6. Heat Balance*
Vivian D. Brown and Susan Landers
Optimal care of sick newborn and premature infants requires meticulous attention to detail. The consequences of overlooking some details may not be readily apparent, whereas other details may affect the very survival of the neonate. Such was the case late in the nineteenth century when French authorities sought to decrease infant mortality as one way to increase the population and provide the needed manpower to support industrialization and the armies of the expanding French empire. Dr. Stephane Tarnier, Chairman of Obstetrics of the University of Paris, was distressed with the plight of the “weaklings” and applied earlier concepts of incubators to the regular care of premature and sick infants, believing that maintaining thermal stability was a key factor for their survival.
The first incubator for neonates was introduced in 1835 by Von Ruehl in St. Petersburg, Russia, and in 1857, the incubator was described by Denunce as a double-walled box that circulated warmed water within the interspace. 2 The care of newborns was delegated to Madame Henry, Midwife-in-Chief, who oversaw the building of a pavilion specifically for the care of these weakling newborns. 20 This pavilion housed 12 incubators in which fragile newborns were warmed over a hot-water reservoir attached to an external source of heat. These were impressive first steps in attempting to control the fragile heat balance of weak preterm infants and contributed to the reported decreased mortality rate from 66% to 38% in babies with birth weights between 1200 and 2000 g. Dr. Tarnier’s successor, Dr. Budin, continued this important early practice of neonatology, focusing on the home care of these high-risk babies. Alexandre Lion improved the design of incubators and charged spectators a fee to see them in action, which led to a very popular show at the Berlin Exposition of 1896. An associate of Lion, Martin Couney, brought the incubator shows to the United States, where Dr. Joseph DeLee adopted the technology and opened an “incubator station” in 1900 at the Chicago Lying-in Hospital. Nearly all of the large expositions in America hosted “Incubator Baby Side Shows.” These began in 1898 with the Trans-Mississippi Exposition and continued on to the New York World’s Fair in 1939.
Dr. Couney’s display of incubators at Luna Park on Coney Island and at a second park named Dreamland hosted premature babies from New York hospitals that lacked the facilities to care for them. These infants were lined up under heaters in incubators, and they breathed filtered air. At least 8000 babies passed through these incubators, and at least 6000 were saved. Servocontrolled radiant heat in incubators was initially reported by Agate and Silverman in 1963. 2
Today’s radiant warmer is an evolution from the original idea of Agate and Silverman. Radiant energy as the sole source of heat from an overhead panel was described in 1969 by Due and Oliver. Widespread use of the warmer in the delivery room was readily accepted and soon led to its use in the neonatal intensive care unit. The factors that effect heat loss and heat production were elucidated. As intensive care became more readily available, easy accessibility to the infants became increasingly necessary and the open warmer became more readily used. 12
Changes in the radiant warmer have included introduction of incubators that are interchangeable with and convert to radiant warmers. The use of humidification in the incubators has also been improved to allow for varying humidification based on the infant’s gestational age and weight. These new beds allow the caregiver to rotate the mattress 360 degrees for easy patient access and provide an in-bed scale. Recent studies have encouraged the introduction of polyethylene plastic bags and wraps for use with babies born at approximately 30 weeks’ gestation or less. 13 This type of warming is ideal for a preterm infant (at birth and the immediate hours following) awaiting transportation to a tertiary care facility or indeed a baby born in a tertiary care facility. The baby is placed on a warm towel (but not dried) and placed under a radiant warming heating device. The baby (excluding his or her head) is placed fully in the polyethylene bag or is wrapped in the polyethylene sheet. The baby should remain under the radiant warmer as the heat, acting through the covering on the baby’s moist skin, creates a warm thermal environment. Cutting an appropriate-size hole through the covering over the area of insertion can facilitate the introduction of any catheter or cannula. Polyethylene bags for warmth have been adopted by the Neonatal Resuscitation Program (NRP) (see Chapter 4).
NRP guidelines emphasize how hypothermia may reduce the extent of brain injury after hypoxia and that hyperthermia may worsen the extent of brain injury during reperfusion after hypoxic events. The recommended goal is to maintain normothermia for the infant and avoid iatrogenic hyperthermia in resuscitated newborns (see Chapter 26).
