© Springer International Publishing Switzerland 2015
Pier Luigi Meroni (ed.)Antiphospholipid Antibody SyndromeRare Diseases of the Immune System10.1007/978-3-319-11044-8_11. Genetics and Origin of Antiphospholipid Syndrome
(1)
Zabludowicz Center for Autoimmune Diseases, Chaim Sheba Medical Center – Affiliated to Tel Aviv University, Tel Hashomer, 52621, Israel
(2)
Department of Clinical Medicine and Rheumatology, Campus Bio-Medico University, Rome, 00128, Italy
(3)
Zabludowicz Center for Autoimmune Diseases, Chaim Sheba Medical Center – Affiliated to Sackler Faculty of Medicine, Tel Aviv University, Tel Hashomer, 52621, Israel
(4)
Incumbent of the Laura Schwarz-Kipp Chair for Research of Autoimmune Diseases, Sackler Faculty of Medicine, Tel-Aviv University, Tel Hashomer, 52621, Israel
1.1 Introduction
Antiphospholipid syndrome (APS) is a multisystemic autoimmune disorder characterized by vascular thrombosis and/or pregnancy morbidity associated with the concomitant detection of antiphospholipid antibodies (aPL), including lupus anticoagulant (LA), anticardiolipin antibodies (aCL), and anti-β2 glycoprotein I (anti-β2GPI) antibodies [1].
Actually, aPL are a heterogenous group of antibodies that react with a myriad of phospholipids (PLs), PL-protein complexes, and PL-binding proteins. The main antigenic target of these antibodies is recognized to be β2GPI, which along with prothrombin accounts for more than 90 % of the antibody-binding activity in APS patients [2–4].
The genetic basis of aPL [5] and APS [6] has been suggested by several authors according to many clinical evidences, family studies, and experimental models, although the underlying genetic factors predisposing to APS have not been clearly established. Future research in this field may help in delineating also the mechanisms for APS [7].
Similarly, the origin of pathogenic aPL remains to be clearly elucidated. Several environmental factors have been studied in the last decades, with most of the evidence regarding a wide spectrum of infectious agents, which could be responsible for the “second hit” in the APS pathophysiological mechanisms. Molecular mimicry, epitope spreading, and bystander activation are some of the proposed mechanisms through which infectious agents may act in an individual with a procoagulant phenotype, the latter defined as the “first hit” [8].
The focus of this chapter is to gather the most recent scientific evidence with regard to genetics and origin of APS.
1.2 Genetics in APS: Family, Population, and Genome-Wide Association Studies
The human leukocyte antigen (HLA) association with aPL and APS has been studied through various animal models, family and population studies, and more recently, through genome-wide association studies (GWAS). It has emerged that both autoantibody production and disease expression may be influenced by major histocompatibility complex (MHC) genes [8]. In addition, prothrombotic genetic markers may modify the disease expression in APS patients especially when associated with acquired factors such as aPL [9].
APL are a heterogeneous group of antibodies with many different antigenic targets. Today we know that only a limited number of aPL are pathogenic, being induced in most of the cases by certain viral or bacterial products and determining a break in tolerance because of sequence similarities with host antigens [10, 11].
Nevertheless, nowadays it is also clear that a favorable genetic background is essential for the subsequent development of APS, similarly to other autoimmune diseases.
In 1966, Harvey and Shulman [12] described the first family with persistent false-positive tests for syphilis detected in their members, in whom a full-blown autoimmune disease developed years later. In the following decades, several studies described families with high incidence of primary APS associated with LA, aCL, and other autoantibodies [6, 13, 14].
A link with HLA haplotypes has been reported in different family studies on APS: the paternal haplotypes A30, Cw3, B60, DR4, DRw53, and DQw3 have been shown in association with aCL in all the members of an English Canadian family, both in asymptomatic individuals and in those affected with APS secondary to systemic lupus erythematosus (SLE) and autoimmune thyroid disease. In addition, the presence of LA has been demonstrated in family members carrying DR4 and DR7 [15, 16].
