Fig. 6.1
Schematic representation of development of primordial follicle to pre-ovulatory Graafian follicle. BL Basement laminae, O Oocyte, GC Granulosa cell, ZP Zona pellucida, TC Theca cell, FF Follicular fluid, BV Blood vessel/capillary, CT Connective tissue (loose), FSHR FSH receptor, LHR LH receptor
Follicular Fluid Hormones in Folliculogenesis
In contrast to the previous belief, it has become apparent, over the past two decades that the oocyte is not a passive recipient of developmental signals from oocyte-associated GCs, termed the cumulus. It is now clear that a dialogue occurs between oocyte, GCs, adjacent theca/interstitial cells, and even with the surrounding follicles [4]. A reciprocal cooperation between the different cells is brought into play at all stages of growth and/or atresia of the follicle [6]. This complex interplay of regulatory factors governs the development of somatic cells and the oocyte and influences the follicular fluid (FF) composition. This interaction is essential for both the oocyte and the follicle’s development, beginning with primordial follicle’s transition to the primary follicle and then its continuation through to ovulation [7, 8]. The resultant oocyte’s quality influences subsequent embryo viability [9] such that the regulatory factors communicating within the follicle, and which are potentially quantifiable in the FF, may in part be responsible for the cycle’s outcome. Although such agents are largely unidentified, it is believed that they are produced locally within each follicle and include various cytokines/growth factors that are themselves influenced by the steroidal milieu [10]. Thus, it appears that the hormonal milieu in the FF is crucial in the oocyte’s development, and its analysis may help us understand its prognostic value which may influence the selection of treatment regimen for a better outcome.
Composition of Follicular Fluid
The FF is initially derived from and is similar in composition to thecal capillary serum [11–13]. It contains a conglomeration of non-hormonal components including inorganic elements such as sodium and potassium, gases, carbohydrates, mucopolysaccharides, lipids and proteins [14]. However, as development progresses, in addition to the locally produced steroid hormones, pituitary-derived hormones, other systemic hormones such as insulin and cortisol, have been found to be present in the FF. The transformation growth factor anti-Müllerian hormone (AMH) that is produced by the GCs has been the focus of much clinically applicable research recently, and this too is detectible in the FF [15, 16].
Analysis of the FF at various time points throughout the follicular phase of the natural cycle suggests that a precise sequence of hormonal changes occurs within the microenvironment of the evolving Graafian follicle [17]. The order of these changes and the relative ratio of various hormones impacts follicle’s growth and secretory activity of GCs [17]. Oocytes with the capacity to resume meiosis are more likely to originate from follicles with lower androgen: oestrogen ratio and converse is true for those that show degenerative changes [17]. Furthermore, recent significant developments in analytical techniques, specifically the multiplex immunofluorescence assay, have enabled the quantification of more than 40 cytokines and growth factors simultaneously within FF [18, 19]. The NMR spectroscopy has facilitated the identification of the FF metabolome [20, 21].
As the follicle develops, the GCs produce large polysaccharides, steroid hormones and growth factors, which cannot cross the 100-kDA follicle–blood barrier. This results in an osmotic gradient that leads to the FF accumulation and antrum formation and thus increases the FF volume [22, 23]. The FF from mature follicles in naturally cycling women is enriched with locally produced steroid hormones. Within the FF, as much as 1000 times higher concentration of oestrogen and progesterone (P) than that in the serum has been detected, whilst other pituitary-derived hormones, such as FSH, are not differentially concentrated [24].
Ovary-Derived Hormones
Steroid Hormones
There has been extensive work in the literature studying the steroid hormone composition of the FF. At the turn of the twentieth century, experiments in ovariectomized rodents and rabbits involved injection of ovarian extracts to prevent uterine atrophy. Subsequently steroid hormones, specifically oestrogen, were recognized in “liquor folliculi” [25]. In the past century, with the development of analytical methods more detailed classification of hormonal profile has emerged. In the mare, nine steroid hormones, including oestrogens, progestogens and androgens, were identified in the FF collected during oestrous and luteal phases of the cycle [26]. Oestradiol-17β was identified as the dominant steroid, and concentration of some, notably androstenedione (A4), was found to be higher in FF than in the plasma [26].
During the 1960s, it was found that the concentrations of oestrogens and progestogens in the FF of women with clinical disorders varied. For instance, follicles of women with Stein–Leventhal syndrome had relatively higher A4 and lower oestrogen concentration, probably due to a paucity of 19-hydroxylase activity [27–29]. In FF of women with dysfunctional uterine bleeding, higher levels of P when compared with normally cycling women were identified. This suggests that ovulatory dysfunction is the primary cause of dysfunctional uterine bleeding and that is more frequently seen in adolescent and climacteric period [30].
The level of steroid hormones in the FF also fluctuates throughout the natural cycle. Overall, more steroids, especially oestrogen, are found in the FF of growing follicles. The highest levels of oestradiol-17β and P are reached at the mid-point of the cycle and their levels decline as the follicle enters the pre-ovulatory phase [31]. Studies in rabbits demonstrated a transient increase in FF steroid concentration in the pre-ovulatory follicle followed by a rapid decline, and this is thought to be secondary to an alteration in steroidogenesis by the follicles. This pattern is also replicated by a decline in plasma steroid hormone concentration just prior to ovulation [32]. Edwards concluded that FF must be a repository for the steroids produced by the GCs and TCs, and their concentrations are a reflection of their evolving pattern of synthesis [14].
