding is not specific for lead toxicity and may also be seen in thalassemia and treated iron deficiency.
Differentiating lead intoxication from iron deficiency can, at times, be difficult. Also, lead intoxication and iron deficiency may coexist, further confusing the diagnosis. Lead poisoning and iron deficiency cause FEP to accumulate in the blood because of the inability to complete the heme biosynthetic pathway through the incorporation of iron into heme. The FEP is elevated in both conditions, although extremely high levels are more common in lead intoxication. Further testing, such as determination of blood lead level, is necessary to determine the cause of elevated FEP levels. The fact that even low-level lead intoxication may disturb cognitive function underscores the necessity for clinicians to consider this diagnosis.
Anemia of Inflammation
Inflammatory diseases, such as chronic infection or collagen vascular disease (particularly juvenile rheumatoid arthritis), may lead to hypochromic, microcytic anemia. Although the symptoms of the child’s primary illness usually clarify the diagnosis, there are occasionally cases in which a chronic subclinical infection (particularly of the urinary tract) may go undiagnosed. Differentiating a chronic inflammatory state from other causes of hypochromic, microcytic anemia is usually more easily done on clinical rather than laboratory grounds. One laboratory study that may be of value is serum ferritin determination. Ferritin is decreased in iron deficiency, whereas in chronic inflammatory states it is usually elevated. Also, whereas serum iron is low in both iron deficiency and anemia of chronic disease, TIBC is increased in iron deficiency and decreased in anemia of chronic disease.
Megaloblastic Anemia
Although the etiology of the megaloblastic anemias may vary, common morphologic abnormalities of erythropoietic cells exist. In the bone marrow, the hallmark is the megaloblast, a nucleated RBC with a lacy chromatin pattern and a dyssynchrony of maturation between cytoplasm and nucleus. The morphologic alterations are a direct result of decreased nucleoprotein DNA synthesis compared with cytoplasmic protein synthesis, which stems from a relative decrease in the factors needed in DNA replication, namely folate and cobalamin (vitamin B12).
On the peripheral blood smear, the RBCs are large in size (macrocytes) and display a great deal of variation in their shapes (Fig. 11-9). These macrocytic cells are generally normochromic. In addition, all of the actively dividing marrow cells may become involved in the pathologic process. Neutrophils are the second most likely cells to display morphologic abnormalities. These cells become large and show pathognomonic hypersegmentation of the nucleus (Fig. 11-10). Neutropenia is common. The more severe and prolonged megaloblastic anemias ultimately may lead to moderate thrombocytopenia, with large bizarre platelets on the peripheral blood smear. Megaloblastic changes in each cell line are shown in Figure 11-11.
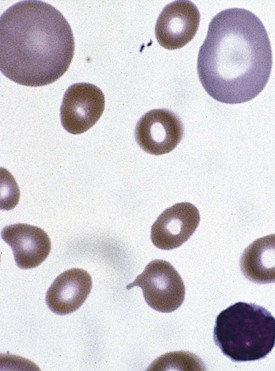
Figure 11-9 Megaloblastic anemia. This peripheral blood smear demonstrates an enlarged red blood cell (RBC, macrocyte) at the upper left of the photograph. The RBC is much larger than the normal small lymphocytes in the same field in this patient with megaloblastic anemia.
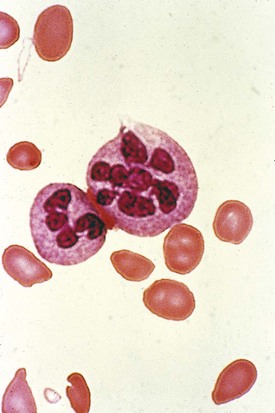
Figure 11-10 This peripheral blood smear demonstrates the presence of a hypersegmented polymorphonuclear leukocyte in a patient with phenytoin-induced folate deficiency.
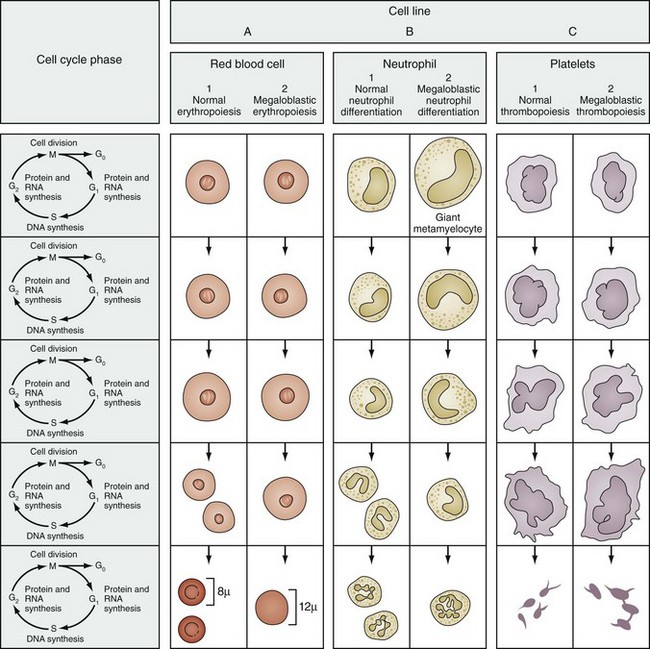
Figure 11-11 Schematic comparison of normal cellular maturation and megaloblastic differentiation of three cell lines. The cell cycle phase is identified to the left of the figure. Note that the megaloblastic cells fail to undergo replication of DNA and cellular division at the S and M phases, respectively, leading to large red blood cells, hypersegmented polymorphonuclear leukocytes, and large, bizarrely shaped platelets.