Building on these early findings and occasional misguided efforts over the next several decades, 17,18 researchers have gained insight into the physiology of thermoregulation and developed the technology to maintain thermal neutrality in the tiniest and sickest neonates. Although the staffs of modern neonatal intensive care units (NICUs) have the expertise and equipment to avoid the consequences of inadequate thermoregulation, determining the most appropriate ways of attaining the best temperature balance is the subject of ongoing investigation. This chapter discusses the current knowledge of the physiology and pathophysiology of neonatal thermoregulation and techniques used not only to prevent heat loss but also to manage heat balance.
PHYSIOLOGY
Animals that maintain their body temperature within a narrow range through a wide range of environmental temperatures are known as homeotherms. Humans, as homeotherms, maintain a “normal” body temperature by balancing the amount of heat lost from the body with the amount of heat generated from within the body. Our ability to cope with changing thermal environments improves physically and physiologically with age. Eventually we are physically able to move to a different place with a more suitable environment or dress more appropriately when the temperature is uncomfortable.
Babies, especially preterm or small-for-gestational-age (SGA) babies, of course cannot physically respond as older children would, and even their physiologic responses are different and limited. Adults lose some thermoregulatory control during rapid-eye-movement sleep. Because newborns spend much time in active sleep, loss of their ability to compensate for changes in environmental temperatures may be detrimental to their well-being. Recent evidence suggests that thermoregulation is not impaired during active sleep in the neonate, which indicates the developmental importance of both thermoregulation and active sleep in the maturation of newborn infants.
Physiologic responses to a cold environment include metabolic reactions that consume substrate and oxygen and result in heat production. A neutral thermal temperature is the body temperature at which an individual baby’s oxygen consumption is minimized. Thus a minimal amount of the baby’s energy is expended for heat maintenance, and energy is conserved for other basic functions and for growth. Minimal metabolic activity is possible within a narrow range of temperatures, so temperatures that are too high or too low add stress and increase metabolic rate. Extreme deviations from this range overwhelm the thermoregulatory mechanisms, leading to body temperature changes and potentially death.
CAUTION: All studies used to developFigures 6-1and6-2were conducted under specific, controlled environments that may not exist in the clinical setting. The ideal temperature varies with the particular baby and environmental variables such as relative humidity, type of incubator, and clothing used.
![]() |
FIGURE 6-1 (From Klaus M, Fanaroff A: Care of the high-risk neonate, ed 2, Philadelphia, 1979, Saunders.) |
![]() |
FIGURE 6-2 (From Hey EN, Katz G: The optimum thermal environment for naked babies, Arch Dis Child 45:328, 1970.) |
The goal in controlling a neonate’s environment is to minimize energy expended by him or her to maintain a “normal” temperature, thus eliminating thermal stress. This neutral thermal environment is the sum total of factors at which a baby with a normal body temperature has a minimal metabolic rate and therefore minimal oxygen consumption. Both traditional indirect calorimetry and the more accurate and sensitive direct calorimetry are used to study the production and expenditure of heat in newborns. Factors such as ambient air temperature, airflow velocity, relative humidity, and temperature and composition of objects in direct contact with the infant or to which heat may be radiated compose the infant’s thermal environment.
When exposed to a cold environment, a neonate senses the reduced skin surface temperature (using sensors in the skin, primarily the face) and senses core body temperature (using sensors along the spinal cord and in the hypothalamus). Information from these various sensors is processed (probably in the posterior hypothalamus), including average temperature, rate of temperature change, and size of the stimulated area. Cold stress results in the initiation of a series of reactions to increase heat production and decrease heat loss. In adults, the most significant involuntary method of heat production is shivering. Neonates rarely shiver and must rely on nonshivering, or chemical, thermogenesis to produce the needed heat. This process is initiated in the hypothalamus and transmitted through the sympathetic nervous system, leading to the release of norepinephrine at the site of brown fat. Brown fat, found mostly in the nape of the neck, axillae, and between the scapulae of newborns, is a specialized type of fat. It is unique in that it contains thermogenin, which is the key enzyme regulating nonshivering thermogenesis. Norepinephrine causes the release of free fatty acids, which with thermogenesis undergo combustion in the mitochondria of brown fat cells, releasing heat. Lipoprotein lipase also provides further triglyceride substrate for heat production.