The type of oestrogen synthesis varies during the cycle too. During the follicular phase of normally cycling women, more free compared with conjugated oestrogens are present in the FF, but during the luteal phase, the corpora lutea contain similar concentrations of free and conjugated oestrogens [33]. Incidentally the follicles of women with polycystic ovaries even during the follicular phase display a deranged steroid hormone profile that is similar to that of the luteal phase corpus luteum of normally cycling women.
Steroidogenic pathways have been comprehensively scrutinized during the twenty-first century, and the ‘two-cell, two-Gn’ model has been described. Produced de novo from cholesterol, progestogens, androgens and oestrogens are synthesized sequentially by the GCs and TCs within the ovary and are secreted into the follicular antrum and from there into the peripheral circulation [34]. All products of the steroidogenic pathway act via specific nuclear receptors to regulate reproductive function [34]. Since each steroid hormone serves as a substrate for the next, many of these roles are inextricably linked (Fig. 6.2). Within the systemic circulation, these steroids actively participate in the regulation of pituitary gonadotrophin secretion, and within the ovarian microenvironment, they act as important paracrine factors that qualitatively influences the development of follicle–oocyte complex.


Fig. 6.2
The “two-cell, two-gonadotrophin” model. FSH Follicle-stimulating hormone, LH Luteinizing hormone
Oestrogens
The receptors for FSH have developed by the time the primordial follicle transits to the primary pre-antral follicle stage. By the late pre-antral stage, follicles have developed the capability of synthesizing oestrogen. As a consequence of the high numbers of GCs, and capacity for androgen aromatization, the pre-ovulatory follicle exhibits the highest levels of oestrogen in its FF [35]. Local paracrine/autocrine effects of oestrogen are demonstrated by the hypophysectomized rat model, where oestrogen has been shown to promote follicle’s growth, GC proliferation and development of the antrum even without the pituitary hormones [36, 37]. Furthermore, oestrogen in association with gonadotrophins also promotes the differentiation of GCs by induction of the FSH, LH, P and prolactin receptors and by stimulation of 3′,5′-monophosphatase (cAMP) accumulation. The increase in GC cAMP binding sites is also dependent upon oestrogen [38, 39].
There are two forms of oestrogen receptors (ER): ERα and ERβ [34], with the ERβ predominating in the ovary [40]. Both receptor forms have different roles; whilst ERα inhibits follicular rupture or ovulation, ERβ is responsible for follicle’s growth, prevention of atresia and induction of specific gene expressions. Studies in ER knockout oestrogen-depleted mice have demonstrated that oestrogen is vital for follicular development beyond the antral stage. Furthermore, oestrogen is also essential to maintain the female phenotype of ovarian somatic cells [34]. During COH, the levels of AMH in FF decrease in growing follicles that predominantly express ERβ, and this is thought to be due to the rising E2 in the FF [41].
Studies of FF and plasma concentrations of E2 in various subfertility-related disorders have provided an understanding into their pathophysiology. For example, even though plasma level of E2 was elevated, E2 level in the FF of women with polycystic ovarian syndrome (PCOS) did not show a corresponding rise unlike normal women undergoing IVF with male factor infertility (MFI) or controls [42]. Furthermore unlike normally cycling controls, the free and conjugated oestrogens had similar concentrations in the FF of women with PCOS [33]. On the other hand, similar E2 levels were observed in the FF of women with endometriosis, normal women undergoing IVF with MFI or controls [43]. This exemplifies disordered steroidogenesis within even the pre-ovulatory follicles of women with PCOS which may impact the development of the follicle–oocyte complex.
Progestins
One of the most abundant hormonal components of FF, P, is critical for the end stages of follicle development and ovulation [34], and knockout mice lacking P fail to ovulate [44]. The role of P is inextricably linked with E2 as increased levels of oestrogen induce the production of P receptors.
The cellular response to P is more disparate when compared with E2, and therefore, it is harder to implicate, although it plays a key role in ovulation, implantation and maintenance of pregnancy [45, 46]. Within the follicle, progestins have been reported to have a direct effect on GCs. In rat GCs, P increases cAMP and consequently enhances the GCs response to FSH, but it also inhibits FSH-stimulated E2 production by GCs, a function that may be of value when providing luteal support with high-dose parenterally administered progesterone in women at risk of OHSS after IVF.
The P levels in FF are significantly affected by age and rise with advancing age. They are also increased with reduced ovarian reserve (ROR) [24]. Follicular P levels have been found decreased in women with PCOS and endometriosis [42, 43]. The precise cause or impact of this is not clear. In an IVF cycle, following COH, the relationship of serum P on the day of hCG and pregnancy rate has been debated extensively because of contradictory results [47, 48].
