, Arndt Borkhardt2, Bobby Gaspar3, Eleonora Gambineri4 and Stephan Ehl5
(1)
Department of Pediatric Hematology and Oncology, Center of Pediatrics, Freiburg, Germany
(2)
Pediatric Oncology, Hematology and Clinical Immunology, Heinrich Heine University Medical Center, Düsseldorf, Germany
(3)
ICH Infect, Imm, Infla. & Physio Med UCL GOS Institute of Child Health Faculty of Pop Health Sciences, London NHS Trust, London, UK
(4)
“Nuerofarba” Department, Anna Meyer Chidlren’s Hospital, Haematology Oncology Department, BMT Unit, University of Florence, Florence, Italy
(5)
Center for Chronic Immunodeficiency, University Hospital Freiburg, Freiburg, Germany
Keywords
Immune dysregulationImmune homeostasisAutoimmunityAutoinflammationBenign LymphoproliferationHemophagocytic lymphohistiocytosis5.1 Introduction
The primary role of the immune system is defense against infection. Antimicrobial immune responses are highly dynamic processes that involve rapid expansion and contraction of immune cell populations, targeted exertion of highly potent effector functions and secretion of soluble mediators that have antimicrobial properties and influence cell functions and interactions. To maintain homeostasis, both innate and adaptive immune responses require tight regulation. Exaggerated inflammatory responses can be the consequence of uncontrolled activation of the immune system and failure to control immune responses against host antigens causes autoimmunity. There are many checkpoints that help to maintain homeostasis in the immune system involving a variety of cells and mediators. It is therefore not surprising that genetic deficiencies in many immunologically relevant molecules can lead to immune dysregulation in addition to but also in the absence of susceptibility to infection.
Failure to regulate immune responses may lead to various clinical manifestations including (benign) lymphoproliferation, febrile inflammatory responses and autoimmunity. In many cases infections trigger these aberrant responses. In some circumstances, failure to appropriately control pathogens contributes to their maintenance, but in others, no exogenous stimulus can be identified. The molecular and cellular mechanisms responsible for immune dysregulation vary in different forms of primary immunodeficiencies. In many diseases, several mechanisms are involved. Immunodeficiencies associated with immune dysregulation include humoral deficiencies, T cell deficiencies, phagocyte defects and complement deficiencies. These diseases are discussed in the respective sections of this book. This chapter describes several immunodeficiency syndromes that predominantly manifest with immune dysregulation. This includes the familial hemophagocytic syndromes, the closely related immunodeficiencies with hypopigmentation, X-linked lymphoproliferative disease, autoimmune lymphoproliferative syndrome, APECED and IPEX syndrome.
Hypopigmentation or even albinism combined with a variable degree of immunodeficiency is a characteristic hallmark of Chediak Higashi syndrome (CHS), Griscelli syndrome (GS) type II, and Hermansky-Pudlack syndrome (HPS) type II. In addition, mutations in the endosomal p14 adaptor protein cause a complex B-and T-cell immunodeficiency with neutropenia, short stature (usually not seen in any of the aforementioned syndromes) and partial albinism. Skin, hair and the iris may be affected to variable degrees, this predominant clinical appearance is usually seen at birth. Thus, oculocutaneous hypopigmentation may pave the way for early diagnosis of a profound immunodeficiency. The common pathophysiological cause for the astonishing variety of clinical symptoms is severe perturbation of lysosomal and endosomal pathways. On the molecular level, the various steps of biogenesis, transport, distribution and segregation of lysosomes are not yet understood in detail. However, given the complex spatiotemporal control of secretory lysosomes, particularly in melanocytes and immune cells, it becomes understandable why the clinical phenotype may differ widely.
Many but not all of the diseases summarized in this chapter are collectively named “inherited hemaophagocytic lymphohistiocytosis” (HLH), the fatal immune dysregulation resulting in uncontrolled lymphocyte and macrophage activation, hypercytokinemia, cell infiltration and severe organ damage [94].
Another subject of this chapter is primary immunodeficiencies that lead to a particular susceptibility to EBV-triggered HLH, pronounced lymphoproliferation, dysgammaglobulinemia and (aside from XIAP deficiency) lymphoma development of both categories, Hodgkin’s lymphoma and Non-Hodgkin’s lymphoma. Patients with EBV-induced lymphoproliferative syndromes may present with an X-linked (XLP1, XLP2, MAGT1) or autosomal-recessive mode of inheritance (ITK, CD27). (See Table 1.4 and Fig. 1.11 for updated classification of genetic disorders of immune regulation)
5.2 Familial Hemophagocytic Lymphohistiocytosis
(Perforin deficiency, UNC13D deficiency, Syntaxin 11 deficiency, STXBP2 deficiency)
5.2.1 Definition
Familial hemophagocytic lymphohistiocytosis (FHL) is a group of genetically determined, life-threatening diseases caused by the uncontrolled proliferation of activated lymphocytes and histiocytes secreting high amounts of inflammatory cytokines [46, 59, 60]. The symptoms were first described in 1952 and include prolonged fever, hepatosplenomegaly, pancytopenia and neurological symptoms [56]. Currently there are 5 known forms of FHL (FHL1-5), for four of which the causative genes have been identified: FHL-2 (OMIM*603553) is caused by mutations in the gene encoding perforin (PRF1; OMIM*170280) [192, 193], FHL-3 (OMIM*608898) is due to mutations in the gene encoding MUNC 13-4 (UNC13D; OMIM*608897) [57], FHL-4 (OMIM*603552) is caused by mutations in the gene encoding syntaxin 11 (STX11; OMIM*605014) [229], and FHL5 (OMIM*613101) as result of mutations in the gene encoding STXBP2 (OMIM*601717) encoding the protein munc 18-2 [37, 228]. All of these proteins are involved in cellular cytotoxicity mediated by NK cells and T cells [64]. FHL-1 has been linked to chromosome 9q21.3-22; however, its genetic basis is still unknown [141]. In addition, there are further familial forms of the disease whose genetic basis remains to be elucidated.
5.2.2 Etiology
Contact-dependent cellular cytotoxicity by NK cells and CD8+ cytotoxic T cells (CTL) is one of the key effector mechanisms of the immune system against intracellular pathogens such as viruses and intracellular bacteria [100]. Cellular cytotoxicity is mediated by cytotoxic granules in the cytoplasm of NK cells and CTL containing perforin, granzymes and other components. After target cell recognition and formation of an appropriate contact area between effector and target cell (the immunological synapse), granules migrate to the site of cell contact, fuse with the plasma membrane and their contents are secreted into the intracellular space. Perforin and granzymes then cooperate to mediate rapid apoptosis of the target cell (Fig. 5.1) [194].


Fig. 5.1
Pathogenesis of cytotoxicity defects. HPS Hermansky-Pudlak syndrome, FHL Familial hemophagocytic lymphohistiocytosis, CHS Chediak-Higashi syndrome, GS Griscelli syndrome (Adapted with permission from [45])
Perforin, MUNC13-4, Syntaxin 11 and munc 18-2 are all expressed in NK cells and CTL. Perforin is a pore-forming protein that can insert into the lipid bilayer of target cell membranes causing cell death by osmotic lysis and allowing entry of apoptosis-inducing granzymes [23]. MUNC 13-4 is involved in vesicle priming and MUNC 13-4 deficiency results in defective exocytosis despite polarization of lytic granules and docking with the plasma membrane [135]. Syntaxin 11 is also expressed in APC and an impaired interaction between CTL and APC may contribute to FHL-4 [229]. However, the association of syntaxin 11 with other lysosomal proteins and the recent description of impaired CTL and NK cell degranulation in patients with syntaxin 11 deficiency suggests that it is also important for granule exocytosis [31]. Munc 18-2 is important for controlling intracellular granule/membrane trafficking and exocytosis but not only in effector cell populations [228], but also in other cell types including neutrophils [225] and platelets [1].