Laboratory diagnosis requires the measurement of folate and vitamin B12 levels in the serum and RBCs. Because a typical Western diet is unlikely to lead to folate or vitamin B12 deficiency, a low level of either should raise questions of altered bioavailability or peculiar diet. Goat milk is folate deficient (although in more recent years many canned goat milk products are folate supplemented), and an infant on a goat milk diet may become folate depleted over time. Fad diets often are not structured thoughtfully and may lead to folate deficiency. Various drugs that decrease folate absorption (phenytoin) or interfere with folate metabolism (methotrexate) may also lead to megaloblastic changes. A pathologic condition of the gastrointestinal tract may cause malabsorption of folate or vitamin B12 and secondary megaloblastic changes. This condition may be iatrogenic if a portion of the intestines is removed for medical reasons. Pernicious anemia is a specific cause due to a deficiency of intrinsic factor, which is required for vitamin B12 absorption. Glossitis (Fig. 11-12) or angular stomatitis, commonly seen in vitamin B12 deficiency, can be helpful physical findings in the diagnostic process.
Pure Red Cell Aplasia
Transient erythroblastopenia of childhood (TEC) occurs in 1- to 4-year-old children and appears 2 weeks to 2 months after a respiratory or gastrointestinal illness. TEC is transient and self-limited, whereas the other forms of red cell aplasia may be chronic. It has been noted that the RBCs in pure red cell aplasia have fetal characteristics, whereas the RBCs of TEC have age-appropriate characteristics (Table 11-2). The cause of TEC remains obscure. TEC may occur after many different viral infections and most likely represents an altered immunity from the infection that affects erythropoiesis. Treatment is supportive and determined by the degree of symptoms.
Table 11-2 Features Differentiating Pure Red Cell Anemia from Transient Erythroblastopenia of Childhood
disease | ||
---|---|---|
RBC Characteristic | Pure Red Cell Anemia | TEC |
Hemoglobin | Increased fetal | Normal fetal |
Cellular antigen | i | I |
Mean corpuscular volume | Increased | Normal |
RBC enzyme activity | Normal or high | Low |
RBC, red blood cell; TEC, transient erythroblastopenia of childhood.
Non–TEC-acquired pure red cell aplasia is rare in pediatrics. Three types of non–TEC-acquired pure red cell aplasia have been recognized. Type I has a serum IgG inhibitor of erythropoiesis and high erythropoietin levels. Type II has a low erythropoietin activity level and an erythropoietin IgG antibody. Type III has no anti-erythropoiesis or anti-erythropoietin serum antibodies. Rather, the defect appears intrinsic to the stem cells. Some of these children are preleukemic. Thymoma-associated pure red cell aplasia is extremely rare in children. Drugs, particularly chloramphenicol, have been responsible for a significant percentage of pure red cell aplasias historically. The peripheral blood smear from a patient with pure red cell aplasia is often nonspecific, and the bone marrow aspirate rarely is more specific. It may demonstrate the vacuolated erythroblasts of the chloramphenicol effect or the multinucleated giant cells indicative of the rare congenital dyserythropoietic anemia.
Hemolytic Anemias
Hemolytic anemias are defined by the premature destruction of red blood cells. This can occur by mechanical, infectious, enzymatic, or immune-mediated mechanisms. Symptoms and severity vary not only among individuals but also between diagnoses. Jaundice, especially scleral icterus, as well as splenomegaly and dark urine can be common presentations during hemolytic episodes. Although all hemolytic anemias may have acute exacerbations, some also feature more chronic phases of red cell breakdown. The history of individual patients, laboratory studies, and blood cell morphology are important factors in making the accurate diagnosis. Treatments can vary; often, splenectomy may be a consideration. However, splenectomy in children is fraught with dangerous sequelae—both infectious risks and vascular injury or thromboses. Some of the most common features are described in Table 11-3.
Table 11-3 Common RBC Hemolytic Disorders by Predominant Morphology*
Spherocytes |
Bizarre Poikilocytes |
Elliptocytes |
Spiculated or Crenated RBCs |
Prominent Basophilic Stippling |
Irreversibly Sickled Cells |
Intraerythrocytic Parasites |
Target Cells |
Nonspecific or Normal Morphology |
Hgb, hemoglobin; HMP, hexose-monophosphate shunt; RBC, red blood cell.
* Nonhemolytic disorders of similar morphology are enclosed in parentheses for reference.
† Usually associated with a positive Coombs test.
‡ Disease sometimes associated with this morphology.
Modified from Nathan DG, Oski FA, editors: Hematology of infancy and childhood, ed 2, Philadelphia, 1981, WB Saunders.