A dominant or codominant model for inheritance of the disease by segregation analysis was suggested in a 1998 study considering seven families with a high incidence of primary APS, among which 30 out of 101 members met the diagnostic criteria. However, the study failed to find a linkage to HLA nor to other candidate genes including β2-GPI and Fas [17].
Apart from family studies, association of HLA and APS has been demonstrated in cohorts of patients with primary APS, in which DRB1*04, DR7, DQB1*0301/4, DQB1*0604/5/6/7/8/9, DQA1*0102, and DQA1*0301/2 were found with increased frequency [18–20]. The analysis of a large series of European patients with SLE by Galeazzi et al. [21] has found an association of aCL with HLA-DRB1*04, −DRB1*07, DQA1*0201, DQA1*0301, DQB1*0301, DQB1*0302, and DRB3*0301, while anti-β2GPI were positively associated with DQB1*0302.
Furthermore, the detection of anti-β2GPI was strongly associated with DRB1*1302 and DQB1*0604/0605 haplotypes in African American and in white British patients with primary APS [18, 22], while in Caucasian and Mexican Americans, DQB1*0302 was strongly correlated with anti-β2GPI [18, 22, 23].
Prothrombotic genetic factors have been found playing a role in modifying the disease expression in APS, namely, factor V Leiden mutation; antithrombin III, protein C, and protein S deficiencies; and prothrombin mutations [24]. Subjects with APS carrying factor V Leiden mutation showed an increased incidence of thrombosis in comparison to those without the mutation. On the other hand, other studies comparing the rates of thrombosis in APS patients with factor V Leiden mutation and in the general population (in which the frequency of factor V Leiden mutation ranges from 1 to 15 % for Caucasians) failed to detect a strong influence in APS population on the development of the thrombotic event [24–26]. Moreover, there have been reports of increased risk of thrombosis in APS in case of protein C and S deficiency, as well as in case of association with G20210A mutation [27].
Tissue factor pathway inhibitor [28], type I plasminogen activator inhibitor [29], annexin A5 [30], and p-selectin glycoprotein ligand 21 [31] may potentially positively influence the risk of thrombosis in APS patients.
Recently, Pierangeli et al. [32] showed the in vivo pathogenic role of toll-like receptor 4 (TLR-4) in APS by studying the thrombogenic aPL activity in lipopolysaccharide (LPS) non-responsive (LPS −/−) mice and the association between tlr4 gene polymorphisms and APS in patients affected. The authors found significantly smaller thrombi and fewer white blood cells (WBC) count adhering to endothelial cells in LPS −/− mice displaying a tlr4 spontaneous mutation, when immunized with IgG from patient with APS, suggesting a potential role of TLR-4 in the interaction between aPL and endothelial cells in vivo.
Genome-wide association studies (GWAS) are a relatively new search method based on the study of several single nucleotide polymorphisms (SNPs) in order to identify those occurring more frequently in people affected with a certain disease. This type of study is generally used to pinpoint genes that may contribute to a subject’s risk of developing the disease.
Before the GWAS approach, SNPs analyses had been performed to investigate the genetic association between primary APS and SLE, given their clinical and serological similarities.
In 2009, 10 SNPs previously shown to be associated with SLE have been genotyped in a cohort of Italian APS patients [53]. The rationale of the study was to investigate whether the analysis of SLE susceptibility genes could partially provide an explanation for the small percentage of primary APS patients developing a full-blown SLE over the years, despite the similarities between the two conditions.
The study showed a very strong association with STAT4 and BLK SNPs; conversely, no association was detected with BANK1 [53]. These results were further confirmed when the authors extended their investigation through an additional group of APS patients [54].
Interestingly, in this further study a stronger association with one SNP of IRF5 (rs2070197), which is a tag for risk haplotype in SLE, was found in the additional group [54]. On the other hand, the lack of association with rs2004640, the strongest IRF5 SNP associated with SLE, was also confirmed. These last findings underlined that the differences in the genetic effects of IRF5 between APS and SLE may also account for some of their different clinical expressions.