In the context of its antimicrobial function, perforin-dependent cytotoxicity also plays an important role in the maintenance of T cell homeostasis [44]. During infections, pathogen-specific T cells undergo a massive expansion and activate their direct and indirect antimicrobial effector pathways including cytotoxicity and release of inflammatory cytokines such as interferon-gamma. These pathways are also used by NK cells and lead to control of pathogen replication in infected tissues, but also to the elimination of antigen-presenting cells (APC). Both of these processes lead to a reduction in the level of antigenic stimulation of T cells. As a consequence, most of the effector T cells die leaving a pool of memory T cells that can mediate recall responses on further exposure to antigen. In the absence of perforin-dependent cytotoxicity, this “negative feed-back loop” is ineffective [45, 129]. Prolonged stimulation by APC and impaired pathogen control leads to uncontrolled expansion and persistence of the activated CTL.
Uncontrolled secretion of cytokines by activated CTL and NK cells leads to the hyperinflammatory state characteristic of hemophagocytic syndrome (HLH). Experiments with perforin-deficient mice have identified interferon-gamma (IFN-γ) as a key cytokine involved [99]. IFN-γ is toxic to hematopoietic cells, which contributes to the cytopenia of HLH [18]. It is also a crucial activator of macrophages and tissue infiltration by macrophages with increased phagocytic activity are key features of HLH. This includes phagocytosis of blood cells in bone marrow and other infiltrated organs such as the liver, spleen or the brain, the demonstration of which is relevant for the diagnosis of the disease [61].
5.2.3 Clinical Manifestations
In about 85 % of patients with FHL, the disease manifests within the first year, in 70 % before 6 months of life [95, 96]. A short period of absence of symptoms and normal development after birth is typical. Although the full picture of HLH is rather characteristic, the initial clinical presentation of the disease is highly variable. In most patients, high fever unresponsive to antibiotic therapy, often undulating, in combination with pallor, vomiting and weight loss, are the first signs of the disease. Hepatosplenomegaly is usually pronounced and progressive, lymphadenopathy can only be observed in about 30 % of patients. Jaundice and edema, purpura and bleeding and non-specific skin rashes may also be present. Neurological symptoms can manifest at the beginning of the disease, but more commonly develop later during disease progression. Typical symptoms and signs include irritability, bulging fontanelle, hyper- or hypotonia, seizures and apathy or coma [79]. These symptoms and signs of HLH may be progressive leading to a lethal outcome if untreated, or may be remittent occurring in several bouts that ultimately lead to a lethal episode. Although a milder course of the disease with recurrent exacerbations and remissions has been observed in some patients with syntaxin-11 deficiency [169], clinical criteria do usually not allow to differentiate between the different genetic variants of the disease. Late-onset forms of FHL have been described, in particular in patients with missense mutations in perforin. These patients manifested late into adulthood and frequently showed atypical presentations including predominantly neurological disease [58] or aplastic anemia [188].
5.2.4 Diagnosis
Due to the non-specific symptoms and signs, the diagnosis of FHL is difficult, in particular in patients with an incomplete, late-onset manifestation of the disease (Table 5.1). The two important challenges are to diagnose the hemophagocytic syndrome and to verify a genetically determined form of the disease. Typical laboratory findings of HLH include anemia, thrombocytopenia and, to a lesser extent, leukopenia. Clinical chemistry reveals signs of liver dysfunction including hypertriglyceridemia, hyperbilirubinemia, elevated transaminases, highly elevated ferritin (>500 ng/mL), hyponatremia and hypoproteinemia [95, 96]. In addition, coagulation abnormalities are common, in particular hypofibrinogenemia. Analysis of the cerebrospinal fluid frequently shows mononuclear pleocytosis and increased protein, but may also be normal despite the presence of significant MRI changes such as hyperdense areas, atrophy or brain edema [79].
Clinical criteria | Fever |
Splenomegaly | |
Laboratory criteria | Cytopenia ≥2 lineages |
Hypertriglyceridemia ± Hypofibrinogenemia | |
Histopathologic criteria | Hemophagocytosis in bone marrow, spleen or lymph node |
New Criteria | Impaired NK cell function |
Ferritin >500 μg/L | |
sIL2R >2400 u/mL |
Immunological findings include markedly decreased cytotoxic activity by NK cells and increased levels of activated CD8+ T cells. High levels of several cytokines including TNF-α, IFN-γ, IL-1 and IL-6 can be demonstrated as well as high levels of soluble CD8 or soluble CD25, reflecting the massive T cell, NK cell and macrophage activation [83]. The major histopathological finding is the infiltration of various organs by activated CTL and macrophages. Hemophagocytosis of erythrocytes and leukocytes is frequently observed, but may be absent (Fig. 5.2). Most organs can be infiltrated, but most frequently the spleen, liver, lymph nodes, bone marrow and CNS.


Fig. 5.2
Bone marrow aspirate smear from a HLH-patient showing a macrophage engulfing a granulocyte and a red cell precursor (hemophagocytosis)
Diagnostic guidelines for the diagnosis of HLH have been established and may help in the differential diagnosis [82, 84]. Five of the following 8 criteria must be fulfilled:
Fever
Splenomegaly
Cytopenia ≥ 2 lineages (Hb < 9 mg/l, Platelets < 100,000/ul, Neutrophils < 1000/ul)
Hypertriglyceridemia and/or Hypofibrinogenemia
Hemophagocytosis in bone marrow, spleen, lymph nodes or CSF
Impaired NK cell function
Ferritin > 500 ng/mL
sCD25 > 2400 U/mL
In parallel to the diagnostic evaluation for HLH, the question must be addressed, whether this is a genetic or (more frequently) a secondary form of the disease. A positive family history and parental consanguinity and an early age at manifestation may suggest a familial form. However, secondary forms due to infections, hematopoietic malignancies or autoimmune disease may also manifest in the first year of life [95]. Since infections also contribute to the manifestation of HLH in genetic cases, a careful microbiological work-up is required [50]. This includes blood and CSF cultures, diagnostic evaluation for viral infections (EBV in particular, but also CMV, HIV, adenovirus, enterovirus, parvovirus or HHV-6), fungal infections (aspergillus), bacterial or parasitic infections (congenital lues, military tuberculosis, leishmaniosis, malaria, and brucellosis). Visceral leishmaniosis is particularly difficult to diagnose and may require repeated very careful analysis of bone marrow smears in addition to serological tests. It is not a rare cause of HLH and should be actively sought for [67].
Demonstration of an infectious trigger of HLH may allow directed therapy, but does not discriminate between primary and secondary forms of the disease. Although useful diagnostic algorithms have been proposed [7], the role of phenotypic functional immunological need further prospective evaluation in larger HLH cohorts. Absent intracellular staining of perforin in NK cells can support the diagnosis of FHL-2, but variants of the disease with remaining perforin expression have been reported [58]. Absent NK cytotoxicity and is a typical features of FHL, but can also be observed in secondary forms of the disease (Fig. 5.3a). Normalization of NK cell activity during remission is important evidence for a secondary form of the disease. Measurement of CTL mediated cytotoxicity can be more informative, since it is not compromised during active HLH. Recently, measurement of expression of the lysosomal marker protein CD107 on CTL or NK cells has been introduced as a parameter to quantify secretion of lytic granules (Fig. 5.3b) [16]. Reduced degranulation can be observed in patients with FHL-3, FHL-4, FHL-5 or yet undefined genetic disorders of degranulation [29, 30, 120]. The CD107 assay is also useful in the diagnosis of patients with more complex lysosomal trafficking disorders leading to albinism and immunodeficiency [53]. Hair microscopy and evaluation of granule morphology in granulocytes may be helpful in differentiating the FHL variants from these diseases (see below).


Fig. 5.3
(a) Results of an NK cell cytotoxicity assay showing severely impaired cytotoxic activity of patient cells in comparison to cells from a healthy control. (b) CD8+ T cell degranulation assay. Short-term PHA-blasts were stimulated with anti-CD3/anti-CD28 and stained for markers of T cell activation (IFNγ) and degranulation (using the lysosomal marker protein CD107). The T cells from a patient with Chediak-Higashi syndrome fail to degranulate despite normal activation
Genetic analysis can help to establish a definite diagnosis of perforin, Munc 13-4 or syntaxin 11 deficiencies. However, in a relevant proportion of cases, diagnosis of FHL still is a diagnosis of exclusion, depending on many anamnestic, clinical, laboratory, immunological and genetic criteria.