Hereditary spherocytosis (HS) is the most common cause of genetically determined hemolytic anemia in the white population. HS is transmitted frequently as an autosomal dominant trait, and it is named for the peculiar appearance of the RBCs on the peripheral blood smear (Fig. 11-13). The RBC membrane defect, which most commonly results from inherent cytoskeletal membrane instability due to spectrin deficiency, leads to loss of membrane. Membrane repair occurs, which decreases the normal RBC surface-to-volume ratio; this causes the normal biconcave disk to assume a more geometrically efficient spherical shape (Fig. 11-14). The new morphology creates a less pliable cell. The inability of this new cell to deform during transit through the splenic microcirculation leads to RBC destruction (Fig. 11-15). Osmotic fragility testing, used commonly in patients with suspected hereditary spherocytosis, is an excellent confirmatory test but is not pathognomonic for the diagnosis.
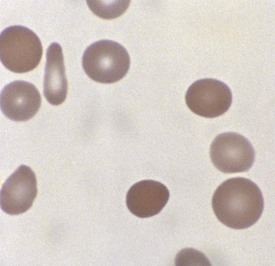
Figure 11-13 Peripheral blood smear of a patient with hereditary spherocytosis. Note the presence of small, perfectly round cells without an area of central pallor. The mean corpuscular hemoglobin concentration (MCHC) is often increased in this condition but may be normal in some cases. Reticulocyte count is also often elevated owing to the shortened life span of the RBCs and need for increased RBC production relative to individuals without hereditary spherocytosis.
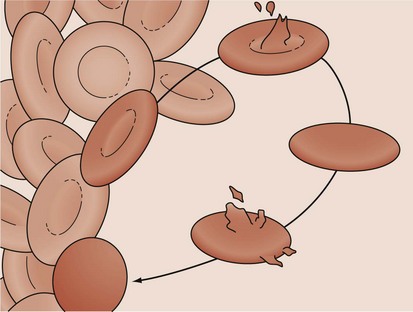
Figure 11-14 A developing spherocyte resulting from the process of repeated membrane fragmentation, loss, and repair.
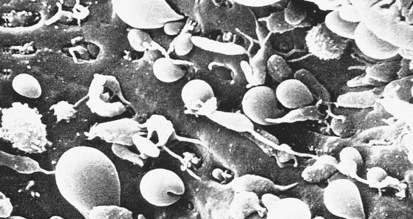
Figure 11-15 Electron micrograph of red blood cells (RBCs) traversing the splenic sinusoids. Note that the RBCs become deformed as they traverse the microcirculation of the splenic sinusoids.
Hereditary elliptocytosis (HE) is another membrane defect morphologically distinct from hereditary spherocytosis (Fig. 11-16). However, its inheritance and pathophysiology of RBC destruction are similar to that in spherocytosis. In most instances, HE is a mild, well-compensated hemolytic anemia that is clinically insignificant unless splenic hypertrophy develops from another disease process. The elliptocyte form bears only a superficial similarity to that of the ovalocyte of iron deficiency anemia. Unlike those cells, the elliptocytes of HE have normal size, have a normal MCV, and are true elliptocytes. Elliptocytes may also be found in the peripheral blood smear of thalassemia or in megaloblastic anemia—although these findings do not suggest mixed pathologies.
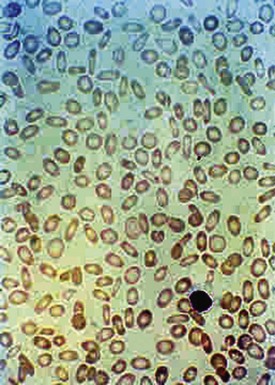
Figure 11-16 This peripheral blood smear resulted in an incidental finding of hereditary elliptocytosis in a 3-year-old child. More than 90% of the cells are elliptocytes. The child’s only remarkable history was one of neonatal jaundice.
A number of hemolytic anemias are characterized by spiculated RBCs referred to as acanthocytes or echinocytes (Fig. 11-17). Abetalipoproteinemia generally presents as a neurologic disorder with progressive ataxia, retinitis pigmentosa, fat malabsorption, and the absence of chylomicrons and very low–density and low-density lipoproteins. Acanthocytes develop as a direct result of the alterations of the serum lipids. Altered membrane lipid composition changes the fluidity of the RBC, leading to the abnormal form. Malabsorption of fat-soluble vitamins in abetalipoproteinemia results in vitamin E deficiency, leaving the RBCs subject to oxidative injury. However, despite altered membrane fluidity and vitamin E deficiency, hemolysis in abetalipoproteinemia is mild.
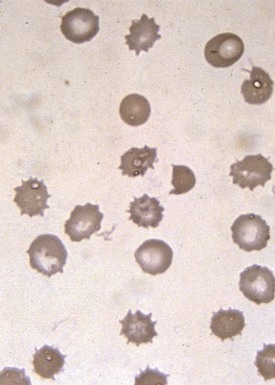
Figure 11-17 Spur cell anemia. The peripheral smear from this patient demonstrates the hematologic findings of acute hepatic necrosis. The anemia associated with the presence of these spiculated cells in the periphery can be severe.
