Gene
Genotype associated with RIF
Genotype associated with RM
Ethnicity
Other associated phenotypes
Apo E
Nil
E2, E3, E4 [34]
Asian, Caucasian
Higher LDL cholesterol
ACE
Nil
Insertion, deletion [35]
Asian, Caucasian
Thromboembolism, stroke, coronary artery disease
MTHFR
Nil
c.C677T, c.A1298C [36]
Multiple
Hyperhomocysteinemia, thrombophilia
MTHFD
Nil
c.G1958A [36]
Multiple
Hyperhomocysteinemia, thrombophilia
GSTT1
Nil
Null [37]
Multiple
–
HLA–G
Insertion, deletion [38]
Insertion, deletion [39]
Asian, Caucasian
–
VEGF
rs2010963, rs3025039 [42]
Multiple
–
IL–1
IL-1RN*2 [43]
rs16944, rs1143634, VNTR [44]
Multiple
–
P53
p.Pro72Pro [40]
p.Pro72Pro [45]
Caucasian
–
PAI
4G/4G [40]
Nil
IL–10
Nil
c.G1082A [46]
Multiple
–
Comparative Studies on Gene Expression Profiles
Implantation is a long continuing process, rather than a discrete event. It may be affected at different stages of the implantation (attachment, migration, and invasion). The precise genetic mechanism involved in implantation among different species may vary, transcriptome profiling around the time of implantation may be used to examine the similarities and differences among different species. However, transcriptome data analyses across species need to be relied on the knowledge of orthologous and species-specific genes. So far, many genes for animal models have not been well annotated and under a prediction-based level, and some genes are species-specific which cannot be easily annotated. The main reason is lack of full-length cDNAs sequencing data of the model organisms, which has fundamental implications on RNA-seq data mapping and qualifying. Presently, most of the gene annotation is based on cDNA sequencing data from human, mouse, and rat [27] (Table 21.2).
Table 21.2
Summary of genes or pathways in murine models account for implantation
Gene title |
Gene symbol |
Possible etiology |
---|---|---|
Death effector domain-containing protein |
Dedd |
Defective decidualization [47] |
Sphingosine kinase genes |
Sphk |
Abnormal function in sphingolipid metabolic pathway results in defective decidualization with severely compromised uterine blood vessels [48] |
Transformation-related protein 53 |
Trp53 |
|
Lysophosphatidic acid receptor 3 |
Lpar3 |
Delayed implantation and embryonic development, and hypertrophic placenta [51] |
Wingless-type MMTV integration site family, member 4 |
Wnt4 |
Defect in embryo implantation and subsequent defects in endometrial stromal cell survival, differentiation, and responsiveness to progesterone signaling [52] |
Leukemia inhibitory factor |
Lif |
Blastocysts fail to implant and do not develop [53] |
Indian hedgehog |
Ihh |
Embryo implantation [54] |
Chicken ovalbumin upstream promoter transcription factor II |
Nr2f2 |
Regulated by the progesterone-Ihh-patched signaling axis, defects in decidualization |
Heart and neural crest derivatives-expressed 2 |
Hand2 |
Antiproliferative action of progesterone in uterine epithelium WOI, impaired implantation [55] |
Early growth response gene 1 |
Egr1 |
Follicular development, ovulation, luteinization, and placental angiogenesis [56] |
There were some common pathways in endometrium shared among human and model animals. For example, leukemia-inhibitory factor (LIF), also known as cholinergic differentiation factor, was mapped at chromosome 22q12.2 in human and chromosome 11 in mice [57]. Increased LIF expression can cause recurrent miscarriage [58]. The protein encoded by this gene is a pleiotropic cytokine with roles in multiple stages [59]. Endometrial LIF increases the expression of epithelial growth factors (HB-EGF, Ereg, Ar) and implantation-related genes (Msx-1, Wnt-4) in receptive endometrium; during implantation, LIF produced by endometrium and blastocyst regulates growth and development of the embryo by increasing hCG secretion, enhancing the embryo-endometrium cross talk by increasing pinopodes and adhesion molecules (JAM-2, MUC-1, MUC-4), and synthesis of PGE2, COX-2, and mPGES-1 to stimulate decidualization. LIF can recruit a specific group of leukocytes (Eosinophil, NK cell, T and B lymphocytes) and limit the population and function of macrophages which participate in inflammatory tolerance at the maternal–fetal interface.