Oxygen and glucose also are consumed during nonshivering thermogenesis. Thus an infant who already has low oxygen or glucose levels may become hypoxemic or hypoglycemic if added thermal stress occurs. Preterm babies do not develop sufficient brown fat stores to mount a significant heat production response to compensate for even minimal cold stress. 7When servocontrol is used, the thermistor must not be placed over an area of brown fat, which may directly heat the overlying skin, causing a decrease in servocontroller heat output.
Heat generated within the body is transferred by conduction through tissues along a gradient from warmer to cooler areas such as the skin surface. An initial response to a cold environment is to constrict superficial blood vessels to minimize the transfer of heat from the core to the surface of the body. Superficial vasoconstriction, which gives the skin a mottled appearance in response to cold stimulus, results in a lower skin temperature reading to the thermocontroller and consequently causes an increase in the incubator temperature. The smaller the body size, the less effective vasoconstriction is in conserving heat.
Compared with adults, newborns have a very large surface area to body mass ratio and therefore have a relatively large area exposed to the environment from which heat can be lost. More mature infants may try to minimize their surface area by changing positions to decrease exposed surface area when faced with cold stimulus, but immature infants cannot flex the trunk and extremities effectively. They also have little subcutaneous fat tissue (which acts as insulation) to help prevent heat conduction to the body’s surface, where the heat would be lost.
Heat is transferred from the infant’s body to the environment (i.e., everything in proximity to the baby) along a temperature gradient from warmest to coolest. This transfer of heat occurs by four principal mechanisms: radiation, conduction, evaporation, and convection.Figure 6-3 illustrates these four mechanisms and identifies interventions to minimize their effects.
Much less frequently, a newborn must call on physiologic responses to an environment that is too warm, and these responses are somewhat limited. As skin temperature rises, superficial blood vessels dilate, increasing the transfer of core body temperature to the surface. Increasing the temperature gradient between the skin and the environment increases heat loss from the body. When exposed to elevated environmental temperatures, preterm babies generally cannot generate sweat to eliminate heat by evaporation. Maturing babies develop this eccrine gland function first on the forehead, followed by the chest, upper arms, and more caudal areas.
Thermoregulation requires energy (caloric) expenditure:
• Basal metabolic rate: 50 kcal/kg/day
• Thermoregulation: 10 kcal/kg/day
• Thermic effects of feeding: 8 kcal/kg/day7 (see Chapter 17)
Temperature Management
A neonate’s temperature can be determined by various methods. Deep body (core) temperature may be measured in the rectum or esophagus and on the tympanic membrane. Rectal thermistors are thin, flexible probes that must be inserted at least 5 cm to obtain an accurate reading. Insertion to this depth runs the risk for perforation, because the sigmoid colon makes a right-angle turn approximately 3 cm from the anal opening. Esophageal and tympanic readings are difficult to obtain and usually impractical. Noninvasive infrared thermometry, a rapid and painless method of determining tympanic membrane or axillary temperature in children, is not recommended for use in newborns at this time. Studies have failed to demonstrate an accurate correlation between infrared thermometer readings and axillary or rectal temperature readings in newborn infants. Continuous monitoring of abdominal skin temperature with the newborn lying supine is a noninvasive method that has been reported to show good correlation with rectal temperatures. It is impractical to keep babies in a supine position constantly, so research to find the best practice to monitor and servocontrol infants’ temperatures continues. 12 Correlation with this method and core temperature requires further study, and whether its use with servocontrol is appropriate is yet to be determined, because incubators are programmed to respond to insulated skin temperature, not core temperature.