Spur cell anemia is another disorder characterized by acanthocytes. The hemolysis in this disorder, in contrast to abetalipoproteinemia, is brisk. Spur cell anemia develops in the setting of sudden and massive liver injury arising from any cause (e.g., hepatitis with acute yellow atrophy, shock liver, hepatic infarction). The hepatic decompensation leads to increased serum lipid and cholesterol levels, which lead, in turn, to increased RBC membrane cholesterol content, thus altering RBC membrane fluidity. Spiculated cells in lesser numbers can also be seen in uremia and anorexia nervosa, as well as severe malnutrition. Conversely, one of the most frequent causes of spiculated RBCs on the peripheral blood smear is inadequate slide preparation.
Target cells draw their name from their characteristic appearance on the peripheral blood smear. They are often seen as a secondary response to a process that increases the RBC membrane or decreases the RBC content, leading to an increase in the surface-to-volume ratio of the RBCs (Fig. 11-18, A). In a dried smear, the excess surface accumulates and bulges outward in the area that is normally the RBC’s central pallor, producing the characteristic target cell morphology. Liver diseases of any type, particularly obstructive hepatopathy, are well-known causes of target cell formation. Splenectomy decreases reticuloendothelial remodeling of reticulocytes, removes lipid-loaded RBC membranes, and leads to targeted RBCs. Mechanisms previously discussed that decrease RBC intracellular content, such as hypochromic, microcytic anemia, also induce target formation. In addition, target cells (or more accurately, pseudotarget cells) occur with various hemoglobinopathies. This happens more often in conditions associated with Hgb C, but also with Hgb S, D, and E (Figs. 11-19 and 11-20), because of aggregation of hemoglobin in the central region of the RBC (Fig. 11-18, B).
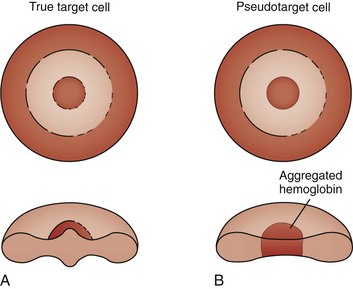
Figure 11-18 A, Schematic of the morphology of a target cell. B, Schematic of the morphology of a pseudotarget cell.
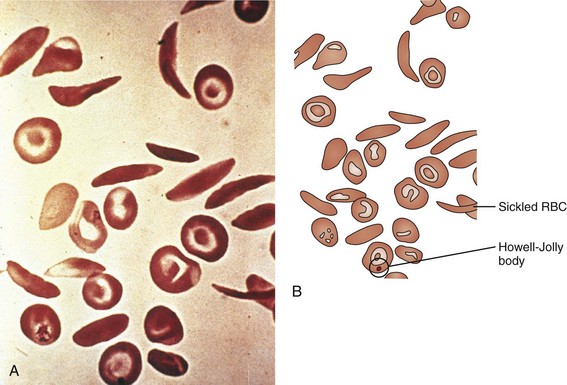
Figure 11-19 A, Peripheral blood smear in sickle cell disease. Note the presence of sickle cells and prominent target cells. A Howell-Jolly body is also present, consistent with auto-infarction and subsequent loss of splenic function. B, Schematic of sickle cell and Howell-Jolly body.
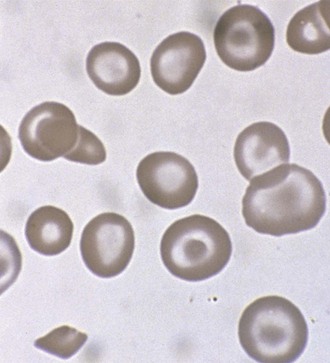
Figure 11-20 Peripheral blood smear of a child with hemoglobin SC disease. Sickle cells are seen less frequently in this disease, whereas target cells are more prominent.
Malaria is the most frequent cause of hemolysis on a worldwide scale. The patient who contracts the disease after being fed on by the tropical Anopheles mosquito is parasitized within the RBCs with organisms at the merozoite stage (Fig. 11-21). The parasitization causes a clinical picture of intermittent fever, chills, and jaundice and may lead to encephalopathy, massive hemolysis with hemoglobinuria (blackwater fever), and death. The cause of the hemolysis has been attributed to multiple mechanisms including altered RBC osmotic fragility, membrane loss of negative surface charge, direct injury by the parasite, autoimmunity, splenic pitting, and hypersplenism.
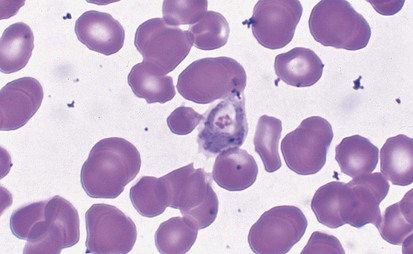
Figure 11-21 Plasmodium vivax malaria. This peripheral blood smear is prepared by the so-called “thick prep” method. Plasmodium vivax malaria is seen intracellularly in the red blood cell in the center of the smear.