However, although some genes share similar pathways among different species, the regulatory mechanism may be different. For example, upregulation of msh homeobox 1 (MSX1), a transcription factor in the WNT signaling pathways, was found in endometrium of mice in the receptive stage, while downregulation was found in WNT signaling pathways of bovine, porcine, and human [27]. Through the recent high-throughput transcriptome studies of bovine, porcine and equine endometrium during the estrous cycle and early pregnancy, 42 genes have been identified which share similar up/down expression pattern, while about 2000 genes are differently expressed [60].
Transcriptome Pattern and Endometrium Receptivity
Endometrium cyclic change is regulated by steroidal hormones binding to their respective receptors and interacting with specific co-chaperones or co-regulators. Initially, the endometrium proliferates under the influence of estrogen. After ovulation, the ovary starts to produce progesterone. Progesterone receptors (PR-A and PR-B) and estrogen receptors (ERα and ERβ) are differentially expressed in different cell types in the endometrium. The endometrium becomes receptive to the implantation of embryo 7 days after ovulation, known as the window of implantation (WOI), which usually appears 7–10 days after ovulation. Implantation is unlikely to be successful outside the WOI, or even if it does take place may become defective.
A series of studies have been carried out to delineate the gene expression pattern of the endometrium at the WOI. By comparing the endometrium samples in pre-receptive phase and receptive phase of normal individuals, 134 genes with a threefold differential expression have been identified as signature for endometrium receptivity [61]. To test the reproducibility of this panel of genes, 7 individuals were recruited to sample the endometrium twice at the same day of menstrual cycles with an interval of 2–3 years, and the same array platforms were performed. The reproducibility was 100%; the accuracy in predicting the WOI was significantly higher than that of the conventional method by histologic dating [62]. It was reported that the personalized embryo transfer guided by the result of the array platform improved the pregnancy rate and implantation rate of patients with recurrent implantation failure [63]. Such a novel approach need to be confirmed by prospectively planned clinical trial.
Advances in genomics have triggered a revolution in discovery-based research to understand complex biological systems. With the innovation of technologies, more information about implantation is uncovered, and the enigmatic ‘black box’ of implantation was soon been opened.
References
1.
2.
Tan BK, Vandekerckhove P, Kennedy R, Keay SD. Investigation and current management of recurrent IVF treatment failure in the UK. BJOG. 2005;112:773.
3.
4.
5.
6.