Because of the risks involved, rectal temperatures should not be taken on a routine basis in neonates. Axillary temperatures are easy to obtain and safely measured using glass, electronic, or disposable thermometers. The tip of the thermometer should be held firmly in the midaxillary area for at least 3 minutes in preterm infants and 5 minutes in term infants. When taken properly, axillary temperatures provide readings as accurate as rectal and core temperature methods. In term infants, axillary temperatures should be maintained at 36.5 ° to 37.5 ° C (97.7 ° to 99.5 ° F). For preterm infants, the normal axillary temperature ranges between 36.3 ° and 36.9 ° C (97.3 ° and 98.4 ° F).2
In critically ill infants, the skin temperature is usually routinely monitored in addition to regular axillary temperature readings. A skin probe is secured to the right upper quadrant of the abdomen. The temperature probe should not be placed under the axilla or any other position except as recommended by the manufacturer. Because an infant responds to cold stress by vasoconstriction, a drop in skin temperature may be the first sign of hypothermia. The core temperature may not fall until the infant can no longer compensate. The axillary temperature may remain normal (or even be elevated) because of proximity to brown fat stores.
ETIOLOGY
The ambient temperature range in which a healthy full-term infant maintains a stable core temperature is narrower than the temperature range in which an adult maintains a normal temperature. When measures are taken to provide a neutral thermal environment for the neonate, excessive heat losses or gains are avoided and heat balance is maintained. Recognition of infants at risk for heat imbalance is essential in the prevention of thermal stress.
Premature infants have a limited ability to control body temperature and are extremely susceptible to hypothermia. Factors that contribute to temperature instability include very thin skin, large surface area relative to body mass, limited substrate for heat production, decreased subcutaneous tissue, and an immature nervous system. These infants often have multiple health problems that necessitate frequent interventions by health care providers with consequent disruption of the infant’s neutral thermal environment.
A premature infant’s very thin skin and larger surface area to body mass ratio allow for increased evaporative heat loss. Term infants can reduce surface area by flexing their extremities onto their trunk, a skill that increases with gestational age. Unable to maintain flexion, a preterm infant lies primarily with extremities extended. Care providers may reduce the surface area by positioning infants in flexion and supporting them with blankets and rolls. The shortened gestation limits lipid supplies, brown fat, and the accumulation of subcutaneous tissue. The immature nervous system delays or mutes the infant’s response to thermal stress.
The premature infant is likely to experience other complications (e.g., respiratory distress, sepsis, intraventricular hemorrhage, hypoglycemia) that may increase basal metabolic rate and oxygen consumption, thus interfering with the ability to maintain thermal stability. Numerous procedures and interventions (e.g., medication administration, placement of intravascular catheters, obtaining vital signs) may impede efforts to maintain a neutral thermal environment. Care providers should routinely check the infant’s temperature before initiating treatments. If the temperature is low, treatment should be delayed until a more normal temperature is obtained. If interventions are prolonged, temperature should be monitored frequently, an external heat source provided, and the intervention stopped if hypothermia occurs.
Late-preterm infants are predisposed to morbidities because of their developmental immaturity (see Chapter 5). Less adipose tissue for insulation, less brown fat for chemical thermogenesis, more heat loss, and a larger ratio of surface area to weight contribute to problems with heat balance in these infants. Morbidity associated with heat balance in the late-preterm infant is 10% compared with 0% for term infants. 3
Low-Birth-Weight Newborns
Low-birth-weight (LBW) (<2500 g) infants can be divided into two groups: the very-low-birth-weight (VLBW) infant (<1500 g) and the extremely-low-birth-weight (ELBW) infant (<1000 g). Preterms in each of these groups have specific needs for thermoregulation. Heated incubators, radiant warmers, and skin-to-skin care are all methods for maintaining the temperature and promoting weight gain of the VLBW infant. Infants weighing more than 1500 g may be weaned to an open crib if all criteria are met. (See Figure 6-5 for weaning criteria.) During the first 12 hours of life, ELBW preterms become hypothermic with procedures such as intubations, chest x-ray examinations, IV line placement and manipulation, suctioning, repositioning, and vital signs. Like the late-preterm and the LBW infant, ELBW infants have even less brown and subcutaneous fat for maintaining body temperature. Their thin skin also contributes to increased insensible water loss.