Androgens
Whilst predominantly involved in male development, androgens are also expressed in the ovary and the fallopian tube and are critical in the development of the early follicle [49]. Although in the female circulation adrenal cortex is the major source of androgens, ovarian follicles also contribute towards their FF and systemic levels. Androgens may affect folliculogenesis directly via androgen receptors (ARs) or indirectly through aromatization to oestrogen. Androgens, predominantly A4 and testosterone (T) are produced by the TCs in response to stimulation by LH (see Fig. 6.2). In vitro and in vivo studies have demonstrated that androgens can stimulate the growth and development of follicles in mammals [49, 50]. Female mice lacking a functional AR are less fertile and have a shorter reproductive life [49]. ARs are located on GCs, TCs and stromal cells [51]. The AR expression is more pronounced in the early and intermediate stages of folliculogenesis [52]. During this time, local androgen production facilitates the transcription of genes involved in the transition of pre-antral follicles from the reserve to the growth pool as well as the subsequent development of the more mature follicle [51]. Exposure to androgens augments FSH receptor expression in developing GCs, thus enhancing FSH-induced cAMP formation required for the transcription of genes that control GC proliferation and differentiation, as evidenced by an increase in E2 and P production [53–56]. Activation of the AR in the oocytes of primordial follicles and in the GCs and TCs of growing follicles enhances the expression of insulin-like growth factor (IGF-1) and its receptor. This in turn leads to follicular recruitment and development [57]. Thus, androgens may play an important role in selection and transition of primordial follicles to antral stages.
However, in mature follicles, the AR expression is reduced and there is associated reduced androgenic activity, which indirectly implies that androgens at this stage may adversely influence the appropriate maturation of the follicle and may induce atresia [48]. In vitro mice oocytes arrest in meiosis after T exposure, and hence, in late stages of follicle development, T is thought to adversely influence oocyte maturation [58].
Age and ROR have not been shown to affect T levels in the FF [24]. However, the FF of mature follicles in women with PCOS has been found to contain significantly raised T levels [42]. Hyperandrogenism is a classic symptom of PCOS, and the finding of raised T level in the FF of women with PCOS provides further evidence that abnormal steroidogenesis by the ovary is responsible for the systemic excess of androgens and the finding of poor-quality oocytes. It has been proposed that in this condition, TCs fail to respond to Gn down-regulation and this associated with TC hypertrophy in PCOS leads to hyperandrogenism [59].
Anti-Müllerian Hormone
AMH is a TGF-β family growth factor, is secreted by pre-antral and antral follicle GCs and is present in both the FF and the circulation. In the past decade, it has also been implicated in folliculogenesis and is thought to play a role in both the growth and differentiation of the follicle [60]. One primary function of AMH is its inhibition of primordial follicle growth. Even though AMH knockout mice have normally developed ovaries and are fertile, they have a narrow window for fertility [61]. AMH serum levels vary throughout the female lifespan, with increased levels in early childhood, a peak in the early 20s, followed by a decline to undetectable levels in menopause [60, 62, 63]. AMH decreases sensitivity to FSH and is negatively correlated with FSH and E2 [64–66]. AMH expression has been found to be differentially regulated by E2 and depends on the type of ER. During COH, its levels in FF decrease in growing follicles that predominantly express ERβ, and this is thought to be due to rising E2 [41]. However, the growing follicle’s AMH level is only a marker of its status, and its level thereafter is not affected by the fluctuations in other hormones during the menstrual cycle [65].
The levels of AMH in the FF also reflect serum concentrations, thus decreasing with age and ROR. The FF AMH levels are negatively correlated with FF FSH concentrations. AMH in both serum and FF predicts ovarian response to COH in an IVF cycle and this correlates with treatment outcome [16]. Higher AMH concentration in the FF has been correlated with higher fertilization, pregnancy and implantation rates in women undergoing COH [67, 68]. In mice, even the oocyte has been shown to influence AMH expression, thus suggesting a direct role for the developmentally competent oocyte. It is thought that this effect may be mediated by oocyte’s activation of various physiological processes in the surrounding GCs [70].
Pituitary-Derived Hormones
Pituitary hormones, particularly Gn, play a critical role in folliculogenesis. Historically continuation of pre-antral follicular growth following hypophysectomy has been demonstrated, thus establishing the notion of the Gn-independent phase of folliculogenesis [71]. By quantifying the levels of Gn in the FF and plasma during the follicular phase of the menstrual cycle, their actions with respect to follicular steroidogenesis have been studied and their key functions have been established [17]. It has been found that in the FF, E2, which has long been recognized to increase the sensitivity of the ovary to FSH, is highest in the mid-follicular phase, whereas LH significantly increases in the late follicular phase. Pre-ovulatory follicles have higher concentrations of E2 and P even though the LH and FSH levels are only 30–60% of those found in serum [17]. FSH is essential for GC proliferation and development of the basement membrane, whilst both FSH and LH are responsible for the follicular antrum formation and development of the thecal vasculature [72]. Follicle’s growth is accompanied by an increase in both the quantity and quality of Gn binding sites [14]. Follicles containing high levels of FSH in the early follicular phase have a higher mitotic index and a faster growth rate [17]. The GC LH receptor expression also correlates with increased mitotic activity. Subsequent to the entry of LH into the follicle, there is cessation of mitotic activity and associated P secretion increases. A correlation between the LH level in the FF and oocyte maturation has been demonstrated. Oocytes in metaphase I and II stages have been found to originate from follicles containing comparatively higher LH levels [17].