Hassold T, Merrill M, Adkins K, Freeman S, Sherman S. Recombination and maternal age-dependent nondisjunction: molecular studies of trisomy 16. Am J Hum Genet. 1995;57(4):867–74.PubMedPubMedCentral
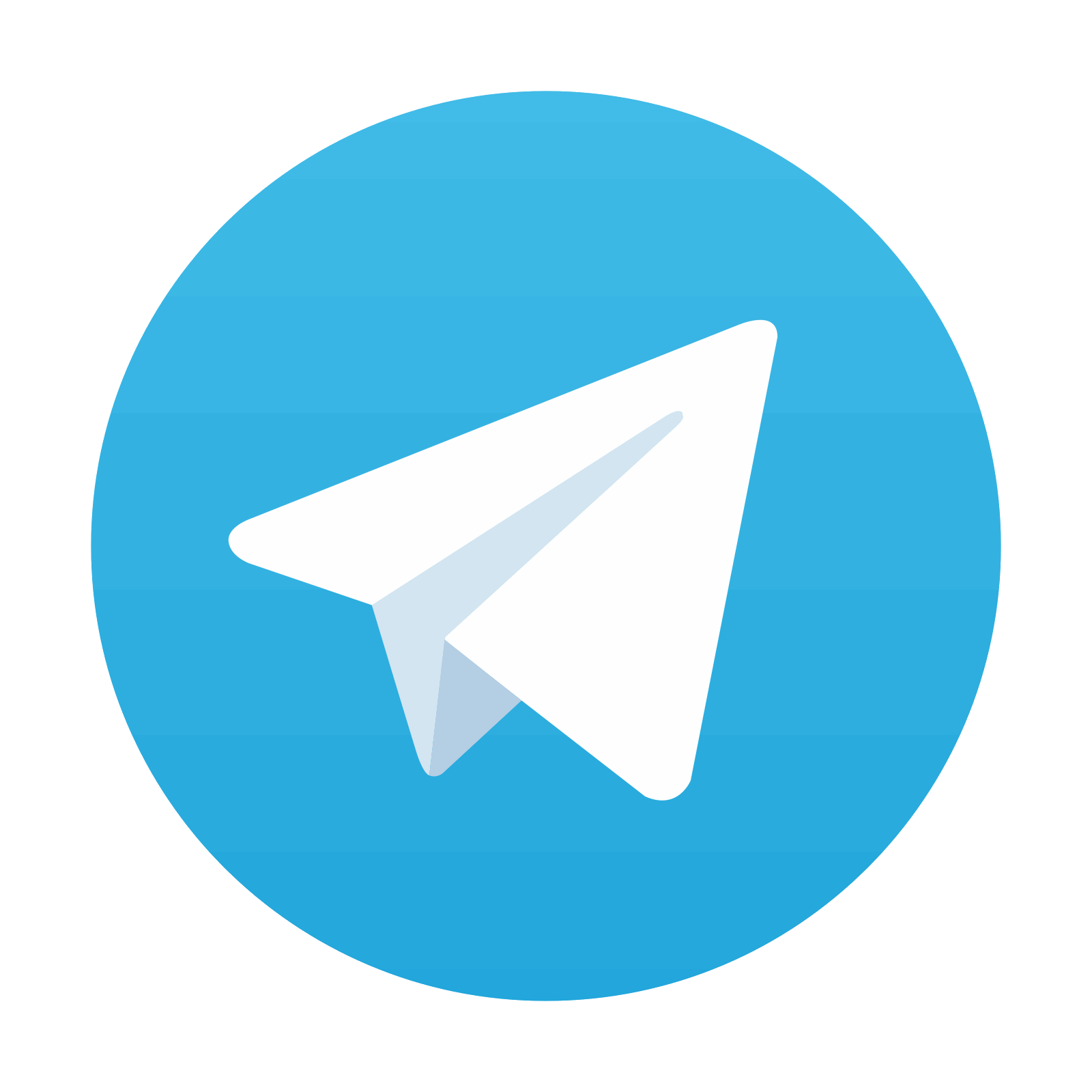
Stay updated, free articles. Join our Telegram channel

Full access? Get Clinical Tree
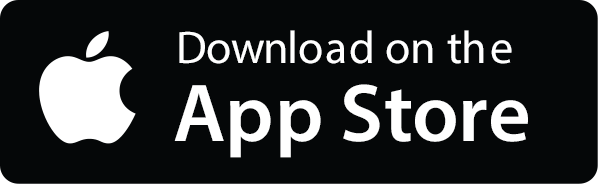
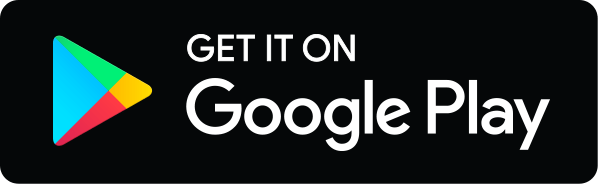