5.2.5 Management
Without treatment, FHL is usually lethal within the first year of life. Forms with very early onset of HLH tend to be more aggressive. There is no established prophylaxis to prevent HLH in patients with a genetic diagnosis of FHL prior to the manifestation of HLH. Current protocols for the treatment of HLH (HLH-2004) include chemotherapeutic (etoposide) or immunotherapeutic (ATG) regimes in association with cyclosporine A and dexamethasone [84, 144]. Appropriate antimicrobial treatment may help to control the infectious trigger, although this will only sometimes modify the course of the disease. This includes the use of rituximab for control of EBV infection. Intrathecal MTX may help to treat the neurocerebral involvement and to limit further relapse. Unfortunately, these treatments are not always effective in controlling the primary disease and frequently fail to control relapses. Future strategies to control the acute phase of the illness may include attempts to neutralise the hypercytokinemia that is a major driver of the cellular activation. The use of a blocking anti-interferon gamma monoclonal antibody was able to induce recovery from LCMV (lymphocytic choriomeningitis virus) induced hemophagocytosis in two different murine models of HLH (perforin deficient and Rab27a) and is currently evaluated in a prospective human trial.
At present, hematopoietic stem cell transplantation (HSCT) is the only curative treatment [138, 180]. The success of HSCT depends on the extent of control of HLH prior to transplantation. Partial chimerism appears to be sufficient to prevent HLH reactivation in most cases. In a murine model of perforin deficient HLH, it has been shown that engraftment of wild type donor CD8 cells above a threshold of 10–20 % is sufficient to protect against immune dysregulation after viral challenge [202]. The estimated 3-year survival for patients with confirmed FHL in the HLH-94 study was about 50 % [85] and similar numbers have been reported in a recent single-center study of 48 patients [144]. However, the use of reduced intensity conditioning regimes (RIC) using agents such as Fludarabine, Melphalan in combination with Alemtuzumab sertherapy to effect T cell depletion may have significant benefits and can improve survival and disease free outcomes for all HLH forms [36, 124]. New targeted immunotherapeutic approaches are needed for the better control of the severe immune dysregulation prior to HSCT. Concerns regarding the neurocognitive outcome following HSCT for HLH remain with a significant proportion of children showing long term cognitive and psychosocial difficulties despite the absence of significant motor defects [93]. The reasons for these problems need to be investigated in more details.
5.3 Autoimmune Lymphoproliferative Syndrome
(ALPS-FAS, ALPS-FASLG, ALPS-CASP10, CEDS, RALD, FADD deficiency, CTLA4 deficiency)
5.3.1 Definition
Autoimmune lymphoproliferative syndrome (ALPS) (OMIM*601859) is a disease of disturbed lymphocyte homeostasis [181]. Chronic non-malignant lymphoproliferation, various autoimmune manifestations (mainly autoimmune cytopenias) and an increased incidence of lymphoid malignancies are hallmarks of the disease. Most patients harbor mutations in genes, which regulate the extrinsic, Fas (CD95) mediated, apoptotic pathway (FAS, FASLG and CASP10). Nonetheless, the genetic basis remains unknown in a relevant number of patients, suggesting also alternative pathways of disease pathogenesis. As proof of principle, a few patients with lymphoproliferation and autoimmunity were found to carry mutations in NRAS and KRAS, affecting the intrinsic apoptotic pathway. This variant of ALPS has recently been summarized as RAS-associated autoimmune leukoproliferative disease (RALD; OMIM*614470). Moreover, chronic lymphoproliferation and cytopenias may also be the leading clinical manifestation in other defined primary immunodeficiencies (e.g. combined immunodeficiencies, XLP, ICOS, LRBA deficiency, CTLA4 deficiency or PKCdelta and PI3Kdelta associated immunodeficiency), but also in hematological (e.g. Evans syndrome) and rheumatological disorders (e.g. systemic lupus erythematosus, SLE) significant phenotypic overlap has been described [106, 176].
5.3.2 Etiology
The Fas death receptor pathway is crucial for lymphocyte apoptosis induction [8, 168] and defects in the molecular machinery of this and probably other extrinsic and intrinsic pathways of lymphocyte apoptosis are the pathophysiological basis of ALPS [65, 170]. Fas is a member of the death receptor family, a family of transmembrane proteins containing similar intracellular death domains (Fig. 5.4). Activation of Fas by binding of its ligand (FasL/CD95L) requires formation of homotrimers of both molecules [153]. Their interaction mediates formation of the death inducing signaling complex (DISC), which is formed by interaction of the death domains of Fas trimers with the adaptor protein FADD and subsequent recruitment and activation of the proteases caspase 10 and 8 [168]. These molecules cleave multiple downstream targets including effector caspases that induce the death of the cell. Apart from this receptor-mediated “extrinsic” pathway of lymphocyte apoptosis, an “intrinsic” pathway triggered by cytokine deprivation, DNA damage or treatment with cytotoxic drugs has been described [137, 142]. This “intrinsic” pathway is dependent on the induction of mitochondrial enzymes. Members of the bcl-2 family of proteins such as BIM are the key molecules involved. Disturbed intrinsic apoptosis can also cause an ALPS phenotype [137, 142].


Fig. 5.4
Simplified overview of the CD95 mediated apoptosis pathway. Both FasL and its receptor Fas need to be trimerized to be activated. Upon interaction a so called „death-inducing signaling complex“ – DISC is initiated. This complex consists of the trimerized death domain, the adaptor molecule FADD (Fas-associated death domain) and activated Caspase 8 (also called FLICE-1) and Caspase 10 (FLICE-2). This complex activates effector caspases which then further mediate the induction of apoptosis
Fas is highly expressed on activated B and T cells, which appear to take a different differentiation pathway in the absence of Fas signaling. Accumulation of such abnormally differentiated cells due to impaired death leads to chronic enlargement of lymphoid tissues, in particular lymph nodes, liver and spleen [109]. Both B and T cells accumulate, but the most characteristic lymphocyte population in patients with ALPS are CD4-CD8- “double negative T cells“ that express an α/β T cell receptor (DNT cells) [19]. Neither the origin of these cells nor their differentiation pathway in vivo is fully understood. Since DNT cells are polyclonal and express markers of terminally differentiated T cells, linear differentiation from formerly single positive T cells failing to undergo apoptosis has been suggested [20, 27]. However, a more recent study suggests that DNT resemble a unique T cell subset, which originates from both CD4+ and CD8+ cells and have features of terminally differentiated effector memory T cells (TEMRA), but are CD27+CD28+KLRG1+ and do not express the transcription factor T-bet [163]. DNT cells may contribute to the pathogenesis of ALPS by producing high amounts of IL-10 and other Th2 cytokines favoring the production of autoantibodies [66, 114]. However, increased DNT cells are not exclusively observed in patients with defects of the Fas apoptotic pathway and elevated numbers may also be observed in patients with lymphoproliferation and autoimmune cytopenias of undetermined genetic cause [162]. Only markedly elevated DNT (>8 %) are clearly associated with the presence of FAS mutations [162]. B cell differentiation is also altered in the absence of Fas and data from mouse models have shown an impaired germinal center reaction leading to inappropriate survival of autoreactive B cells [80, 164].
Due to advances in the immunological and genetic understanding of the disease, the classification of ALPS has recently been revised [143]:
ALPS-FAS represents the largest subgroup and summarizes all patients who harbor germline mutations in the FAS (OMIM*134637) gene. Most patients carry heterozygous mutations (formerly referred to as ALPS type Ia), leading either to decreased Fas expression and thus haploinsufficiency or to expression of dominant negative Fas receptors [105]. In 75 % of cases the mutation is located within the intracellular death domain [186]. The penetrance of the disease is highly variable even within families, pointing towards a role of additional intrinsic or extrinsic factors, which may alter the phenotype. As proof of principle it has been observed that occurrence of a second somatic FAS mutation or somatic loss of heterozygosity (LOH) can aggravate the clinical manifestation in some patients [81, 117]. The most severe phenotype of ALPS has been observed in patients with homozygous germline mutations in FAS (formerly described as ALPS Type 0) [170, 207].
ALPS-sFAS refers to patients with somatic mutation in FAS (about 15 % of patients with FAS mutations) [51, 86]. In these patients the mutation can only be detected in sorted DNT, which harbor the mutation to nearly 100 % due to their Fas dependent accumulation [86]. Clinically the phenotype is not distinguishable from patients with germline mutations (ALPS-FAS). However, in vitro analysis of FasL induced apoptosis of T cell blasts is normal in most ALPS-sFAS patients [86].
