Fig. 20.1
Germ cell transplantation. Germ cells obtained from a donor testis are transplanted into the recipient testis either directly or after propagation in vitro. To improve donor cell colonization efficiency, the recipient testis should have undergone endogenous germ cell depletion prior to the transplantation by either genetic engineering, irradiation, or cytotoxic drug treatment. The recipient testis gives rise to donor-derived spermatogenesis after germ cell transplantation, which can be used for biological research and fertility preservation both in human medicine and in animals. (a) Donor germ cells are transplanted into the recipient testis through an efferent duct (black arrow) via microinjection. (b) Appearance of a recipient mouse testis after germ cell transplantation. Trypan blue was added to the germ cell suspension before transplantation in order to visualize filling of the seminiferous tubules with the donor cell suspension
Successful GCT has also been reported in non-rodent animal models such as bulls [25], cats [26], dogs [27], sheep [28], monkeys [29, 30], goats [31], and pigs [32], and autologously in human [33]. Allogenic GCT trials were also performed between mouse and various animal species such as rats [19, 34], hamsters [35], rabbits and dogs [36], primates [37], bulls [38], and cats [26]. Transplanted germ cells from rat and hamster undergo complete spermatogenesis in host mouse testis, while germ cells from other animal species result in incomplete spermatogenesis. This is presumably because the mouse somatic cells lack the ability of supporting germ cells from phylogenetically distant species.
Even now, GCT is the only way to conclusively identify the stemness of SSC. Importantly, GCT provides a functional and quantitative assay system of SSC activity and population . Considering the principle that only an SSC has the ability to generate and maintain a spermatogenic colony in the infertile recipient testis, each colony is thought to arise from a single SSC [39, 40]. Therefore, total number of transplanted SSCs can be roughly estimated by counting the number of colonies formed in the recipient testis.
Neospermatogenesis
After transplanting germ cells, the recipient testis undergoes donor-derived spermatogenesis [4]. Elucidating the mechanism of neospermatogenesis originating from the transplanted SSCs is important for the improvement of GCT efficiency and for further analyses of regulatory mechanisms in SSCs. The transplanted SSCs move towards the basal compartment of the seminiferous tubule to colonize within the SSC niche. This movement of SSCs is called “homing ,” and the homing efficiency determines the number of donor-derived SSC colonies in the infertile recipient testis [41]. In order to home into the niche environment, SSCs have to interact with Sertoli cells, migrate from lumen to the basal compartment of seminiferous tubule, and attach to the basal membrane of the seminiferous tubule [41].
Colonization efficiency of transplanted SSCs is assumed to be 5–10% in mouse and even lower in other animal species [41, 42]. This is mainly because of the difficulty of passing through the tight junctional areas between Sertoli cells which is called blood-testis barrier (BTB) , and this low homing efficiency of transplanted SSCs has prevented the clinical applications of GCT. To improve the homing efficiency to overcome this limitation, work has been directed at uncovering the mechanism of SSC homing. It has been shown that in the absence of small G proteins called Rac1, SSCs lack the ability to migrate through the BTB , but retain their ability to reinitiate spermatogenesis in the testis. Rac1 modulates the expression of tight junction-associated proteins such as claudin 3 [43]. Secreted chemotactic factors from the niche environment, such as GDNF and C-X-C chemokine ligand 12 (CXCL12), were also implicated in promoting migration of transplanted SSCs from the tubular lumen to the basal compartment of the seminiferous tubule [44, 45]. Also, the adhesion molecule beta-1 integrin on both SSCs and Sertoli cells plays a critical role in the localization of SSCs to the basal membrane [46].
Most of the factors required for successful homing are also crucial in the maintenance of SSCs in vivo. For example, deficiency of beta-1 integrin, Rac1, and CXCL12 signaling via its receptor C-X-C chemokine receptor type 4 (CXCR4) in SSCs impaired the maintenance of SSCs, leading to loss of the germ cell population [43–47]. These findings underscore the importance of the niche environment on germ cell homing and survival. In mice, this homing process occurs in the first week after transplantation, and subsequently donor-derived spermatogenic colonies expand [41]. Due to the conserved homing mechanism among various animal species, homing of SSCs happens even in xenotransplantation. However, species-specific differences in spermatogenic regulatory mechanism prevent complete spermatogenesis from xenotransplanted SSCs [26, 27, 36–38].