Follicle-Stimulating Hormone
The predominant function of FSH is to stimulate the development of the primordial follicles via a feedback loop enabling selection and maintenance of the dominant follicle [73]. FSH blocks apoptosis in pre-antral follicles, consequently FSH receptor (-R) knockout mice are anovulatory, lacking circulatory oestrogen, possess significantly less P, and serum T levels are elevated, thus implicating FSH firmly in the steroidogenic pathway [74]. Serum FSH levels rise at puberty and climax at the menopause. Higher levels of serum FSH signify ovulatory dysfunction and in younger women are indicative of a ROR [75, 76]. Conversely, despite ovulatory dysfunction, young women with PCOS exhibit lower levels of FSH [77]. There is a paucity of research investigating FSH levels in the FF during natural cycles. A study identified higher concentrations of FSH in the FF of older women with ROR, but younger women with ROR and healthy controls exhibited similar levels. The authors wondered whether the elevated intra-follicular FSH level in older women was simply due to higher serum FSH concentration or whether it was secondary to reduced FSH receptor expression [24]. In stimulated cycles of women with endometriosis, FF concentration of FSH was not altered [43], but to date there are no studies that have investigated the FF FSH level in women with endometriosis or PCOS.
Luteinizing Hormone
LH is fundamental to supporting steroidogenesis via TC LH receptors (-R) [78]. LH-R knockout mice are infertile with atrophic ovaries and have low serum E2 and P levels [79]. Whilst LH is essential for normal oocyte and embryo development, follicles exposed to high concentrations of LH may luteinize prematurely, which is thought to compromise normal oocyte development [73]. Basal LH levels in the serum rise during reproductive life and peak at the menopause [80, 81]. However, FF levels of LH remain consistent throughout reproductive life [24, 82] and are not affected by age, ROR and endometriosis [24, 43, 82].
Prolactin
Prolactin and E2 levels in the FF of the maturing follicle are inversely related. Whilst E2 levels in FF progressively increase throughout the follicular phase, FF prolactin levels decrease, but this reduction is restricted to the follicle destined to become the pre-ovulatory Graafian follicle [83]. This fall in prolactin is also inversely related to the progressive increase of P in FF [83]. A similar inverse correlation between prolactin levels and the number of LH receptors was demonstrated in the follicle of postpartum cows [84]. However, with regard to the relevance of prolactin levels following COH with gonadotrophins, the findings are conflicting [85–93].
Growth Hormone
Growth hormone (GH) is primarily produced by the pituitary but is also produced locally within the follicle itself. It acts on GH receptors of the GCs, TCs and luteal cells and enhances GC FSH-dependent E2 secretion [94] directly or via an insulin growth factor (IGF)-1-mediated mechanism and perhaps also the expression of FSH and LH receptors in GCs [95]. GH also impacts follicular growth [96], has been shown to increase the sensitivity of ovaries to Gn stimulation [97] and hence has been suggested as an adjuvant therapy in assisted reproduction [98]. However, a clear association between intra-follicular GH and successful pregnancies has not been identified and results have been contradictory. Both lower [87] and higher [99] GH levels in the FF of follicles generating oocytes/embryos resulting in pregnancy after in vitro fertilization (IVF) have been reported.
Systemic Hormones
Various systemic hormones have been identified in the FF.
Insulin
Insulin stimulates GC aromatase activity as well as steroid production [23, 100]. Furthermore, it has been recognized as a regulating factor for oocyte maturation [101]. Insulin has been identified in FF following COH with gonadotrophins and clomiphene citrate (CC), and its concentrations have been found to correlate with P concentrations in the FF [102].
Cortisol
At the time of the LH surge, an increase in total and free cortisol is apparent in FF, and it is postulated that cortisol, together with its regulation by 11β-hydroxysteroid dehydrogenase, is involved in oocyte maturation and ovulation [103]. Similarly, following COH in IVF cycles, a high cortisol/cortisone ratio in FF has been associated with increased pregnancy rates, and it is thought to enhance final oocyte maturation as well as subsequent embryo implantation [104, 105]. Low FF cortisol levels in women with endometriosis have been suggested to contribute to follicular dysfunction associated with subfertility secondary to this condition [106]. However, a trial involving administration of adjuvant corticoid therapy during IVF has failed to improve oocyte fertilization, embryo implantation and clinical pregnancies [107].
Renin
Renin levels have also been studied in FF throughout natural cycles [108]. Active renin levels increased in the FF during the follicular phase, peaking in the peri-ovulatory phase following the LH surge (in patients undergoing laparoscopic sterilization) or exogenous hCG administration (in modified natural IVF cycles), thus supporting the hypothesis that the ovarian renin–angiotensin system is also under Gn control [108].
Follicular Fluid Hormones in Natural Cycle IVF
Evidence for the Detrimental Impact of Exogenous Gonadotrophin Stimulation
Gonadotrophins with COH have improved success rate of IVF by increasing the number and availability of embryos for selection before transfer [109–111] and also by enabling the cryopreservation of supernumerary embryos [112, 113]. However, there is increasing evidence that COH may be detrimental to a variety of pathways involved in reproduction. The foundation of COH involves bypassing physiological regulatory mechanisms in order to support the growth of multiple follicles. This is achieved via the administration of exogenous gonadotrophins to achieve levels above the obligatory threshold for selection of the dominant follicle; hence, the entire cohort of recruited follicles is able to attain pre-ovulatory status [112]. An agent to desensitize the pituitary and prevent premature LH surge is also given in either a long protocol (Gn-releasing hormone [GnRH] analogue) or a short protocol (GnRH antagonist), thus preventing the adverse consequences of supra-physiological E2 levels leading to premature LH surge and luteinization or ovulation. An ovulation trigger using either human chorionic gonadotrophin (hCG) or GnRH analogue (in short protocol) is finally administered.