ALPS-FASLG summarizes a small subgroup of patients with mutations in the gene encoding the FAS ligand (formerly ALPS type Ib and c). So far, only germline mutations have been reported [47, 119, 220]. The first described patient had a heterozygous dominant negative mutation, lacked DNT cells, had no splenomegaly and presented rather with features of SLE [220]. Later, two patients with homozygous mutation in FASLG (OMIM*134638) were reported [47, 119]. The clinical phenotype of autoimmune cytopenias and lymphoproliferation was similar to ALPS-FAS. In addition, one patient suffered from recurrent bacterial and viral infections [47]. In vitro apoptosis studies are normal in ALPS-FASLG and sFasL (typically elevated in ALPS-FAS) is not detectable in null mutants [119].
ALPS-CASP10 refers to a small group of patients with mutations in the gene coding for caspase 10 (formerly ALPS IIb) (CASP10; OMIM*601762). The contribution of identified mutations to the clinical phenotype of ALPS is not fully understood. While the L285F and I406L mutations seem to result in impaired apoptosis, other initially reported variants were later identified as common polymorphisms [211, 226].
Other apoptotic pathway defects
Mutations in CASP8 (OMIM*601763) were formerly referred to as ALPS IIb. However, since the clinical phenotype of these patients is more severe and includes a profound immunodeficiency due to activation defects of T and B cells, caspase-8 deficiency has been removed from the current ALPS classification and has been termed Caspase-Eight Deficiency State (CEDS) [143].
ALPS related features combined with severe bacterial and viral infections were also identified in a consanguineous kindred with homozygous missense mutations in FADD (OMIM*602457), which encodes the Fas-associated death domain protein (FADD) [24]. Although impaired Fas mediated apoptosis has been observed in vitro, FADD deficiency is not included in the current ALPS classification.
In addition, there is a significant number of patients with autoimmunity, lymphoproliferation and elevated DNT cells in whom no mutation can be found in known molecules of the Fas apoptotic pathway [162, 169]. These patients were formerly referred to as ALPS III and more recently as ALPS-U (undetermined), if Fas mediated apoptosis induction is defective or as ALPS phenotype if apoptosis is normal [143]. In most of these patients, there is no defect in Fas mediated apoptosis, indicating that defects in other intrinsic and extrinsic pathways of apoptosis might contribute to the clinical onset of ALPS.
As proof of concept, patients with activating NRAS (OMIM*164790) or KRAS (OMIM*190070) mutations leading to a defect in “intrinsic” apoptosis induction have been described with features of ALPS and significant propensity to hematopoietic tumors [137, 142]. This condition is now termed RAS-associated autoimmune leukoproliferative disease (RALD) [143].
5.3.3 Clinical Manifestations
The phenotype of ALPS is highly variable. Onset of disease ranges from birth to adulthood, but usually occurs within the first 2–5 years of life [169, 186, 187]. The typical presentation in patients with mutation in FAS includes features of lymphoproliferation, in many cases accompanied by autoimmune cytopenia of one or more cell lineages. Patients from the originally published National Institutes of Health (NIH) cohort presented with lymphadenopathy in >90 %, splenomegaly in 88 % and hepatomegaly in 72 %. Coombs positive anemia occurred in 51 %, autoimmune thrombocytopenia in 47 % and neutropenia in 23 % of the patients [186]. Autoimmune cytopenia may also be the first manifestation of the disease in the absence of lymphoproliferation. Therefore, any patient with unexplained autoimmune bi- or tricytopenia (“Evans syndrome”) should be investigated for ALPS [179, 200]. Aside from hematological symptoms, many other signs of autoimmunity like urticarial rashes, hepatitis, uveitis, thyroiditis or glomerulonephritis have been described. ALPS might therefore clinically mimic symptoms of SLE. The risk to develop malignancy is estimated to be around 10–15 % for ALPS-FAS patients and is mainly due to B-cell lymphomas [136, 195]. The majority of ALPS patients do not suffer from an increased susceptibility to infections, but those who underwent splenectomy have a high risk for overwhelming sepsis despite appropriate antibiotic prophylaxis [136].
Lymphoproliferation leading to local anatomic obstructions and autoimmune neutropenia may also predispose to bacterial infections in some patients. Although many ALPS patients have elevated IgG levels, about 10 % develop symptomatic hypogammaglobulinemia over time [164].
5.3.4 Diagnosis
The NIH defined the first diagnostic criteria for ALPS in 1999. These criteria required the presence of chronic, non-malignant/non-infectious lymphadenopathy and/or splenomegaly, elevated DNT cells and a reduced apoptosis response of T cell blasts to Fas stimulation in vitro [196].
Over the years, several clinical observations and new insights into the pathophysiology and genetics of ALPS increasingly challenged these original criteria: (1) The clinical penetrance of ALPS is highly variable and clinical presentation without lymphoproliferation (e.g. Evans Syndrome) can occur [136, 179, 187]. (2) A relevant number of patients that are positive for the original NIH diagnostic criteria (including DNT cells) do not have mutations in the Fas apoptotic pathway [162]. Thus, a number of conditions including RALD and other genetically undefined diseases (e.g. SLE) can mimic the clinical phenotype [137, 142, 220]. (3) ALPS patients with somatic mutations in FAS or mutations in FASLG have normal in vitro apoptosis [51, 86, 119]. In addition, apoptosis testing is labor intensive, costly and only available at few specialized centers.
The recently revised ALPS diagnostic guidelines address these problems [143]. A pathological apoptosis test is no longer required, if other criteria (e.g. a proven mutation in FAS) are fulfilled. The guidelines now also include the evaluation for characteristic histological changes of lymph nodes (i.e. paracortical expansion with proof of DNT cells) and positive family history. Moreover, they include vitamin B12, IL10 and soluble FasL as new diagnostic biomarkers for the disease. While these biomarkers were originally identified in retrospective analyses of two large ALPS cohorts [31, 118], a more recent prospective study confirmed their a priori positive and negative predictive value also in an unselected cohort of patients with lymphoproliferation and autoimmune cytopenias [162]. In fact, in this study the combination of vitamin B12 (cut-off 1255 pg/mL) and sFasL (cut-off 559 pg/mL) was even more useful to predict or exclude FAS mutations than the determination of DNT, apoptosis studies or germline sequencing of the FAS gene [162]. Nevertheless, the determination of DNT in combination with analysis of their Fas expression remains useful for identification of patients with a second somatic genetic event such as LOH, leading to loss of Fas expression on DNT (Fig. 5.5) [81, 117].




Fig. 5.5
(a) Flow cytometric evaluation of peripheral blood lymphocytes. In ALPS patients with germline mutation in FAS (ALPS-FAS) and germline plus additional somatic FAS mutation, resulting in loss of heterozygosity (ALPS-FAS-sLOH). The number of TCR a/b + CD4-/CD8- T-cells (DNTs) are elevated in both patients. (b) LOH results in decreased FAS expression. Pathological findings are highlighted in red. (c) Induction of apoptosis in lymphocytes via stimulation with FasL (CD95L). After ligation of Fas, T cell blasts of an ALPS-FAS patient show a reduced number of apoptotic cells in comparison to a healthy control. However, in patients with somatic FAS mutations (ALPS-sFAS) apoptosis analysis is frequently normal
5.3.5 Management
The clinical management of patients with ALPS is mainly focused on the problems of lymphoproliferation and autoimmunity. Patients with a probable diagnosis of ALPS should be clinically managed in the same way as patients with a definitive diagnosis [143].
While aggravations of autoimmune cytopenias frequently respond to short-term steroid treatment, chronic or refractory courses require the use of additional and steroid sparing agents [160, 201]. Although rituximab has been used successfully to treat refractory autoimmune cytopenias [161, 214], the generally increased susceptibility of ALPS patients to develop hypogammaglobulinemia (and the observed disturbed B cell development) should generally warrant caution with B-cell depleting therapies [164]. Mycophenolate mofetil (MMF) is another drug that has shown good response in the control of chronic autoimmune cytopenias and is generally well tolerated by patients [104, 159, 160]. But like other widely used immunosuppressants (e.g. azathioprine or cyclosporine) also MMF does not reliably reduce the size of spleen or lymph nodes [159, 201].