Abnormalities of RBC enzymes may also lead to hemolysis. Although almost any RBC enzymes involved in RBC glycolysis or free radical detoxification via the pentose shunt may be responsible for hemolysis, glucose-6-phosphate dehydrogenase (G6PD) deficiency is by far the most frequent. A second enzyme, pyruvate kinase (PK), when deficient, also leads to a hemolytic state. The blood smears of patients with RBC enzyme deficiency–induced hemolysis are often normal, although occasionally with G6PD deficiency a suspicious morphology may be present (Fig. 11-22). More than 370 variants of G6PD have been identified, for which enzyme activity may be normal, elevated, or severely deficient. G6PD deficiency is transmitted by a sex-linked recessive mode of inheritance. Heterozygous females may rarely be affected because of inactivation of the X chromosome according to the Lyon hypothesis or because they are doubly heterozygous. Clinical syndromes may vary as well, with the degree of hemolysis inversely paralleling the level of G6PD activity.
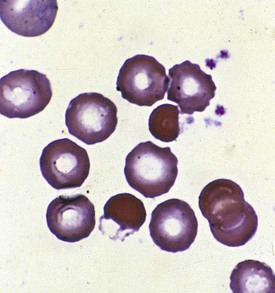
Figure 11-22 Peripheral blood smear of a patient with glucose-6-phosphate dehydrogenase (G6PD) deficiency. This patient is experiencing a hemolytic crisis. Note the blister cells with hemoglobin condensed in the remaining (nonblistered) portion of the cell.
In all instances the hemolysis is due to the intracellular generation of free radicals and peroxides, which fail to be detoxified by the patient with G6PD deficiency. Chronic hemolysis is extremely mild and subclinical in the common types. Triggered hemolysis associated with G6PD deficiency (Mediterranean type) is severe and abrupt and parallels inversely the severe enzyme deficiency. In contrast, triggered hemolysis with G6PD deficiency (A type) may be severe but self-limited when the enzyme deficiency is mild. In addition, some agents that trigger hemolysis with G6PD deficiency (Mediterranean type) may be tolerated by patients with G6PD deficiency (A type). A list of some drugs associated with clinically significant hemolysis in G6PD deficiency is provided (Table 11-4). Research has deemphasized the role of certain drug triggers. In many instances the infection being treated with certain drugs, rather than the drugs themselves, is responsible for the hemolysis.
Table 11-4 Drugs Associated with Clinically Significant Hemolysis in Glucose-6-phosphate Dehydrogenase Deficiency
Antimalarials |
Antipyretics and Analgesics |
Sulfa Drugs |
Miscellaneous |
Pyruvate kinase (PK) deficiency is the second most prevalent enzyme abnormality of the RBC that leads to hemolysis; however, it is a far second, indeed, with 1 case of PK deficiency occurring worldwide for 500,000 cases of G6PD deficiency. Autosomal recessive transmission of the enzyme defect is usually observed in PK deficiency. Like G6PD deficiency, wide clinical variability exists with PK deficiency. Mild, fully compensated hemolytic anemia, as well as severe neonatal hemolysis and hyperbilirubinemia, may occur. Hemoglobin levels range from 6 to 10 g/dL with normochromic anemia with normocytic or macrocytic indices, depending on the degree of reticulocytosis. The reticulocyte count can range from 5% to as high as 90% in the patient who has had a splenectomy. For the subgroup of patients with severe, transfusion-dependent disease, splenectomy may ameliorate or eliminate the need for transfusions. In addition to chronic hemolysis, PK deficiency may have triggered episodes (usually resulting from intercurrent infection).
Direct and indirect Coombs tests, which evaluate the patient’s blood for the presence of anti-RBC antibody and complement on RBCs or in the serum, respectively, identify immune-mediated hemolytic anemia. Antibody- and complement-mediated RBC destruction produces spherocytes on the peripheral blood smear (Fig. 11-23). Coombs-positive hemolytic anemia in the newborn most often represents an isoimmune hemolytic anemia. This is caused by a maternal antibody that has crossed the placenta into the neonate, hemolyzing the newborn RBCs. Maternal antibodies form to fetal RBC antigens when fetal blood gains entry into the maternal circulation via a break in placental integrity. Maternal antibodies then cross the placenta and cause fetal RBC hemolysis. In the majority of cases of isoimmune hemolytic anemia, the maternal antibody is directed at ABO or Rh RBC antigens. When Rh antigen is the antibody target, the blood smear does not show spherocytes.
Hemoglobinopathies
Hemoglobinopathies often result in a characteristic and even diagnostic peripheral blood smear. They can be responsible for hemolysis resulting from unstable hemoglobin variants or decreased hemoglobin solubility. Baseline levels of hemoglobin can be much lower in patients with these disorders. Nearly all of the following diagnoses are life-long conditions.
Of the hemoglobinopathies that have altered hemoglobin solubility, none is as well known or as ubiquitous as sickle cell disease. Substitution of valine for glutamic acid at position 6 in the β chain of the hemoglobin molecule leads to the cross-linking of one β chain to a second hemoglobin molecule’s β chain when the hemoglobin is in its deoxygenated state. This cross-linkage tips the solubility balance, leading to sickling of the RBC (see Fig. 11-19). Sickle cell disease is by no means exclusive to patients of African origin, with Mediterranean and Middle Eastern peoples also being affected. Sickle cell disease is an autosomal recessive disorder with the heterozygote having a significant but less than 50% proportion of hemoglobin of the sickle cell type. Heterozygous carriers of the sickle cell gene, although suffering a number of difficulties (e.g., poor urine-concentrating ability, occasional episodes of renal papillary necrosis with hematuria), have normal life expectancies and are virtually free of any significant consequences of their heterozygous state.