In mice, after SSCs reach to the basal membrane, transplanted SSCs proliferate to generate monolayered colonies in 1 week–1 month posttransplantation. After 1 month of transplantation, colonized SSCs undergo both mitosis and the resulting cells enter meiosis to generate sperm while expanding their colonies in longitudinal axis of the seminiferous tubule [42, 48, 49]. Thus the length of each colony increases with the passage of time, but the number of the donor-derived colonies decreases gradually after 2 months of transplantation [42]. Most of the homing and colonization mechanisms of transplanted SSCs still remain an enigma, but further understanding of these mechanisms will ultimately lead to successful and efficient clinical applications of GCT.
GS Cell and Testicular Tissue Organ Culture
The culture system of germ cells is well established in mouse, and has provided an SSC propagation system and valuable insights to understand basic regulatory mechanism of SSCs. Cultured germ cells were termed germ-line stem (GS) cells, which were shown to include SSCs as a subpopulation by GCT. GS cells are inducible both from neonate and adult mouse [50, 51], even in feeder-free culture conditions [52, 53]. A crucial role of GDNF for maintaining SSCs in culture was established [50, 51] and soluble GDNF family receptor alpha-1 (GFR alpha-1) is known to enhance the trophic effect of GDNF on GS cells [54]. Recent study has shown that FGF2 can maintain and expand the SSC population in vitro without GDNF [10]. Germ cell purification by FACS or MACS sorting and differential attachment of germ cells and somatic cells to tissue culture plates are effective to prevent the contamination with somatic cells and enable stable long-term GS cell culture.
Up to the present, GS cell culture systems were reported in various animal species including rats [54, 55], hamsters [56], rabbits [57], bulls [25], pigs [58, 59], primates [60, 61], and human [62, 63]. However, it has not been achieved to generate fertile haploid cells from GS cell culture in any animal species. Thus it is still required to transplant GS cells into recipient testes as previously described in order to generate fertility-competent sperm.
While it remains challenging to produce complete spermatogenesis from GS cells all in vitro, an organ culture system with liquid-gas interface can generate functional sperm from neonatal mouse testis in vitro, even after testicular tissue cryopreservation [64]. Long-term spermatogenesis in organ culture from neonatal mice is feasible by using microfluidic devices to mimic in vivo condition [65]. However, the efficiency of spermatogenesis from adult testis is low even in mouse [66], requiring further investigation and system improvements before clinical applications in human patients.
Strikingly, both mouse embryonic stem (ES) cells and induced pluripotent stem (iPS) cells can be induced into primordial germ cell-like cells (PGCLCs) which are capable of spermatogenesis and produce functional sperm when transplanted into infertile recipient mouse testis [67, 68]. Although further study is required to elucidate the properties of PGCLCs, this breakthrough expanded the future possibilities of clinical applications. By translating these results to applications, infertile individuals may be able to regain their fertility in the future.
Testicular Tissue Grafting
Testis tissue xenografting is a feasible method to generate complete spermatogenesis from large animal species in a mouse host. In 2002, complete xenogenic spermatogenesis between large animal species and mouse was first reported by xenografting testicular fragments [69]. In this technique, testicular pieces with a diameter of 0.5–1 mm are obtained from donor and embedded under the back skin of immunodeficient adult mice (Fig. 20.2a). The testicular xenografts increased in size and developed to form seminiferous tubules with all stages of spermatogonic cells (Fig. 20.2b, c). The grafts are then excised, minced, and dispersed to isolate sperm that can be used to obtain viable offspring by ICSI (Fig. 20.2d) [70, 71]. After grafting, testicular tissue grows capillaries to construct a functional circulatory connection between the graft and the recipient mouse [72]. The grafted testicular tissue interacts with hypothalamus and pituitary gland, and undergoes continuous steroidogenesis [24]. Testis xenografting was successfully performed in various animal species, including rodents, carnivores, ungulates, and primates [73]. Although the efficiency of spermatogenesis is different for different donor species, testis grafting is considered an applicable technique for a wide range of animal species.