In contrast, the mTOR inhibitor sirolimus was found to control not only cytopenias but also lymphoproliferation (including splenomegaly) in a small case series of steroid refractory patients with ALPS [199]. Since this report the use of sirolimus has been increasingly recommended (i.e. in patients with pronounced lymphoproliferation and FAS mutations) [198, 201].
Half of the patients from the originally reported NIH cohort have been splenectomized [186]. Indications for splenectomy included refractory cytopenias, rupture or simply tremendous size, which limits quality of life or planned pregnancy. However, more recent long-term data from a European cohort suggests that splenectomy should be avoided whenever possible, as splenectomized ALPS patients are at particular risk to develop overwhelming bacterial sepsis despite appropriate antibiotic prophylaxis [136]. The successful use of sirolimus might be beneficial to avoid splenectomy in ALPS-FAS patients.
Long-term monitoring of ALPS patients for lymphoma development remains a challenge. In many cases, repeated lymph node biopsy is warranted since imaging studies, including MRI or PET-CT, are not helpful in differentiating between benign and malignant lymphoproliferation [158]. HSCT can cure the disease and has been performed in some patients with very severe refractory cytopenias [184], but is usually only indicated in a small subgroup of patients with homozygous mutations in FAS. Overall, the life expectancy of patients with ALPS is not significantly reduced. Reported deaths were mainly due to sepsis after splenectomy, bleedings and lymphoma [136].
5.4 Chediak-Higashi Syndrome
5.4.1 Definition
Chediak-Higashi syndrome (CHS) is an autosomal recessive disorder (OMIM*214500), caused by homozygous or compound heterozygous mutations in the large lysosomal trafficking protein LYST (OMIM*606897) [134]. The human gene is located on chromosome 1q. Curiously, CHS-similar syndromes have been described in many mammalian species including cats, foxes and even killer whales, one of them become famous and was held in captivity as tourist attraction (“Chimo”) in British Columbia, Canada, but likely died early due to infectious complications [167].
5.4.2 Etiology
LYST stands for lysosomal trafficking regulator which plays an important role in steps like vesicle docking and vesicle fusion. Perturbations in specific steps during the formation, maturation and trafficking of melanosomes produce the recognizable albinism. In CHS, lysosomal proteins like MHC II and CTLA-4 and perforin are abnormally distributed leading to the formation of giant organelles and inclusion bodies [54, 212]. Analysis of cytotoxic T-cells suggest that the early steps of granule formation are normal. The defect is seen as the secretory granules mature [98]. In addition, LYST is likely to play a role in mediating intracellular membrane fusion (Fig. 5.1).
5.4.3 Clinical Manifestations
The phenotype may range from mild pigmentary dilution to complete albinism. Strikingly, hyperpigmentation in sun-exposed areas are misleading in some cases. It is also characterized by a bleeding tendency, progressive primary neurological impairment and severe immunodeficiency due to lack of natural killer cell function, resulting in recurrent pyogenic infection. According to reduced iris pigmentation photophobia, strabismus and decreased visual acuity may occur.
Neurological symptoms may include seizures, cranial nerve palsy and peripheral neuropathy. Children may die early due to severe bacterial infections. The majority of children progress to the so-called “accelerated phase” with fever, jaundice, lymphoproliferation, and pancytopenia [101]. In summary, children develop the full-blown picture of severe HLH.
A small but significant number of children have a relatively mild phenotype sometimes associated with residual function of LYST (hypomorphic mutations) [215].
5.4.4 Diagnosis
Diagnosis is suspected by the clinical phenotype with albinism. The ultrastructural characteristics – giant organelles with inclusion bodies – occur in virtually all granulated cells and can easily be recognized by light microscopy (Fig. 5.6). Neutrophils, eosinophils and basophils and even platelets may contain abnormal granules. Loss of T-cell and NK cytotoxicity results from inability to secret appropriate amounts of cytolytic proteins. Light microscopy of hair shafts may even help to differentiate between other immunodeficiencies with albinism, e.g. Griscelli syndrome type 2 (Fig. 5.7). MRI scanning may document brain and spinal cord atrophy. The human LYST gene is considerably large with 55 exons and mutations are scattered over the entire gene. In future, it is likely that whole exome sequencing will facilitate molecular diagnostics also in CHS as well as many other Mendelian disorders [10].



Fig. 5.6
Blood smear from a patient with Chediak-Higashi syndrome showing giant granules in the cytoplasm of leukocytes

Fig. 5.7
Light-microscopic hair shaft analysis of Chediak-Higashi and Griscelli syndrome type 2, compared with hair shaft of a healthy control
5.4.5 Management
HSCT is the only curative approach for those children [206]. Although numbers are limited, transplantation appears to be an effective therapy for correcting and preventing hematologic and immunologic complications of CHS. An unrelated donor may be a suitable alternative for patients without an HLA-matched sibling. Long-term follow-up suggests that HSCT does not prevent progressive neurological complications.
Eapen et al. reviewed the outcome in 35 children with CHS after transplantation [52]. The group was heterogeneously treated and matched sibling donors as well as unrelated donors were used. The overall survival after 5 years was about 60 % with clearly worse outcome when patients were transplanted in accelerated phase. This argues for early HSCT once the diagnosis has been established. The 10–15 % of patients with milder phenotypes may survive up to an age of 30–40 years.
5.5 Griscelli Syndrome Type 2
5.5.1 Definition
In 1978, Griscelli et al. described two unrelated patients, a girl and boy who had been presented with many clinical features of CHS, but without the giant organelles typically for CHS [78]. Despite normal numbers of B- and T-lymphocytes the patients showed hypogammaglobinemia.
Klein et al. reviewed the immunological findings and the clinical course of seven patients showing additional differences to CHS also in terms of the pigmentation defect [175]. The hair shafts show large clumps of pigment (Fig. 5.7).
Subsequently, linkage analysis and candidate gene sequencing revealed that homozygous mutations in the ras-associated protein 27 A (RAB27A) (OMIM*603868) located on chromosome 15 cause Griscelli syndrome type 2 (GS2) (OMIM*607624) [130].
5.5.2 Etiology
RAB27 is instrumental for proper exocytosis of cytotoxic granules and thus activity of CD8+ T-cells and NK cells are severely impaired in GS2 (Fig. 5.1). In the absence of functional RAB27, granules are correctly generated, but fail to detach from the microtubules [205].
Thus, a relatively late step in the endosomal pathway is impaired. Other forms of Griscelli syndrome (GS1 and GS3) comprise albinism, but not the immunological anomalies leading to the extremely high risk for development of HLH.
5.5.3 Clinical Manifestation
The pigment dilution is usually more severe than in patients with CHS. Patients were described as having silvery gray hair and very light skin (Fig. 5.8). Some patients have serious neurologic problems, with spasticity, rigidity and convulsions, but this is inconsistently found. When progressing to HLH, CNS involvement as a result of brain infiltration is often described. The risk of HLH is particularly high in GS2 and may exceed that of CHS. Thus, prognosis is relatively poor and most patients die to HLH if not transplanted before.


Fig. 5.8
Patient with Griscelli syndrome type 2 presenting with silver-grey hair at eyelashes and eyebrows
5.5.4 Diagnosis
GS2 should be suspected in any child with oculocuteneous hypopigmentation. Hair shafts contain a typical pattern of uneven accumulation of large pigments. Microscopy of hair shafts is a simple diagnostic test and may substantiate clinical observations. Patients usually have normal numbers of B- and T-lymphocytes and no platelet defect. The characteristic hallmark is the inability of CD8+ T-lymphocytes and NK cells to lyse target cells. Lymphocyte proliferation in response to non-specific stimuli e.g. (PHA) is not impaired.
5.5.5 Management
HSCT is the only curative treatment for GS2. Due the limited number of patients no standardized procedure in terms of conditioning or donor selection has been established. Pachlopnik-Schmid reviewed a single center experience from 10 patients [146]. Four out of the seven surviving patients were cured without neurological sequelae whereas three patients succumbed due to HLH relapse or the HSCT procedure, respectively. Beside that report many small case series or single case reports document effectiveness of HSCT. It is reasonable to assume that outcome of GS2 patients may be in the same range as in other inherited HLH syndromes.