![]() |
FIGURE 6-5 (Modified from Brown V, Gardner SL: Neonatal thermoregulation: clinical practice tools and policy/procedure/protocol packets, 2008, Nurse’s Professional Development & Practice Association, LLC. Accessed August 5, 2009, from www.npdpa.com.) NPDPA™ |
SGA infants, like preterm infants, have a large surface area relative to body mass and decreased subcutaneous tissue, brown fat, and glycogen stores, all of which contribute to heat imbalance. Decreased placental blood flow frequently contributes to the small size. The relatively large surface area of an SGA infant increases evaporative and radiant heat loss, whereas limited brown fat stores and subcutaneous tissue contribute to a decreased ability to produce and conserve body heat. Some flexion of the extremities may be present because flexion depends on gestational age and not weight. SGA infants have a higher metabolic rate compared with infants at similar weights who are appropriate for gestational age. This is believed to be caused by the larger brain size relative to body weight. Hypoxia in utero may depress the infant’s central nervous system (CNS) and alter the ability to regulate temperature. Increased energy requirements coupled with limited glycogen stores may result in hypoglycemia and limited ability to produce heat. SGA infants may require numerous interventions that disrupt the neutral thermal environment. Care providers should ensure that the infant has a normal and stable temperature before initiation of treatments. If treatments are prolonged, temperature should be monitored frequently, an external heat source provided, and treatments stopped if hypothermia occurs.
Infants with neurologic damage or depression may experience difficulty maintaining a stable temperature. Hypoxia before, during, or after delivery, neurologic defects, and exposure to drugs such as analgesics and anesthetics may depress the infant’s neurologic response to thermal stress. Hypoxia decreases the effect of norepinephrine on nonshivering thermogenesis, the main route of thermal regulation in the newborn infant. Hypoxia also may reduce the oxidative capacity of the mitochondria in brown fat and skeletal muscles, which are involved in thermogenesis. Infants who have experienced hypoxia in utero may have increased norepinephrine concentrations, which result in peripheral vasoconstriction. This may cause a delayed metabolic response to cold stress and delayed vasodilation in response to heat stress.
Neurologic defects that affect the hypothalamus also may interfere with heat balance. The hypothalamus coordinates temperature input from various sensors. Drugs such as analgesics and anesthetics cause CNS depression and reduce the infant’s ability to respond to thermal stress. Neuromuscular blocking agents inhibit the infant’s ability to maintain a flexed position, increasing exposed body surface and heat loss. Care providers must be alert to the effect of drugs on the CNS and the infant’s ability to regulate temperature.
Infants with sepsis may have hypothermia or hyperthermia. In a newborn, an elevated temperature may begin as a response to cold stress, with peripheral vasoconstriction and thermogenesis. Heat production continues as the infant attempts to achieve a higher core body temperature. Exogenous and endogenous pyrogens may enhance thermogenesis.
Initially, an infant with sepsis may feel cool to the touch and may have a low body temperature. As fever progresses, temperature may rise and the infant feels warm to the touch. Infants nursed in servocontrolled incubators may not have an elevated temperature. The lower heater output in response to increasing skin temperature (by manual or servocontrol adjustment) may mask a fever by keeping the baby’s temperature within normal limits. The care provider should be alert to a sudden decreased need for incubator heat support in a previously stable infant.
Hyperthermia may be iatrogenic, caused by inappropriate control of the neonate’s environmental temperature. The most common cause is the inappropriate use of external heat sources. Dehydration also may contribute to hyperthermia. Infants nursed with the use of external heat sources should have their temperatures monitored frequently. Phototherapy, sunlight, and the use of excessive clothing and blankets contribute to overheating. Dehydration may be avoided by early recognition of infants at risk for increased fluid loss. Increased insensible water loss occurs in preterm infants because of increased skin permeability and the use of phototherapy and radiant warmers. Vomiting, diarrhea, gastric suction, and ostomy drainage also increase fluid loss. These infants should receive additional fluids to replace the increased losses (see Chapter 14).
PREVENTION OF HEAT/COLD STRESS
Heat balance is determined by the amount of heat lost to the baby’s environment offset by the amount of heat generated by the body plus the amount of heat supplied from outside sources. Because a smaller, more immature, and sicker baby is less able to regulate body temperature, it is crucial that care providers understand the physical and physiologic principles of heat balance and be able to maintain a neutral thermal environment. Two broad categories of interventions foster thermal neutrality1: blocking avenues of heat loss; and providing external heat and environmental support to maintain temperature within the normal range of 36.5 ° to 37.5 ° C (97.7 ° to 99.5 ° F). 4 The theoretical neutral thermal environment necessary for neonates of 1 and 2 kg at a given age is graphed in Figure 6-2. Newborns of less than 800 g are not adequately addressed in currently available tables but should have a starting environmental temperature setting of 36.5 ° C (97.7 ° F).