The clinical signs and symptoms of sickle cell anemia are due to decreased survival and altered rheology of the sickled RBC. As with any chronic hemolytic state, marrow cavity enlargement occurs, leading to maxillary hyperplasia and the so-called sickle cell facies (Fig. 11-24). Sickle cells have altered pliability and lose the ability to deform in the microcirculation (see Fig. 11-15). This leads to tissue infarction and subsequent pain. These vaso-occlusive crises, when occurring in certain locations, give rise to clinical manifestations such as dactylitis (Fig. 11-25), priapism (Fig. 11-26), splenic sequestration, and skin ulceration. The vaso-occlusive phenomenon may also lead to life-threatening complications of sickle cell disease: overwhelming infection with encapsulated organisms (most often Pneumococcus), acute chest syndrome, and stroke. The increased infectious risk in the sickle cell patient has multiple mechanisms, but by far the most important is splenic dysfunction related to congested blood flow and then, ultimately, splenic infarction.
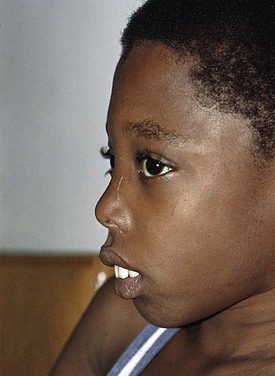
Figure 11-24 Maxillary hyperplasia. Prominent facial bones of a child with sickle cell anemia, due to enlargement of the marrow cavity in this condition of decreased red blood cell survival.
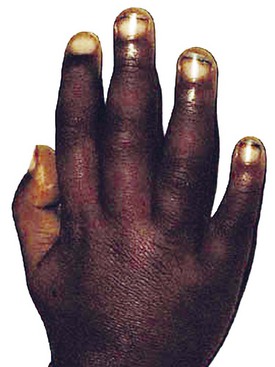
Figure 11-25 Dactylitis (hand–foot syndrome). This syndrome, in children with sickle cell disease, primarily affects toddlers. Dactylitis is seen less frequently in older children because the bone marrow of the small bones of the hands and feet loses hematopoietic activity. This loss of marrow activity is due to cortical thickening from increased use of hands and weight bearing by the feet.
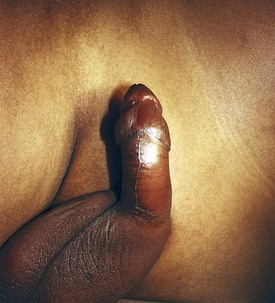
Figure 11-26 Priapism in an adolescent with sickle cell disease. Erection had persisted for 12 hours and had become extremely painful for the patient.
Thalassemia is a term applied to a group of genetic disturbances decreasing hemoglobin production and leading to anemia and/or altered levels of the various hemoglobins in the blood. Thalassemia trait is another cause of hypochromic, microcytic anemia. In patients with β-thalassemia trait, hemoglobin electrophoresis usually reveals an increase in Hgb A2 and Hgb F, whereas in patients with α-thalassemia trait the findings may be normal. β-Thalassemia major generally presents after the first 6 months of life, at a time when β-chain synthesis increases. Hydrops fetalis may result from α-thalassemia major. Ethnicity may be an important clue in the history: β-thalassemia trait tends to occur in patients of African or Mediterranean descent, whereas α-thalassemia trait tends to occur in patients of African or Asian origin. β-Thalassemia major is largely restricted to people of Mediterranean or Middle Eastern heritage.
β-Thalassemia major (Cooley anemia) causes hypochromic, microcytic anemia that results from ineffective erythropoiesis caused by an imbalance between α- and β-hemoglobin chain synthesis. The peripheral blood smear shows hypochromia; microcytosis; target cells; basophilic stippling; and, often, a large number of nucleated RBCs (Fig. 11-27). β-Thalassemia major is associated with increased marrow activity, which is ineffectively attempting to correct the degree of anemia. The increased marrow activity expands the marrow cavity, producing a characteristic bony hyperplasia evidenced by physical and radiographic findings (Fig. 11-28). Untreated patients with thalassemia major have chronic and severe anemia, marked hepatosplenomegaly, scleral icterus, and listlessness and may have high-output cardiac failure secondary to severe anemia. In addition, malocclusion may occur because of molar hypertrophy. This picture is not usually confused with thalassemia trait, iron deficiency, or lead poisoning.
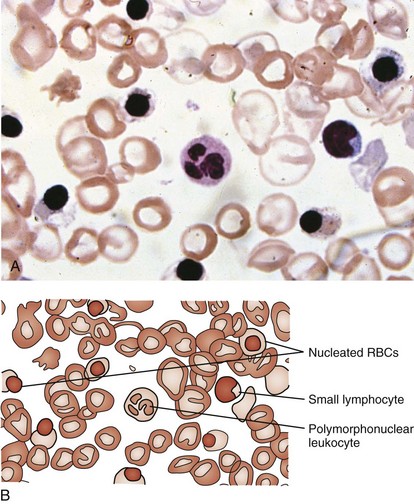
Figure 11-27 Thalassemia major. A, This peripheral blood smear of a child with thalassemia major shows hypochromic, microcytic anemia with prominent nucleated red blood cells surrounding the polymorphonuclear cells and lymphocytes. B, Labeled schematic of peripheral blood smear.