5.6 Hermansky-Pudlak Syndrome
(Type 2, Type 9, Type 10)
5.6.1 Definition
Hermansky-Pudlak syndrome (HPS) defines a group of nine different genetic disorders associating hypopigmentation with bleeding disorders. One of those nine syndromes, HPS2 (OMIM*608233), shows severe immunodeficiency with congenital neutropenia and, like the other syndromes discussed in this chapter, defective cytotoxicity of CD8+ T-lymphocytes and NK cells. Hence, also HPS2 can be regarded as an inherited HLH syndrome. Recently, an HPS-like primary immunodeficiency disease was described in a case (HPS9; OMIM*614171), caused by homozygous mutation in the gene encoding palladin (BLOC1S6; OMIM*604310) [9]. Very recently, a new type of HPS has been explained, in which mutation in the AP3D1 gene, leads severe neurologic disorder and immunodeficiency in addition to oculocutaneous hypopigmentation, which could be named as HPS10 [5].
5.6.2 Etiology
HPS2 is caused by mutations in AP3B1 (OMIM*603401), encoding the ß-subunit of AP-3 which is an endosomal adapter protein [89]. Granules fail to travel towards the immunological synapse. Loss of microtubule-mediated movement of perforin- and granzyme-containing lytic granules results in profound loss of CTL-mediated killing. Interestingly, AP3 also regulates the neutrophil elastase which may explain the neutropenia in those patients. The cell type specific AP3 expression in pulmonary epithelial cells and osteoclasts may explain the bone abnormalities and progressive pulmonary fibrosis in a significant number of patients. Impaired pulmonary repair mechanisms are thought to be the main contributing factor.
5.6.3 Clinical Manifestation
The combination of bleeding abnormalities, neutropenia and oculocutaneous hypopigmentation may guide diagnostic procedures; however, some patients were misclassified as GS2 before genetic identification revealed occurrence of HPS2. Facial dysmorphia, hepatosplenomegaly and susceptibility to bacterial infections are typical clinical symptoms described in the limited number of patients so far. The neutropenia usually responds to G-CSF treatment, the bleeding tendency shows great variability among the patients. In addition, some patients have a history of only few, mild infections whereas other succumbed due to this complication. Pulmonary fibrosis may develop early in childhood and can lead to serious sequelae. In fact, the fatal combination between fibrosis and pneumonia jeopardize patients with HPS2 substantially [72, 216].
The risk for development of HLH is not fully clear yet but a recent comprehensive survey of 22 HPS2 patients only 1 full blown HLH and 2 incomplete HLHs were found. Thus, the authors conclude that the risk for HLH is obviously lower than in GS2 or Chediak-Higashi syndrome [97].
5.6.4 Diagnosis
AP-3 deficiency resulted in increased surface expression of the lysosomal membrane protein CD63. CD63 belongs to the so-called family of tetraspannins, cell surface proteins characterized by four hydrophobic domains. Thus, flow cytometry showing bright CD63 expression together with the clinical triad of albinism, neutropenia and bleedings may lead to the diagnosis which, however, should by genetically verified [49]. Major lymphocyte subset values are within normal limits. Immunoglobulin levels as well as antibody response to vaccines are unremarkable.
5.6.5 Management
Given the apparently lower risk of HLH the preemptive HSCT cannot be recommended to date. Neutropenia can be well controlled by G-CSF but if this may trigger development of HLH is not clear yet. Control of bleedings and infections should be done according to common procedures. Patients should be monitored closely in order to detect impairment of the lung function.
5.7 Other Immunodeficiencies with Hypopigmentation
(p14 deficiency, VICI syndrome)
5.7.1 Definition
Recently, an even more complex multisystem disorder, the VICI syndrome (OMIM*242840), was added to the list of diseases with immunodeficiency and hypopigmentation [48]. In addition to immunodeficiency, it comprises defects of the corpus callosum, pontine and cerebellar hypoplasia, psychomotoric retardation, seizures, cardiomyopathy, hypopigmentation and cataracts [63]. In contrast, the few patients described with p14 deficiency (OMIM*610798) show, beside oculocutaneous hypopigmentation, short stature, severe neutropenia, defective cytotoxic T-cell function and humoral immunodeficiency [21].
5.7.2 Etiology
VICI is caused by recessive mutations in EPG5 (OMIM*615068), a key regulator gene of autophagy and implicated in the formation of autolysosomes [39]. It results in a severe block of autophagosomal clearance in the tissues where EPG5 is expressed, e.g. brain, heart and bone marrow. Thus, VICI syndrome can be regarded as a multisystem disorder of defective autophagy. In the immune system, autophagy is highly relevant for delivery of microorganisms to the lysosomes, as well as T- and B-cell survival and proliferation. P14 (OMIM*610389) is an adapter molecule (LAMTOR2) involved in the configuration of the endosomal compartment. Biallelic mutations within its 3’ untranslated region (UTR) suppresses proper processing of p14 mRNA. It serves as an instructive example that even mutations outside the coding region cause a monogenic disease [21, 108]. Strikingly, in contrast to the Chediak-Higashi or Griscelli syndrome patients, there is no defect in granule release in cytotoxic T-cells. The underlying mechanism of their defective function remains unexplained so far.
5.7.3 Clinical Manifestation
Agenesis of corpus callosum, cerebellar and pontine hypoplasia, hypopigmentation, cardiomyopathy – either dilated or hypertrophic – and a variable degree of immunodeficiency with recurrent infections may lead to early diagnosis of VICI syndrome. The prognosis is rather poor with early deaths due to cardiac failure or severe infections.
5.7.4 Diagnosis
There are several differences on clinical and laboratory findings of p14 deficiency and other immunodeficiencies associated with oculocutaneous hypopigmentation (Table 5.2). The four patients with p14 deficiency came from a large white Mennonite index family. They were presented with short stature, coarse facial features and recurrent bronchopulmonary infections. Their absolute neutrophils counts were <500/μl. Memory B-cells were reduced with consistently reduced serum IgM levels. Two of the four patients reported developed low IgG levels during adolescence requiring substitution therapy. Overall, assessment of the full phenotypical spectrum is somewhat limited due to the low numbers of patients identified yet. Immunodeficiency seems to be milder than in other syndromes described in this chapter.
Table 5.2
Differential diagnosis of oculocutaneous hypopigmentation and immunodeficiency
Griscelli syndrome, type 2 | Chédiak-Higashi syndrome | Hermansky-Pudlak syndrome, type 2 | p14 deficiency | VICI syndrome | |
---|---|---|---|---|---|
Oculocutaneous hypopigmentation | + | + | + | + | + |
Immunodeficiency | + | + | + | + | + |
Developmental delay | – | + | −/+ | − | + |
Neutropenia | − | − | + | + | −/+ |
Bleeding disorder | − | + | + | − | − |
5.7.5 Management
The multi-systemic nature of the disease prevents aggressive therapeutic approaches like HSCT. Patients with p14 deficiency were noted to have reduced memory B-cells and specific antibodies upon vaccination were partially missing. IgG substitution might therefore be indicated in selected individuals [21].
5.8 X-linked Lymphoproliferative Syndromes
(SAP deficiency, XIAP deficiency, MAGT1 deficiency)
5.8.1 Definition
The first EBV-lymphoproliferative disease was originally described as “Duncan Syndrome” more than 35 years ago [156]. In the initial kindred described by Purtillo (“Purtillo-Syndrome”) only males were affected with fulminant infectious mononucleosis and lymphoproliferation leading to subsequent HLH and death.
Up to now, there are three different X-chromosome encoded genes [SAP or SH2D1A (OMIM*300490); XIAP (OMIM*300079) and the magnesium transporter MAGT1 (OMIM*300715)], whose loss-of function mutations were associated with EBV-triggered lymphoproliferation as a characteristic clinical hallmark. Mutations in the above-mentioned genes lead to X-linked Lymphoproliferative Syndrome 1 (XLP1) (OMIM*308240), XLP2 (OMIM*300635), X-Linked Immunodeficiency associated with Magnesium defect, EBV infection, and Neoplasia (XMEN) (OMIM*300853), respectively. More recent observations, however, suggest that the clinical phenotype is much broader, e.g. XIAP deficiency is not limited to lymphoproliferation, but also includes an increasing spectrum of autoinflammatory conditions, i.e. variants of inflammatory bowel disease [145, 165, 166, 189, 219].