Fig. 20.2
Testicular tissue xenografting . To improve graft development, the recipient mouse is to be castrated before testicular tissue grafting. Testis tissue obtained from the donor (here illustrated for a porcine donor) is fragmented into small pieces (1 mm3; (a)) and subsequently grafted into the subcutaneous tissue under the dorsal skin of the recipient mouse. After a certain period, the grafted testicular tissue develops and increases in size (b) and shows donor-derived spermatogenesis within the tubular structures (c). Sperm obtained from the grafts (d) can generate embryos by intracytoplasmic sperm injection (ICSI) into eggs and these can result in healthy offspring after transplantation to surrogate females
Importantly, not only fresh testis tissue but also refrigerated or cryopreserved testis tissue can develop complete spermatogenesis when grafted into mice [69]. Therefore, cryopreservation of testicular tissue from human childhood patients, valuable livestock, or endangered species is important for fertility preservation. Some studies have also demonstrated that testicular tissue xenografting is useful to study gonadotoxicity of drugs [74] and endocrine disruptors [75].
Human testicular xenografts from infants or prepubertal boys have been revealed to survive and proliferate with structural integrity of seminiferous tubules in mouse for a long period (6 months), even after cryopreservation, and promote spermatogenesis up to pachytene spermatocyte [76, 77]. In the xenografts from adult human, SSCs and spermatogonia occasionally survived, but no spermatocytes were observed and sclerosis of the xenografted tissue progressed in time [78, 79]. Based on these findings, testicular xenografting can be a powerful tool to examine the early period of gametogenesis in human, as well as to preserve germ cell population from infants or prepubertal individuals, rather than adults. Potentially, this model can also be used for fertility recovery of cryptorchid (undescended testes) individuals, considering that a previous study showed that cryptorchid testis tissue recovered spermatogenesis partially in prepubertal human and completely in horse when grafted into immunocompromised mouse [80, 81].
Testis tissue xenografting provides highly valuable options to explore testicular growth, spermatogenic development, endocrine regulation, and toxicity of various substances on spermatogenesis as well as to maintain and propagate male germ cells for the purpose of fertility preservation.
Applications of GCT
GCT technology can be leveraged into applications in various fields. As mentioned before, GCT serves as a functional and quantitative assay system for SSCs. GCT has contributed not only to identify SSCs, but also to uncover biological features of SSC and mechanism of spermatogenic regulation. Transplantation of gene-manipulated germ cells is considered as an especially useful method for such analysis.
Previous studies demonstrated the feasibility of genetic manipulation of cultured germ cells by using viral vectors in rodents, and the genetically modified germ cells generated fertile transgenic sperm upon transplantation [82–85]. The genetic manipulation of GS cells is also feasible in non-rodent animal species such as goat [86], dog [87], pig [88], and bull [89]. In consideration of these results, transplantation of genetically modified GS cells can provide a novel approach to transgenesis and gene editing of non-rodent animal species.
Transgenic large animal models play an important role in biomedical research, agriculture, and human disease modeling and are also used to produce biopharmaceutical proteins. Currently, transgenic large animal models are mainly produced by somatic cell nuclear transfer [90, 91]. However, this method has low efficiency and frequently leads to fetal deformity and neonatal mortality associated with nuclear reprogramming [92, 93]. GCT can provide an alternative to overcome these problems and generate transgenic sperm in significantly shorter time compared to somatic cell nuclear transfer. Gene-manipulated germ cells transplanted into the testis subsequently colonize the recipient testis, undergo spermatogenesis, and continuously generate fertile transgenic sperm, which can be used to generate transgenic embryos by in vitro fertilization (IVF) . Up to the present, production of transgenic sperm through GCT was reported in goat and pig, following viral transduction and nonviral transfection, respectively [31, 88]. These studies have provided the foundation of future practical applications of GCT for animal transgenesis.