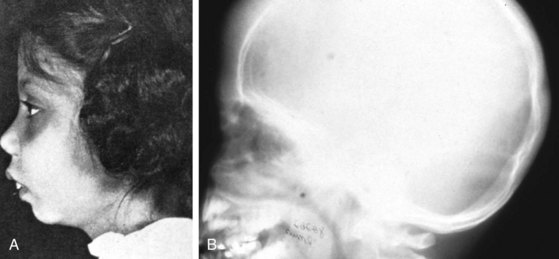
Figure 11-28 A, Maxillary hyperplasia resulting from an increased marrow space in a child with thalassemia major. B, Skull radiograph of the same patient demonstrates an increased marrow cavity of the skull and facial bones.
Although less common than sickle cell disease, anemia associated with hemoglobin C is not rare in the African-American population. As in sickle cell disease, Hgb C disease occurs because of one amino acid change. The change, again like Hgb S, is at the sixth position of the β chain but the replacement is a lysine rather than a valine. In its homozygous form, Hgb C disease is a mild disorder characterized by hemolytic anemia and splenomegaly. The tendency of Hgb C to aggregate into precipitates is responsible for the characteristic target (actually pseudotarget; see Fig. 11-18, B) morphology of the Hgb C homozygous and Hgb C trait (heterozygous) cells on the peripheral blood smear. Vaso-occlusive phenomena are not associated with this disease, although target cells are formed on the dried peripheral blood smear. However, Hgb C, when paired in a double heterozygous state with Hgb S, results in Hgb SC disease (see Fig. 11-20) and is associated with vaso-occlusive phenomena, although less severe than Hgb SS.
Unstable hemoglobin variants, unlike most hemolytic hemoglobinopathies, rarely have a characteristic morphology, although basophilic stippling and Heinz bodies may be noted on special staining of the peripheral blood (Fig. 11-29). The Heinz bodies represent hemoglobin aggregates that have precipitated intracellularly. RBCs in Heinz body anemia usually are normocytic but may be hypochromic as a result of RBC splenic “pitting” of precipitated hemoglobin. Because the precipitated hemoglobin may be mistaken for reticulum, reticulocyte counts may be spuriously high. Methylene blue staining of RBCs after they have incubated for a few hours can demonstrate the Heinz bodies. Unstable hemoglobinopathies have an autosomal dominant pattern of inheritance, and affected individuals are heterozygotes. A homozygous state would, in most cases, be incompatible with life. Congenital Heinz body hemolytic anemia is an important cause of congenital hemolytic anemia. This condition, although frequently a persistent process in the older infant, has been observed to resolve.
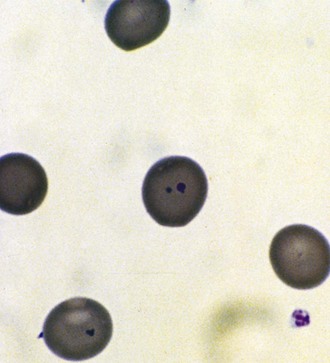
Figure 11-29 Heinz body preparation. This peripheral blood smear of a 2-week-old infant with brisk hemolytic anemia demonstrates a positive Heinz body preparation. The dark-staining material within two of the red blood cells shows the precipitated hemoglobin that one may see in a condition called unstable hemoglobin.
The Coagulation System
Platelets mature from megakaryocytes and have a life span of approximately 7 to 10 days. Platelets adhere to the site of injury by connecting to exposed von Willebrand factor on the vessel basement membrane. Subsequently, fibrinogen binding acts as a stimulus for the aggregation of other platelets. Once platelets have gathered at the site of injury, phospholipids on their surface interact with calcium to enable the hemostatic activity of the coagulation cascade. This process is a complex, interconnected series of reactions between enzymes and cofactors that leads to the deposition of fibrin (Fig. 11-30). The extrinsic pathway is activated when exposed tissue factor is contacted by circulating factor VIIa, causing the initial burst of thrombin production. The intrinsic pathway, through feedback mechanisms triggered by thrombin generation, plays an important role in clot propagation and continued fibrin production. Disorders of platelet plug formation commonly present with mucocutaneous oozing or bleeding, whereas disorders of coagulation factors often present with delayed and deeper tissue bleeding due to the ineffective fibrin deposition.
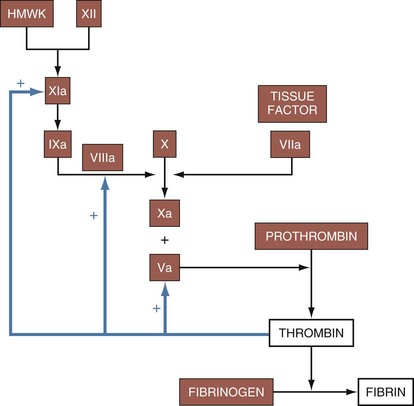
Figure 11-30 Overview of the coagulation system. Red and white boxes represent factors in the clotting cascade. The extrinsic pathway is composed of tissue factor and factor VIIa, whereas the intrinsic pathway features high molecular weight kininogen (HMWK) and factors XII, XIa, IXa, and VIIIa. Both pathways contribute to the conversion of factor X into its activated form (Xa), leading to thrombin generation. Thrombin not only cleaves fibrinogen into fibrin but also upregulates the cascade in several areas to contribute to clot propagation (represented by blue arrows).