5.8.2 Etiology
SAP directly associates with members of the signaling lymphocytic activation molecule (SLAM) family and the protein tyrosine kinase FynT. Absence of functional SAP also perturbs SLAM-SAP signaling outcome in a manner that leads selectively to augmented Th1 cytokine production [87, 127]. There is a remarkable parallelism to the function of the IL2-inducible kinase (ITK, see below) whose inactivation also affects Th2 response [88, 110]. The uncontrolled Th1 response in combination with hyperproliferation of CD8+ cells and interferon- gamma production may contribute significantly to the parenchymal damage seen in XLP1 patients. Despite hyperproliferation, CD8+ and NK cytotoxicity is reduced when SAP is affected by loss-of function mutation. In addition to its physiological role in acquisition of T-cell effector functions, the SAP-SLAM interaction plays a critical role for the generation of T-cell dependent humoral immune responses [111] and thus may explain the low amounts of immunoglobulins secreted from B-cells of XLP1 patients. NKT cells cannot be developed when SAP expression is absent and the generation of long-lived plasma cells is also severely impaired.
The pathogenesis of XIAP deficiency has initially been linked with the ability of XIAP to inhibit apoptotic caspases, presumably leading to an increased apoptosis of patient derived lymphocytes, i.e. invariant natural killer T-cells (iNKT-cells) [171]. However, other reports have also shown that activation induced cell death and iNKT cells can be within normal limits in XIAP deficient patients [125, 189]. More recently, a second function of XIAP was published demonstrating that XIAP is crucial to facilitate innate immune signaling downstream of the bacterial sensors NOD1 and 2 [41–43]. NOD2 induces NF-kB activation leading to the production of various cytokines (e.g. TNFα, IL-1β, IL6 and IL-8) [42]. Disease-causing mutations in various areas of BIRC4 including the RING and BIR2 domains result in an impaired NF-kB response after NOD2 activation [41]. Interestingly, certain mutations in NOD2 itself are associated with the development of Crohn’s disease or an autoinflammatory condition called Blau syndrome (associated with arthritis, uveitis and granulomatous skin lesions). An increasing numbers of XIAP deficient patients are reported to suffer from overlapping autoinflammatory symptoms [145, 189, 219, 223]. MAGT1 encodes for a membrane-associated transporter which is highly selective for Mg2+ and is important for delivering Mg2+as a second messenger for PLCγ1-dependent T cell receptor signaling. Loss of function of MAGT1 abrogates Mg2+ flux in response for stimulation of the T-cell receptor. MAGT1 acts downstream of PLCgamma1 as early events in TCR signaling like phosphorylation of LAT and ZAP70 are preserved [110].
5.8.3 Clinical Manifestations
When not encountered to EBV, boys with SAP deficiency seem to be apparently healthy. Once infected by EBV, clinical consequences comprise fever, cytopenia, and hepatosplenomegaly. Aplastic anemia, vasculitis, development of bronchiectasis and lymphoid granulomatosis are more infrequently seen. Some patients develop severe signs of autoimmunity with colitis and psoriasis [62]. HLH occurs in more than 50 % of the cases, and in a recent collection of 33 SAP-deficient boys, 18 of them developed severe HLH from which 11 subsequently died [145]. Some other rare clinical manifestations have also been described: aplastic anemia, pulmonary lymphoid granulomatosis (Table 5.3). Clinically spoken, XLP1 (and XLP2, see below) should be suspected in any male patient in whom severe infectious mononucleosis progresses to HLH.
Table 5.3
Clinical and laboratory findings of SAP, XIAP and MAGT1 deficiencies
Feature | SAP deficiency | XIAP deficiency | MAGT1 deficiency |
---|---|---|---|
Clinical presentation | |||
HLH | Frequent | Very frequent | Not reported |
Fever, hepatospenomegaly | Yes | Yes | Yes |
Hypogammaglubinemia | Yes | Yes | Yes |
Malignant lymphoma | Yes, mostly Burkitt | No | Yes |
Aplastic anemia | Yes | No | No |
Vasculitis | Yes | No | No |
Genetics and function | |||
Locus | Xq25 | Xq25 | Xq21 |
Gene | SH2D1A | BIRC4 | MAGT1 |
Protein | SAP | XIAP | MAGT1 |
Expression pattern of wt | T, NK, NKT cells, platelets, some neuronal cells | widely expressed in many human cell types | widely expressed in many human cell types |
Signaling pathway affected | TCR, SLAM family receptors, FynT, | Apoptosis and survival, TGFbeta, TNF, NFkappaB, Fas | TCR |
Cellular immune defects | |||
CD4+ | Th2 lineage differentiation and function reduced | AICD increased | Low inverted |
CD8+ | Cytotoxicity reduced | AICD increased | CD4/CD8 ratio |
NK cells | Cytotoxicity reduced, | Normal | Low inverted CD4/CD8 ratio |
NK cells | Strongly reduced or absent | Conflicting data in literature | Normal |
B cells | Antibody production, isotype switching and affinity maturation reduced, strong reduction of memory cells | AICD increased | Normal |
Patients with XIAP deficiency were initially identified among patients with XLP but normal sequence in SH2D1A [171]. Following this report, it has been debated whether XIAP deficiency is correctly classified [122, 145]. While SAP and XIAP-deficiency both predispose to EBV-induced HLH, XIAP deficient patients apparently do not share the same risk as SAP-deficient patients of developing lymphoma [122, 145, 189, 223]. EBV-driven HLH is often an unrelenting disease with significant mortality. The term “HLH” refers to the characteristic morphological accumulation of lymphocytes and macrophages in the bone marrow, which phagocyte own erythrocytes or thrombocytes. Further, laboratory signs may include high ferritin, elevated sCD25 levels, hypertriglycinemia, and absent NK cell activity. Patients may deteriorate rapidly. Notably, typical phagocytosis may be missed in initial bone marrow smears although all other clinical and laboratory signs favor diagnosis of HLH. At least one third of the patients with XLP1 and XLP2 develop dys-and hypogammaglobulinemia. When boys survived initial encounter with EBV and fulminant mononucleosis, they often develop humoral immunodeficiency sometimes misinterpreted as CVID. The risk of developing lymphomas in XLP1 patients has been estimated to be nearly 200 times greater than that in the general population, also exceeding the risk in other primary immunodeficiencies, for example, Wiskott-Aldrich syndrome [76]. About 30 % of all SAP-deficient patients develop malignant lymphomas, with extranodal Burkitt’s lymphoma as being the most common subtype. The ileocecal localization is typical for those cases, but other localizations including the CNS may occur as well (Fig. 5.9) [145].


Fig. 5.9
CNS involvement of EBV-driven lymphoproliferation in a SAP-deficient patient
Accordingly, abdominal complains nausea, vomiting, diarrhea are typical clinical symptoms. Clinical suspicion of appendicitis and surgical intervention may lead to the diagnosis of Burkitt lymphoma on the basis of XLP1.
Interestingly, some patients demonstrate no evidence of EBV infection, indicating that loss of SAP itself may prone to lymphoma development [26, 197]. It is conceivable that impaired immune-surveillance by T- and NK-cells may be linked to this inherent lymphoma risk.
When compared to XLP1, XIAP-deficient boys display several important clinical differences: HLH seems to be even more prevalent (up to 80 %) than in XLP1 patients, HLH occurs recurrently, which is rarely seen in XLP1 patients in whom single and often fatal HLH episodes are described more often. In addition, XLP2 patients do not have lymphoma-proneness associated with XLP1, but instead show chronic colitis with hemorrhagic diarrheas or rectal bleeding in approximately 20 % of the patients [145]. They also show remarkable cytopenia and splenomegaly even in the absence of full-blown HLH. Because of the high prevalence of HLH, some authors prefer the term “X-linked familiar HLH” to underline the predominant clinical feature of the disease [122].
However, very recent reports have also highlighted the occurrence of additional inflammatory manifestations in XIAP deficiency, including early and late-onset inflammatory bowel disease, liver disease, periodic fevers, arthritis and uveitis [145, 189, 219, 223]. In a European cohort reported by Speckmann et al., 17 out of 25 symptomatic patients (68 %) had presenting manifestation other than HLH [189].