There is growing evidence within the literature that indicates that COH has adverse consequences upon oogenesis, embryo quality and endometrial receptivity [114–120]. Obtaining >10 oocytes per woman has been shown to negatively affect their quality as measured by oocyte/embryo morphology, fertilization and implantation rates. [114, 121]. Others have determined that the optimum number of retrieved oocytes associated with a clinical pregnancy is 13 [122]. An excessively high level of E2 is seen in ovarian hyperstimulation syndrome (OHSS); this is commonly associated with poor-quality oocytes and serves as an extreme example of the detrimental impact of COH [123]. There is evidence that COH may disturb oocyte maturation and completion of meiosis, thus increasing the likelihood of resultant aneuploid oocytes and/or embryos [117, 124]. In rodents, a delay in embryo development has been attributed to exogenous Gn administration [125, 126].
Suppression of LH levels approaching the peri-ovulatory phase has been associated with downstream disruption in follicular steroid synthesis. Accordingly, stimulation protocols incorporating exogenous LH were established and an improvement in the percentage of diploid and good-quality embryos was reported [127, 128]. However, such findings were not consistent, and other studies detected a decrease in pregnancy rates and increase in miscarriage rates following the incorporation of exogenous LH into protocols [129, 130]. This leads to the suggestion that there is an “LH window” below which E2 production is inadequate and above which high levels of LH promote premature luteinization or degenerative changes [131]. Excessive LH may stimulate TCs, increase FF androgen levels, switch off E2 synthesis and increase FF P levels prematurely all of which are known to have a detrimental effect on oocyte development.
Notably, in large clinical studies, comparing COH protocols with human menopausal gonadotrophin (HMG), recombinant (r)FSH and rFSH+LH, a definite difference in pregnancy rate per oocyte retrieved was identified [127, 132]. It is thought that low-dose exogenous LH during COH administered either as HMG or as rFSH+LH combination positively affects the quality of the embryos [127, 133], thus implying that type of exogenous Gn administered has a differential impact on follicular metabolism.
Natural Cycle IVF
Natural cycle IVF (NC-IVF) was initially proposed as an alternative treatment for older women and poor responders [134]. Given that the natural cycle that results in a pregnancy is the prototype that we aspire to reproduce in all women, with the emerging evidence of the detrimental effects of exogenous Gn stimulation, an interest in developing protocols avoiding COH has been gaining momentum. This is with a view to creating a cohort of better quality embryos as opposed to obtaining as many as is possible [135]. Other more recently realized benefits of NC-IVF include supporting the international drive to reduce multiple pregnancies with elective single embryo transfer (eSET) and avoiding the deleterious impact of COH induced OHSS. However, the success rates after NC-IVF are much lower than those achieved with COH-IVF cycles and have been reported at less than 10% per cycle [109] in one study and 15.2% live birth rate per initiated cycle in a more recent analysis [136]. Modified natural cycle IVF (MNC-IVF) is the term applied when drugs are administered in a spontaneous cycle to minimize the risk of cancellation due to spontaneous ovulation; hCG is given to induce final oocyte maturation [137] and for luteal support. The third alternative of Mild ovarian stimulation IVF (M-IVF) has also been proposed as a compromise utilizing low-dose Gn stimulation aimed at generating no more than eight oocytes per cycle [138]. A recent publication detailing the outcomes from a Swedish clinic offering MNC-IVF and M-IVF described high cycle cancellation rates of 53.5 and 39.6%, respectively, but ongoing pregnancy rates in women ending up with an embryo transfer (ET) were significantly superior to earlier publications with natural cycle IVF at 26.7 and 27.2%, respectively, per ET [139]. However, including the cancelled cycles, success rate per treatment attempt with MNC-IVF and M-IVF remains significantly lower than that with COH although cost and risk is also lower and more treatments can be performed repetitively in consecutive cycles than is possible with COH.
Follicular Fluid in Natural Cycles
As previously described, during normal folliculogenesis, FF composition exhibits dynamic fluctuations as a consequence of individual cell types responding to gonadotrophins by secreting various hormones, growth factors and cytokines which in turn influence the development/function of both the somatic cells and the oocyte [140, 141]. As oocyte quality impacts ensuing embryo viability [141], it has been postulated that the disruption in the delicate balance of these intra-follicular mediators as a consequence of exogenous Gn stimulation may impact the outcome of the treatment cycle [99, 142, 143]. Precise regulation of hormone pathways is a prerequisite to sustain healthy physiology, and this fundamental purpose is emphasized with a multitude of endocrine disorders being associated with dysfunctional follicles. Such hormonal dysregulation in FF also underpins several pathologies in the reproductive system [29, 43, 46, 50, 69, 106]. Study of the intra-follicular endocrine environment in natural cycles provides a greater understanding of the impact of pathological disorders and exogenous stimulation with Gn upon the developing follicle and oocyte. Such investigations will also help in establishing whether or not mild stimulation with lower doses or exogenous hCG in natural cycle IVF minimizes such risks and whether in randomized studies better success rate per treatment attempt can be achieved.
Comparing Follicular Hormonal Milieu Between NC-IVF and COH-IVF Cycles
Subsequent to the introduction of IVF, a number of studies have analysed FF from women undergoing COH. Majority of these studies were instigated to identify prognostic variables for the likelihood of retrieving a viable oocyte, successful fertilization, high-quality embryo morphology and ultimately a clinical pregnancy [144–146]. There is a paucity of publications studying the intra-follicular endocrine profile of unstimulated ovaries [147–152].