Attention to the details of these interventions begins in the delivery room, in which the first step is to adjust the ambient delivery room temperature higher than ordinary operating rooms or patient rooms. The air temperature in newborn care areas should be kept at 23.8 ° to 26.1 ° C (75 ° to 79 ° F), and humidity should be kept at 30% to 60%.2 Warming the room and placing the resuscitation table away from doors or drafts minimize convective heat loss. The newborn’s skin temperature may drop by as much as 0.3 ° C/min, with core temperature dropping more slowly after delivery. At birth, most heat loss results from evaporation of amniotic fluid from the baby’s skin surface. Drying the infant with prewarmed towels and immediately replacing used ones with dry, warm towels minimize evaporative heat loss. Dry towels conduct heat poorly when contacting the neonate’s skin. However, cold examiner hands, stethoscopes, scales, and bare mattresses are good heat conductors and can add significant cold stress if not warmed before coming in contact with the newborn.
Another means of preventing heat loss in very preterm infants in the delivery room is to wrap them in a polyethylene bag or sheet immediately after delivery. Resuscitation then proceeds as usual, and the baby is unwrapped after placement in a warmed incubator. Admission temperatures were higher in the wrapped group and did not drop after unwrapping when the infants were compared with babies who were dried but not wrapped. 21Treating hypothermia in the newborn prevents serious and life-threatening complications. In an attempt to maintain heat balance, the neonate increases cellular metabolism and oxygen consumption, which increases the risk for hypoxia, cardiorespiratory problems, and acidosis. Hypoglycemia is also a risk factor, since the infant must consume more glucose for heat production. Other complications include clotting disorders, neurologic problems, hyperbilirubinemia, and even death if the untreated hypothermia progresses.
Resuscitation should take place on a preheated radiant warmer so that the adverse consequences of hypothermia are avoided. Because a significant amount of heat is lost through the surface area of the head, with its abundant blood supply and the brain’s high heat production, covering the infant’s head with some insulating material conserves heat during transfer to the nursery or NICU and afterward. Stockinet material is relatively ineffective for this purpose and provides poor insulation. The best material is thick, maintains its shape with use, and has a high percentage of air volume trapped in the fibers. Knitted wool caps or Thinsulate material may provide the best results.
There are a variety of ways to maintain thermal neutrality. Accessibility, insensible water loss, servocontrol versus manual control of temperature, and safety are major considerations when determining the method to use for an individual neonate.
Incubators
Incubators provide a controlled, enclosed environment that is heated convectively with warm air. The temperature in an incubator may be servocontrolled to maintain a desired skin temperature or air temperature. As the temperature varies from the desired “set point,” proportional control units gradually increase or decrease heat output to maintain a constant temperature (without the wider temperature fluctuations seen with simple on-off controllers). In setting the servocontrolled incubator to the desired skin temperature, the sensor should be attached to the right upper quadrant of the abdomen with insulated temperature patches. The sensor should not be placed over areas of brown fat deposits, because the higher-than-expected temperature information to the controlling unit will result in a lower-than-desired heat output.