GCT can also be applied to fertility preservation . GCT technology is expected to provide a fertility preservation option for the patients of Klinefelter syndrome, cryptorchidism, and childhood cancers. Klinefelter syndrome is a disease that causes progressive loss of germ cells along with sexual maturation [94]. Preservation of their SSCs before puberty may give patients the option to obtain their own children in the future. People with cryptorchidism may obtain fertile sperm through GCT as long as SSCs can be successfully extracted and preserved [80]. Prepubertal boys at the risk of infertility due to cytotoxic treatment for cancer may have the option to preserve their fertility by cryopreserving their SSCs in preparation for future GCT. While cancer itself can be the direct cause of infertility [95], chemotheraphy or radiotherapy as a cancer treatment often affects germ cells and causes infertility, especially in childhood cancer survivors [3]. Considering that semen cryopreservation cannot be applied to prepubertal cancer patients at the risk of infertility who require gonadotoxic therapies, testis biopsy and germ cell cryopreservation for future GCT can be an effective alternative to their fertility preservation [96, 97]. Testicular tissue banking is currently available in some programs such as the Fertility Preservation Program of Pittsburgh (http://www.fertilitypreservationpittsburgh.org).
Discussion
Autologous GCT is a potential approach to restore fertility, especially for childhood cancer survivors who have become infertile due to cytotoxic therapies to treat cancer. However, the safety of this strategy has to be evaluated before translating GCT to the human clinic. First, elimination of potential contamination of donor germ cells with malignant cells is necessary before GCT can be considered a safe option [98]. Magnetic or fluorescence-activated MACS/FACS cell sorting systems have been explored for their usefulness to remove cancer cells from the donor cells [99, 100], though an efficient depletion system for the malignant cells is not sufficiently established yet [101]. Also, due to the current limitation of human germ cell isolation and enrichment in vitro, it is difficult to obtain sufficient numbers of germ cells from the donor to use for transplantation. Therefore, the development of in vitro propagation systems for human germ cells has a high significance for the future application of GCT. To date, concerns about safety have prevented the application of GCT in humans, although the production of functional sperm through GCT has already been shown in primate models [29]. To minimize the risk to reintroduce malignant cells into the patient, testis tissue xenografting is a promising method to preserve fertility, as it allows to obtain functional sperm from the xenografts [69]. However, safety concerns regarding xenotransplantation, such as potential for contamination with endogenous retroviruses carried by the recipient, still need to be addressed [102]. To prepare for the further development and future clinical applications of GCT and testis tissue xenografting, testis tissue banking is suggested for children at risk of infertility.
Genetic manipulation of germ cells followed by generation of functional sperm through GCT has been achieved in several animal models and has been suggested as an option for people with heritable genetic mutations to correct the mutation in germ cells, to produce sperm to father healthy children. While this strategy for genetic modification is useful and feasible in livestock, its application to germ-line gene therapy in humans remains controversial because of the ethical issues and possible risks for the offspring.
References
1.
Krausz C. Male infertility: pathogenesis and clinical diagnosis. Best Pract Res Clin Endocrinol Metab. 2011;25(2):271–85.PubMed
2.
Palermo GD, Neri QV, Takeuchi T, Rosenwaks Z. ICSI: where we have been and whdingere we are going. Semin Reprod Med. 2009;27(2):191–201.PubMed
3.
Meistrich ML. Effects of chemotherapy and radiotherapy on spermatogenesis in humans. Fertil Steril. 2013;100(5):1180–6.PubMed
4.
Brinster RL, Zimmermann JW. Spermatogenesis following male germ-cell transplantation. Proc Natl Acad Sci U S A. 1994;91(24):11298–302.PubMedPubMedCentral
5.
de Rooij DG, Russell LD. All you wanted to know about spermatogonia but were afraid to ask. J Androl. 2000;21(6):776–98.PubMed
6.