Original studies included McNatty et al. [147] measuring hormones in follicles resected from ovaries removed following oophorectomy at various stages in the menstrual cycle using an immunoassay (IA) as well as quantifying GCs and assessing oocyte maturity. Their findings led them to postulate that follicles with the potential for further development had a high number of GCs relative to their diameter, contained higher concentrations of 17-β-oestradiol and/or GCs with the capacity to generate this hormone in response to FSH together with a germinal vesicle stage oocyte [147]. Subsequent to this fundamental work, intra-follicular E2 concentrations were shown to rise in dominant follicles and correlate with the diameter of these follicles, implying that significant increase in aromatase activity is restricted to the dominant follicle. This research also only identified P production in the largest dominant follicles, and the authors speculated a limited role for P during follicle development [151].
One of the early studies investigated the impact of pituitary down-regulation and COH on the intra-follicular hormonal milieu and compared FF steroids and hCG levels in women undergoing either a long or short stimulated cycle, or MNC-IVF in which exogenous hCG was administered [140]. In this study, the first aspirate was the only sample analysed and only if an oocyte was retrieved. Both E2 and T levels were significantly higher in the NMC FF when compared with COH FF [140]. Lin et al. [153] also demonstrated similar findings in the FF of naturally cycling women, who in this study had also not received the exogenous hCG. The FF P levels were equivalent between the COH and NC patients, and this led them to suggest only a minor role for P in follicular development [153]. This study utilized IA (solid-phase fluoroimmunoassay [140] and 125I RadioImmunoAssay for hormone analysis [153]). The IA is limited in part because of the diminutive quantity of FF in individual follicles and the inability to quantify multiple analytes simultaneously in extremely small volume samples. Furthermore, there is a high potential for cross-reactivity and this raised doubt regarding the precision of results and hence the conclusions of these studies.
Subsequently, Kushnir et al. [154] attempted to improve the accuracy of quantification of the hormone concentration using liquid chromatography-tandem mass spectrometry and this also enabled simultaneous quantification of multiple steroids. Using this technique, levels of 17-hydroxyprogesterone (17-OHP), total androgens and oestrogens were found to be 200–1000-fold greater in the FF than in the serum confirming the Edwards conclusion of the FF being the repository for hormones produced by GCs and TCs [14]. In keeping with other studies [148], androgens were also the most abundant class of steroids in the FF of natural cycles. However, the absolute concentrations of T, A4 and E2 were much lower than those previously measured by IA [154]. Furthermore, contrary to earlier work, it appears that FF as measured in young healthy egg donors has lower E2 in FF after COH [155], and this finding has also been further verified in other studies [140, 153].
Contradictory results have also been reported in the FF samples from women undergoing COH where they not only had significantly higher levels of E2 compared to NC FF but there were also higher levels of pregnenolone, 17-OHP and cortisol [154]. Notably, this study is limited by the low numbers of participants, and also the fact that the women in the NC arm underwent laparoscopic follicular aspiration prior to day 7 of the follicular phase, with aspiration of all follicles measuring 5–8 mm, and pooling of follicular fluid; hence assessment of the features of a dominant follicle was not possible.
The Association Between FF Endocrine Profiles, Resultant Oocyte and Embryo Quality in NMC-IVF and COH-IVF
Even though there is increasing evidence supporting the deleterious effects of COH, the results per cycle in NC and MNC-IVF are poorer when compared to those in COH-IVF cycles. Jančar et al. [156] attempted to ascertain whether this is associated with a suboptimal intra-follicular environment of NC and MNC-IVF cycles. When a MNC-IVF cohort (hCG to induce ovulation) was compared with a COH cohort (short antagonist stimulation protocol utilizing rFSH) [156], there were no differences in embryo quality but the implantation rate per ET was 4.9% in the MNC cohort compared with 36.4% in the COH cohort (p = 0.031). The authors hypothesized that the administration of hCG (administered when the dominant follicle measured ≥16 mm in diameter and serum E2 exceeded 0.39 nmol/L) in MNC-IVF cycles may have been too premature and may have had detrimental effect on the endometrium. Unlike what one would have expected, amongst the MNC cohort, follicular AMH, FSH, E2 and P concentrations were not found to correlate with embryo quality even though the overall results were higher [67, 68]. By contrast, following COH FF AMH levels were significantly lower in empty follicles or those yielding degenerated oocytes. We think that one explanation for this discordant result could be the follicular heterogeneity seen following exogenous gonadotrophins, which is not a feature of MNC-IVF. When comparing LH in FF of COH group, its level was significantly lower in those follicles that yielded oocytes and ultimately embryos that resulted in an implantation after transfer when compared with follicles that generated embryos that were transferred but which did not implant [156].
Notably LH was significantly higher in the FF and serum of the MNC cohort when compared with the COH cohort, and the authors have attributed to a spontaneous LH surge on the day of oocyte retrieval, in spite of early hCG administration. A high level of LH would be expected to correlate with a high P level secondary to enhanced luteinization. By contrast, serum P concentration was significantly lower in the MNC cohort, implying that in fact premature luteinization had not actually occurred. These discordant results are difficult to explain and the authors have suggested that synchronization between the developing oocyte and receptivity of the endometrium may be affected in such circumstances. [156].