Inadvertent cooling may take place if the sensor is covered with clothes or a blanket or if the baby lies on it. If the sensor becomes disconnected from the skin, unwanted heating may occur because an erroneously low temperature reading causes an unwanted increase in heat output. One also must consider that when an insulated patch is used to cover the thermistor, skin temperature is sensed as being higher than if tape covers the thermistor, resulting in decreased heat output by the warming device. The desired skin temperature used for skin servocontrol is generally 36.0 ° to 36.5 ° C (96.8 ° to 97.7 ° F).15
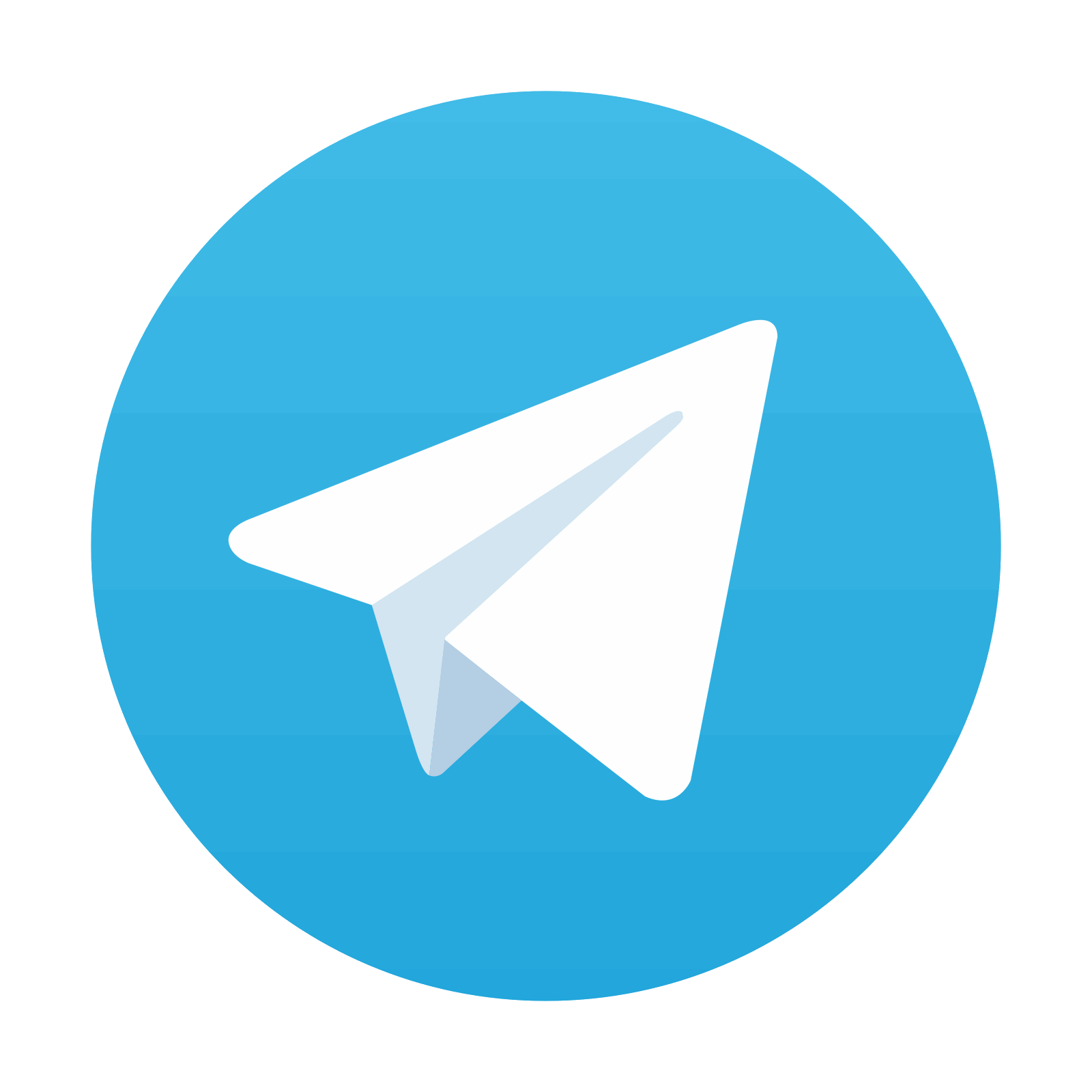
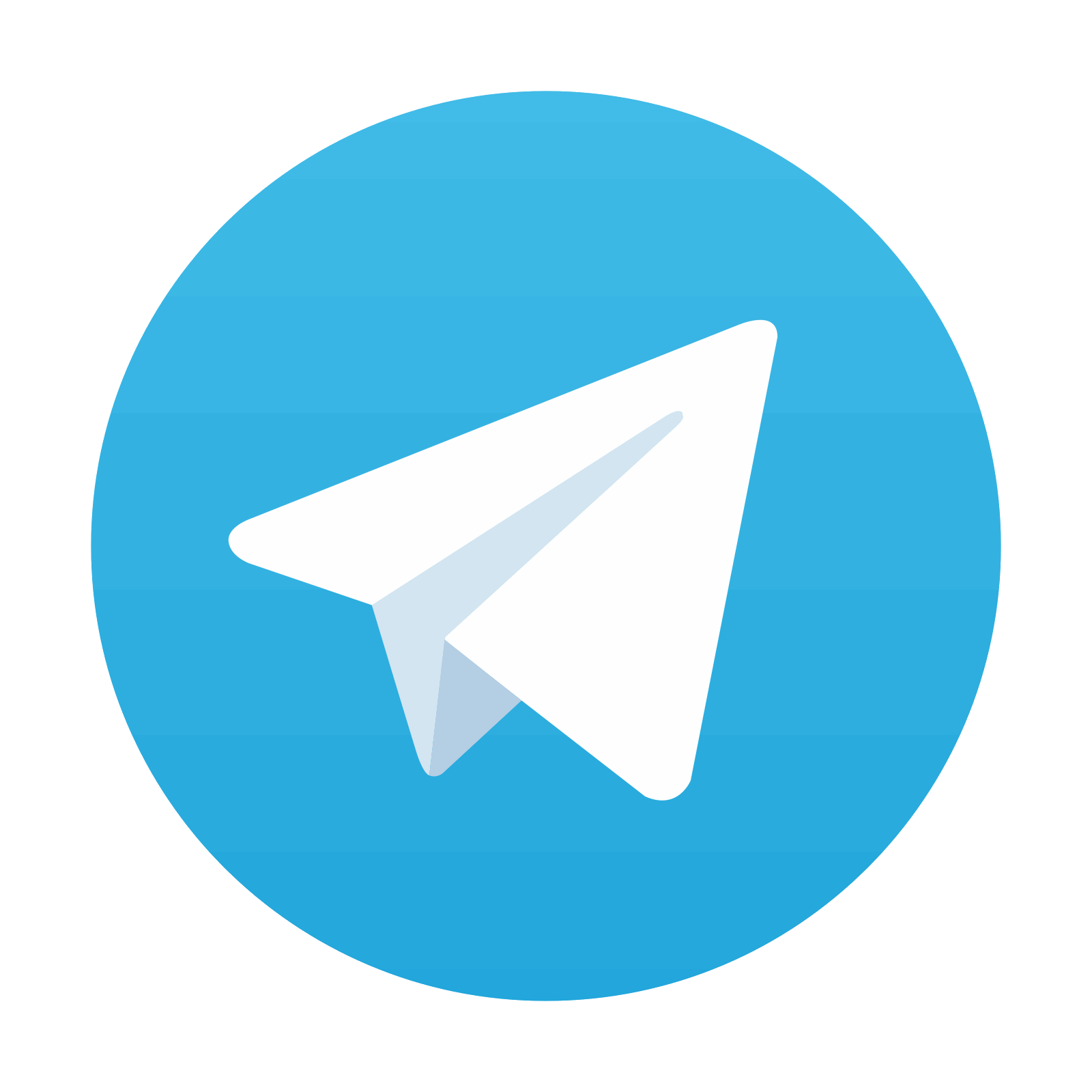
Stay updated, free articles. Join our Telegram channel

Full access? Get Clinical Tree
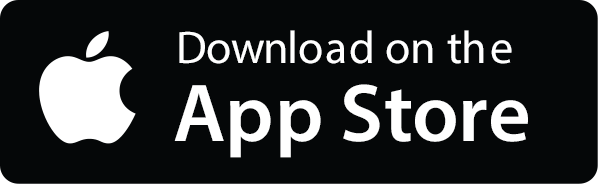
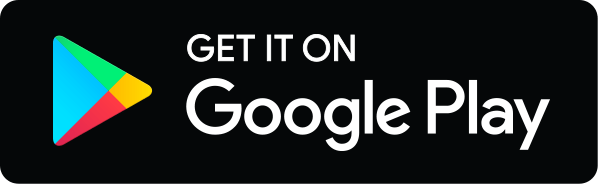