Brinster RL, Avarbock MR. Germline transmission of donor haplotype following spermatogonial transplantation. Proc Natl Acad Sci U S A. 1994;91(24):11303–7.PubMedPubMedCentral
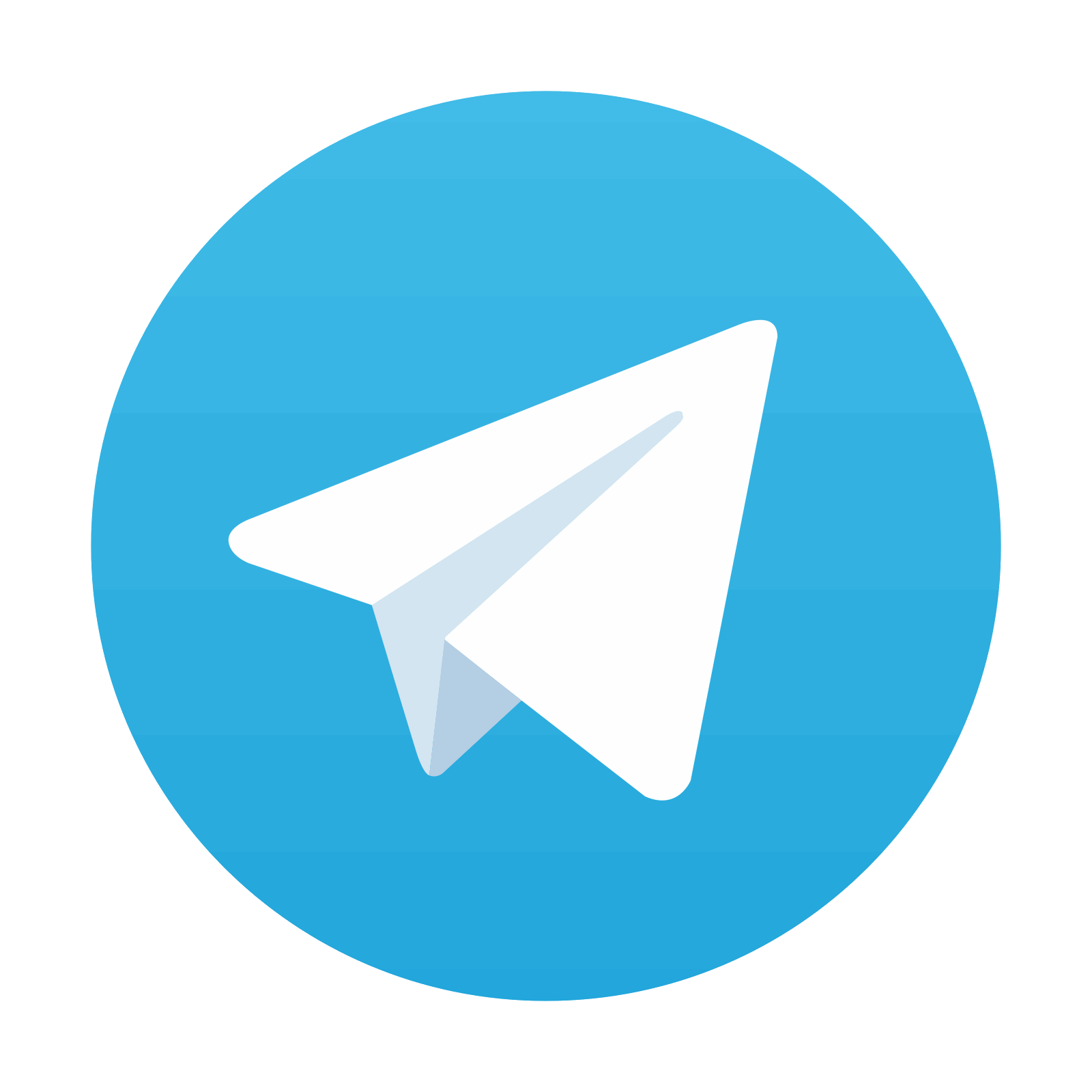
Stay updated, free articles. Join our Telegram channel

Full access? Get Clinical Tree
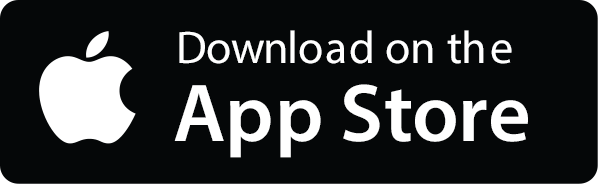
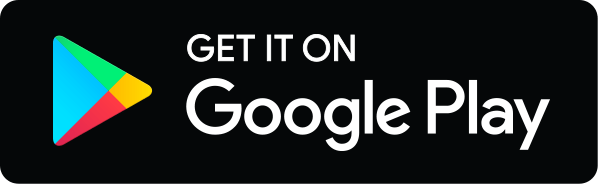