Recently, de los Santos et al. [155] conducted a study on healthy oocyte donors undergoing MNC-IVF (hCG administered when the dominant follicle reached 18 mm in diameter) and COH-IVF (GnRH agonist long protocol). The dominant FF in the MNC cohort and the FF from one of the larger follicles in the COH cohort were analysed. In contrast to the studies previously described, T was higher in the COH cohort. However, in agreement, E2 was significantly higher in the MNC cohort together with A4 and LH. Interestingly, in the MNC cohort, in spite of these findings the E2: T ratio was conserved. Previous work has shown that the E2: T ratio correlates with fertilization and cleavage rates [157, 158]. In this study, in agreement with Jančar et al.’s findings [156], LH in the FF was higher by approximately 100-fold in the NMC cohort, but again this has been presumed secondary to an undesirable premature LH surge in this group. Once again there was no correlation between the FF LH and P levels in both cohorts. Follicular FSH and P were the only follicular hormones that did not appear to be affected by COH. In this study, regardless of intra-follicular hormone levels, there were no differences in embryo morphology between the two groups. However, in the same study the oocyte meiotic spindle and cumulus gene expression profiles were simultaneously studied in the two groups. COH was found to induce changes in gene expression, and together with the alteration in the FF hormone profile, it has been suggested that Gn stimulation may affect immune processes, meiosis and ovulation pathways.
Pursuing the notion that AMH might be a positive marker for oocyte viability following COH, in an attempt to identify the mechanism stimulating AMH production, Andersen and Lossl compared various COH regimens and proposed that a follicle rich in hCG and androgens promoted AMH secretion [159]. A hypothesis thus arose: suppression of endogenous LH in COH cycles may lead to a reduction in TC androgen production and in turn lower intra-follicular androgen concentrations and ultimately lower AMH levels with associated poorer oocyte quality. Whilst this had not been identified in previous studies [155, 156], von Wolff et al. investigated this hypothesis further and simultaneously defined the NC intra-follicular endocrine milieu. They studied the effects of gonadotrophins on follicular physiology by comparing FF hormones following COH with HMG in short cycles (FF analysed from first large follicle aspirated) and in NMC-IVF cycles (hCG administration to trigger ovulation) [160]. AMH, T, E2, A4 and LH were all higher in the FF of the MNC cohort.
Conversely P concentrations were similar in both protocols in the FF, although the serum levels were significantly raised in the COH cohort. The authors demonstrated a positive correlation using regression analysis between AMH and T (r = 0.34, p = 0.0002). The increased T in the MNC cohort may be explained by the elevated LH activity as previously described and is supported in this study by a positive correlation between T and LH, thus supporting, although not proving, the supposition of a dependency of the AMH concentration on T levels. The authors conclude that in FF, LH plays an important role in indirectly contributing towards oocyte quality, and use this argument to favour protocols which enhance LH levels, including both MNC-IVF and also short GnRH antagonist cycles where LH concentrations are less suppressed when compared with long GnRH agonist protocols. Therefore, it has been suggested in studies comparing these stimulation protocols that implantation rates in short cycles are higher [161].
Pathology Related Follicular Hormone Profiles in NC-IVF
A prerequisite for NC-IVF is the ability to spontaneously ovulate. This therefore precludes women with ovulatory dysfunctional PCOS. For this reason, FF endocrine profiles in such cycles are absent from the literature. However, there are data pertaining to intra-follicular hormone levels in different cycle regimens. It is well established that the FF in women with PCOS contains high T concentrations, regardless of the stage of follicle development, as well as reduced P levels, implicating that paracrine factors may inhibit follicular cell P secretion. Such findings offer some understanding into the complex pathophysiology of this disorder [162]. Interestingly, in short cycles, rFSH was found to result in higher FF P concentrations in PCOS women when compared with HMG [163]. Thus, demonstrating how unravelling the intra-follicular milieu in subfertility-related pathologies may assist in designing individualized treatment protocols.
Whilst ovulation may occur spontaneously in women with other subfertility-related pathologies, there remains a paucity of research into the intra-follicular milieu of such patients in natural cycles. In one small study, both endometriosis and unexplained infertility patients demonstrated reduced LH concentrations in FF, implying that there may be a shared common pathophysiology of impaired LH secretion [164]. Furthermore, FF AMH concentrations have been found to be similar in women with mild endometriosis when compared with control subjects [165]. Further work in this area is warranted and in particular may assist in determining undiagnosed endocrine pathology in women considered to have unexplained infertility.
Weaknesses in Published Literature
An important discussion regarding the impact of exogenous Gn upon the ovarian follicle relates to the consequential follicular heterogeneity. Numerous interdependent events affect oocyte maturation and the acquisition of developmental competence, including interactions between follicular somatic cells and the oocyte [4, 7], the composition of the FF and the vascularity of the follicle [166, 167]. Many of these mediators within the follicle including hormones alter with follicle size and oocyte growth in stimulated cycles [168].
Whilst McNatty et al. in their early work demonstrated an evolving endocrine profile throughout the follicular phase in natural cycles which related to follicular antral diameters [17], the studies described compared COH cycles with NMC cycles and generally have analysed the FF from the lead follicle only, or only from the first follicle aspirated in the COH cycles. Thus, the variation in the hormone profiles relative to the size of the pre-ovulatory follicle is inherently and by design not addressed in these studies. Ultimately, the quality of the oocyte is determined by its nuclear and cytoplasmic maturation [169], which have been shown in themselves to be asynchronous in stimulated cycles [170]. Our studies have also demonstrated asynchrony of follicular size and oocyte quality as well as heterogeneity between follicles of the same volume.