Due to the limited number of patients described, the full clinical phenotype of MAGT1 deficiency is only beginning to emerge. The patients identified so far showed respiratory tract infections, viral pneumonia, chronic diarrhea and – importantly – chronic EBV infection and EBV associated lymphoma. HLH has not been reported yet.
5.8.4 Diagnosis
In XLP, EBV virus load in plasma is elevated but usually not up to the level to several millions/mL as it can be seen in ITK-deficiency (see below). EBV antibodies against EBV nuclear antigen (EBNA) are frequently absent. XLP1 patients often have a marked reduction of CD27 memory B-cells, but -generally spoken- immunophenotypic analysis of lymphocyte subpopulations are rather unspecific. Lack of iNKT cells, impaired NK cytotoxicity and highly activated CD8+ T-cells are usually seen.
Analysis of XIAP expression and activation induced cell death of patient derived lymphocytes are frequently used as screening assays for XIAP deficiency [121, 171, 189, 223]. However, apoptosis tests are highly variable and protein expression can be normal in some patients with missense mutations [189]. Yet, flow cytometry with membrane permeable antibodies against SAP or XIAP are a rapid and inexpensive diagnostic tool (Fig. 5.10). Investigations of the NOD2 axis in monocytes by flow-cytometry has also been described as a reliable functional screening test, including those patients with partial XIAP protein expression [4].


Fig. 5.10
Flow cytometry screening for intracellular XIAP (a) and SAP (b) expression allows for a fast an efficient screening in patients with suspected XLP1/2. Bottom panel represent normal XIAP/SAP expression in a healthy control (Ctrl). The dashed line represents the isotype control. Caution is warranted as patients with missense mutations or mutations not affecting the binding region of the diagnostic antibody (i.e. the BIR3 or UBA domain of XIAP) might not be detected. In doubt, genetic analysis is mandatory to exclude the diagnosis
Alternatively, targeted PID gene or whole exome sequencing will be become the method of choice for diagnosis of many PIDs.
The iNKT cell numbers, typically reduced or absent in SAP and XIAP deficiency, seem to be normal in MAGT1-deficient patients.
5.8.5 Management
HLH should be treated according to established guideline with etoposide, dexamethason and cyclosporine A. Immunoglobulin replacement therapy may ameliorate the susceptibility to bacterial infections, but will not be effective against lymphoma development or other XLP manifestations. B-cell lymphomas are sometimes overwhelmingly rapid-growing and may resistant to current conventional approaches for treatment of B-cell lymphoma, including rituximab [128]. In XLP1, allogenic HSCT is the method of choice. In a large, international effort, Booth et al., collected outcome data from 32 centers worldwide that oversee children with primary immunodeficiencies and performed HSCT [25]. They identified 43 XLP1 patients who had received HSCT. Thirty-five survived the procedure with a median follow- up of 52 months. Most importantly, all patients without HLH (n = 27) survived HSCT, whereas this rate dropped down to under 50 % when HLH was present at some point before and during transplant. For details of the donor selection (four haplo donors with good outcome) and conditioning regimens, which consisted of either myeloablative and reduced-intensity approaches (one half each) [25]. The course of the non-transplanted patients was highly variable without apparent correlation to the specific side of mutation in the SH2D1A gene. Seventy percent of those patients received immunoglobulin replacement therapy. As in transplanted patients, HLH was associated with rapid decline and death, especially in younger boys. The mortality rate of HLH in the non- transplanted group was higher than 80 %. This report doubtlessly demonstrated that XLP1 has a very poor prognosis when left untransplanted. Thus, the current data suggest early transplantation from any available donor soon after definitive genetic diagnosis has been established.
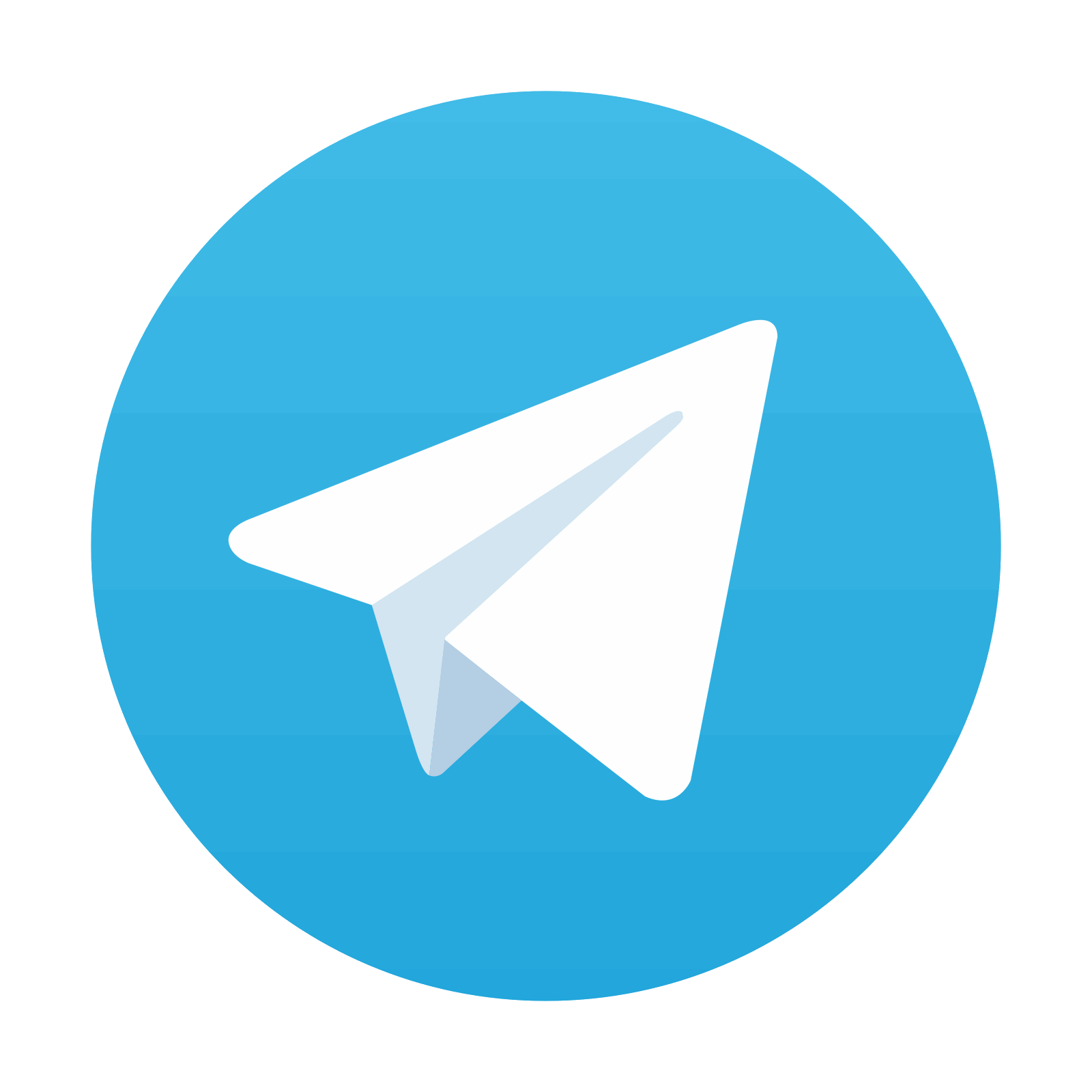
Stay updated, free articles. Join our Telegram channel

Full access? Get Clinical Tree
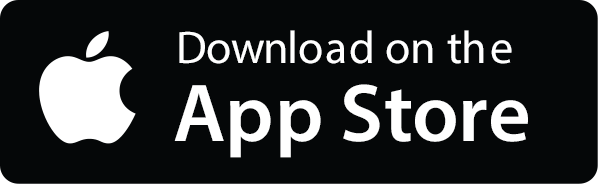
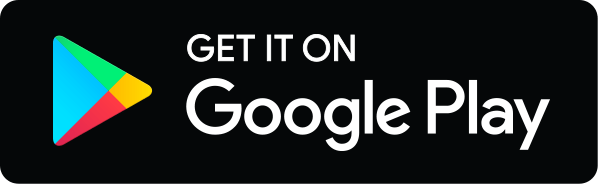