A genome-wide association study recently performed in an effort to detect loci/genes for aCL, anti-β2GPI, and LA in a cohort of women of European ancestry affected with SLE and carriers of aPL identified a number of suggestive novel loci for aPL, including genes not located near or at the MHC locus [7].
Although the study did not identify loci meeting the conservative threshold of genome-wide significance, it has suggested the potential role in APS of other genes involved in the immune response, like SESTD1 and TANK, which play a role in binding PL species and in type I interferon production, respectively [7]. Undoubtedly, GWAS will contribute to open the way through new directions in the comprehension of APS genetics in the next future.
1.2.1 Genetic Animal Studies
Despite the fact that the first animal models on APS date back to 1980s [33, 34] – before the definition of APS – after three decades animal studies assessing the genetic basis for the development of this syndrome remain few.
The role of certain class of haplotypes in the production of pathogenic aPL came first from a study of Papalardo et al. [35] who elegantly showed the influence of MHC-II and the expression of DQ6, DQ8, and DR4 on the production of pathogenic aPL in mice. MHC-II deficient (−/−), DQ6, DQ8, and DR4 mice, MHC-II-deficient-transgenic mice, or their corresponding wild type (WT) were immunized with human β2GPI or with control protein ovalbumin. WT mice immunized with β2GPI produced significantly higher titers of aCL and anti-β2GPI when compared to controls, and this finding correlated with enhanced thrombus formation and tissue factor activity. MHC (−/−) mice immunized with β2GPI did not produce aCL or anti-β2GPI; mean thrombus sizes and tissue factor activities were significantly diminished, when compared to WT animals. Finally, the pathogenicity of aPL was fully reestablished in MHC (−/−) mice expressing DR4 and DQ8 haplotypes and partially restored in those expressing human DQ6 [35].
In 1992, Hashimoto et al. [36] in their model of APS demonstrated the spontaneous production of IgG aCL antibodies exhibiting the β2GPI-dependent binding to cardiolipin in NZW x BXSB F1 (W/B F1) male mice. Subsequent studies showed a preferential usage of certain VH and VK genes for the production of pathogenic aCL, while random V gene combinations were used for the production of nonpathogenic aCL, suggesting the possible antigen-driven mechanisms.
The search for triggering antigens was proved only in 1992, when Gharavi et al. [37] immunized NIH/Swiss mice with heterologous β2GPI rather than pure PLs, obtaining high-titer production of pathogenic aPL. These results were pivotal to orient research through the concept that the formation of immunogenic complexes against which aPL may be produced may occur when foreign PL-binding proteins with structures similar to β2-GPI bind to self PLs in vivo [8].
Several efforts have then been made in inducing high-titer production of pathogenic aPL in animal models, mainly through the immunization with peptides from microorganisms with functional and sequence similarity to that of the PL-binding site of β2GPI. These studies also contributed to elucidate the origin of pathogenic aPL, as resumed in the following paragraph.
1.3 Infections and Innate Immunity Mechanisms
Apart from the complex aspects of the genetics of APS, the ontogeny itself of the pathogenic aPL remains poorly understood.
As underlined in the introduction to this chapter, despite the presence of an induced procoagulant phenotype which may be genetically determined and finally defines the so-called first hit, an additional inciting factor, namely, “second hit,” is necessary for the clinical manifestations of the disease [8].
Currently, infections are considered one of the most relevant environmental factors responsible for both aPL production and APS development.
The first infection recognized to be linked to aPL production was syphilis, although it was first hypothesized that syphilis-linked aPL were nonpathogenic [38–40].
In the following decades, several reports then showed a link between different types of infections and the development of aPL, as well as clinical manifestations of APS. Namely, human immunodeficiency virus (HIV), human T-cell lymphoma/leukemia virus (HTLV), hepatitis B and C virus, varicella-zoster virus (VZV), parvovirus B19, and cytomegalovirus (CMV) have been associated with the production of aPL and the development of clinical manifestations of APS.