Furthermore, the authors have found that the lead follicle in stimulated cycles is not consistently the follicle that yields the oocyte with the greatest viability. Exogenous gonadotrophins accelerate the follicular phase by increasing the follicular growth rate [171], and this may also accelerate cytoplasmic maturation of oocytes in all follicles in both the leading and the secondary cohorts. Consequently, follicles from secondary and tertiary cohorts are also capable of yielding mature oocytes that have a good developmental potential. The authors think that failing to cater for follicular heterogeneity and asynchrony of follicular and oocyte development induces errors in assessments when correlating FF endocrine profiles and these errors could lead to lack of or incorrect conclusions.
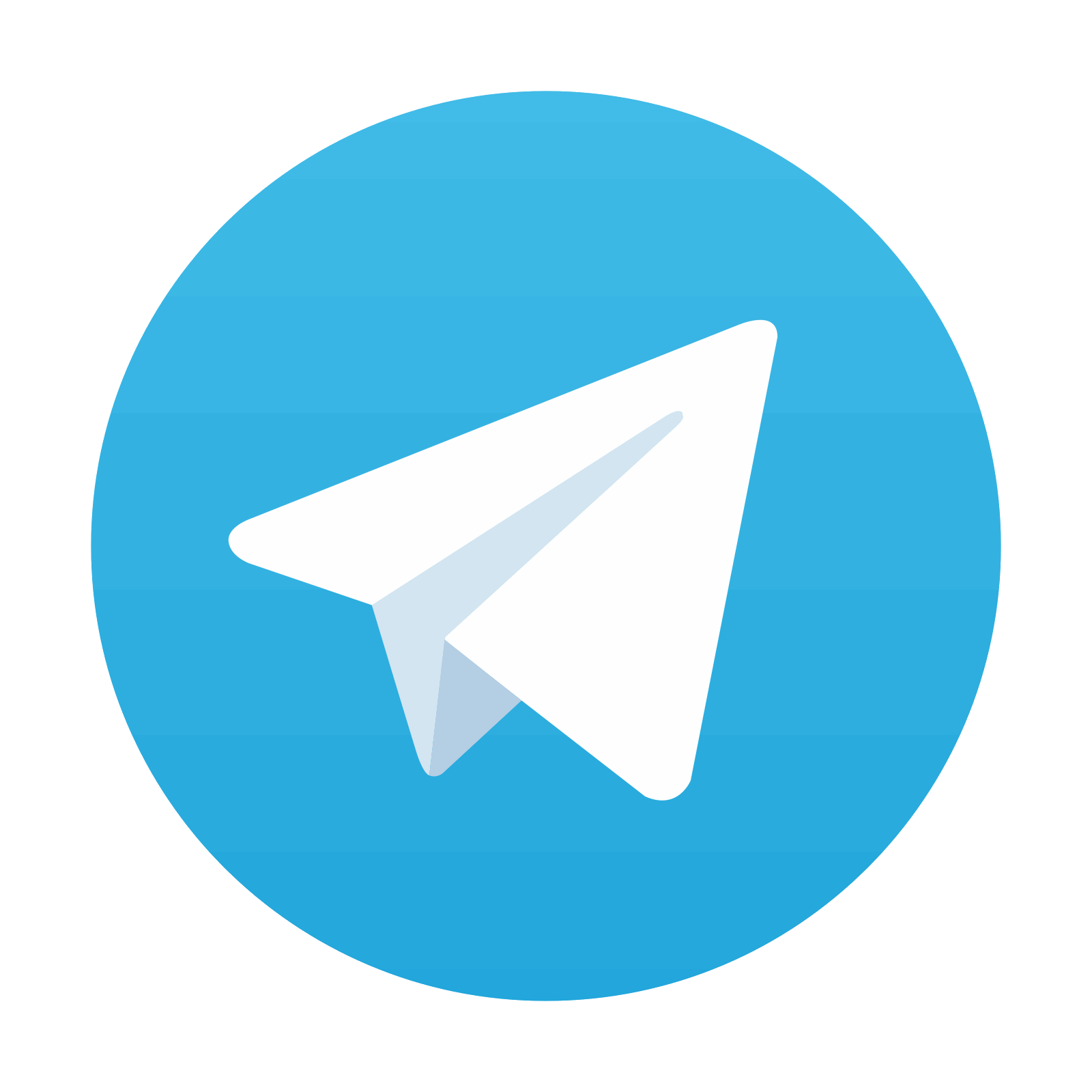
Stay updated, free articles. Join our Telegram channel

Full access? Get Clinical Tree
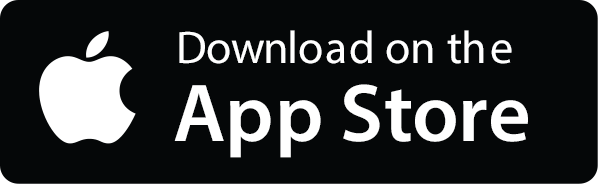
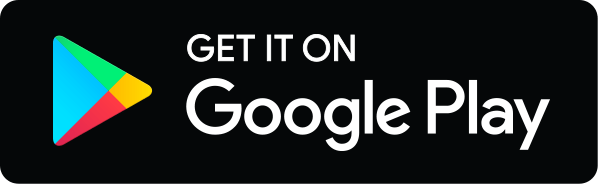