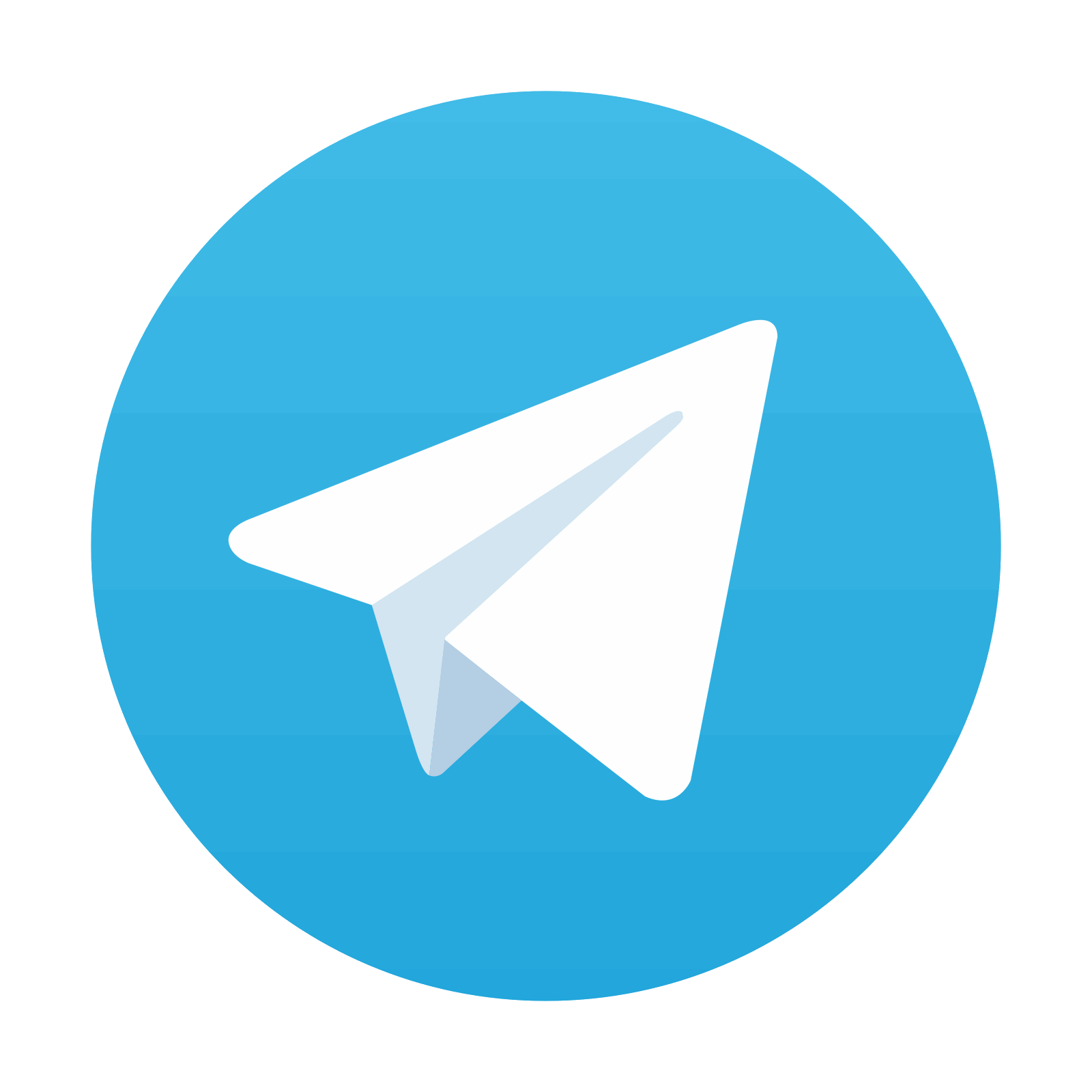
Stay updated, free articles. Join our Telegram channel

Full access? Get Clinical Tree
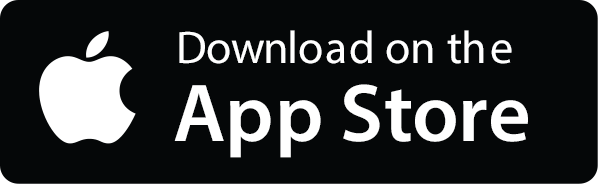
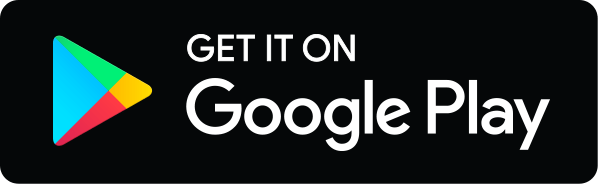