Later on, it was clearly postulated that incidental exposure of genetically susceptible individuals to certain infectious agents that bear β2GPI-like structures can induce a break in tolerance to this antigen, thus facilitating the production of pathogenic aPL [8]. In this regard, molecular mimicry has been proposed as one of the pivotal mechanisms for the production of pathogenic aPL, because of the breakdown in the normal mechanisms of peripheral tolerance.
Great part of the efforts in inducing high-titer production of pathogenic aPL in animal models have been made through the immunization with peptides from microorganisms with functional and sequence similarity to that of the PL-binding site of β2GPI [41].
Greater degrees of PL-binding were shown by the peptides TADL from adenovirus (AdV), TIFI and VITT from cytomegalovirus (CMV), and SGDF from Bacillus subtilis, all of them inducing both high-titer aPL and anti-β2GPI production in mice. The pathogenicity of the antibodies induced in TIFI-immunized mice was then confirmed in subsequent experiments in vitro and in vivo [41–43].
Blank et al. [44, 45] added further evidence for a plausible role of molecular mimicry for the infectious origin of aPL: a β2GPI-related hexapeptide, TLRVYK, was identified by anti-β2GPI monoclonal antibodies originated from a patient with recurrent fetal loss on APS peptide background and peptide phage-displayed library. High titers of aCL β2GPI-dependent antibodies were produced through naïve mice immunization with 7 pathogens out of 24 showing high homology to TLRVYK. When purified anti-TLRVYK antibodies were passively transferred to naïve female mice, clinical manifestations of experimental APS were caused only by the autoantibodies originated from Haemophilus influenzae, Neisseria gonorrhoeae, or tetanus toxoid immunized mice, with high affinity to β2GPI.
The use of three synthetic peptides (namely, “A,” NTLKTPRVGGC; “B,” KDKATFGCHDGC; “C,” CATLRVYKGG) derived from a peptide phage-displayed library and reacting specifically with the anti-β2GPI monoclonal antibodies (mAbs) ILA-1, ILA-3, and H-3 has been shown to neutralize the pathogenic functional activity of anti-β2GPI mAbs in vitro [44]. In vivo infusion of each of the anti-β2GPI mAbs into BALB/c mice, followed by administration of the corresponding specific peptides, was able to prevent the peptide-treated mice from developing experimental APS [44].
Moreover, the synthetic peptide named peptide “A” NTLKTPRVGGC that shares similarity with common bacterial antigens prevented induction of thrombosis ex vivo in an aPL-mediated thrombosis [46]. Peptide “A” is also found in region I/II of β2GPI. A scrambled form of peptide “A” (scGTKGCPNVRLT) was used as a control and had no effect.
Evidence that LPS primes the endothelium of rat mesenteric vessels has been provided for anti-β2GPI-mediated thrombosis [55].
Additionally, treatment of mice with intraperitoneal injection of LPS following the injection of cyanine 5.5-labeled β2GPI has been shown to promote protein localization on gut and brain endothelial cells associated with IgG, C1q, and C9 deposition in immunized mice [56].
In this regard, a critical role for complement in inducing aPL-mediated blood clots and fetal resorption has been found first by using aPL of undefined specificity [55] and, afterward, by using specific antibodies to β2GPI [56]. Agostinis et al. [57] recently proposed a novel therapeutic approach based on the use of a high-affinity human monoclonal antibody to β2GPI that competes with the APS patients’ antibodies for binding to β2GPI and inhibits their ability to induce blood clots and fetal loss by determining the failure of complement activation [57].
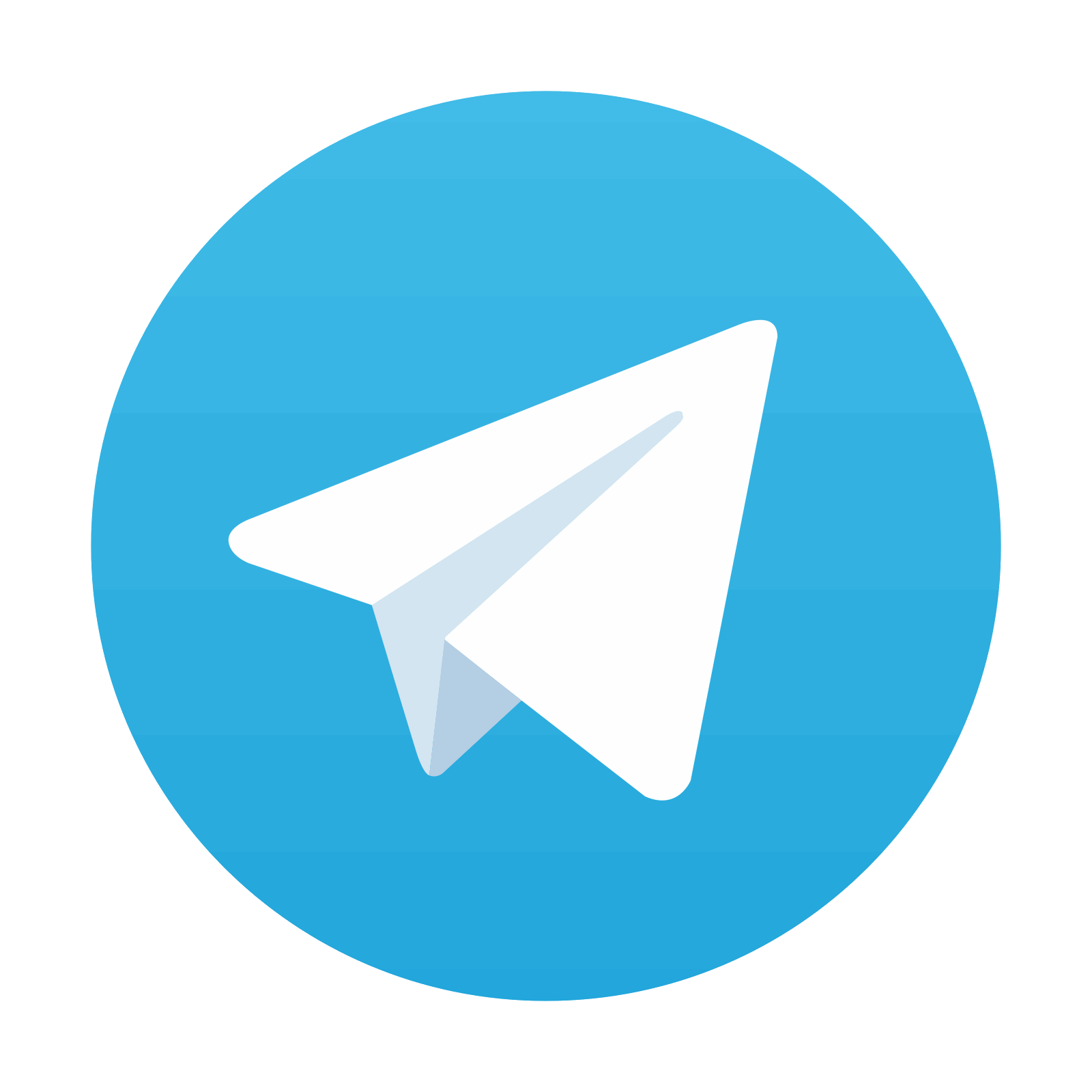
Stay updated, free articles. Join our Telegram channel

Full access? Get Clinical Tree
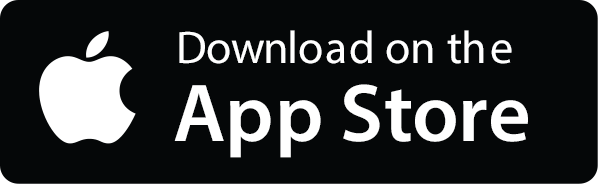
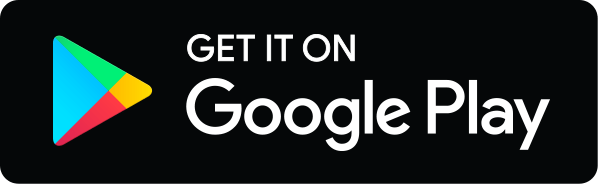