6 T.K. Lau Fetal Medicine Centre, Paramount Medical Centre, Hong Kong A large part of normal obstetric care consists of a series of screening tests designed to identify those pregnant women who are at risk of various maternal, obstetric or fetal complications so that early interventions can be initiated so as to minimize maternal and fetal mortality and morbidities. This series of ‘screening’ tests includes not just blood tests and ultrasound scans but also history‐taking and physical examination. Many of these screening tests have been discussed in other chapters in this book under specific conditions and therefore will not be repeated here. This chapter focuses on the antenatal screening of chromosomal abnormalities. In deciding what diseases to screen for, one should balance the potential benefits and harms that a screening test may result in people who are otherwise well. The most widely used set of principles at present is the one described by Wilson and Jungner in a 1968 report commissioned by the World Health Organization (WHO). These principles are as follows. A screening test will categorize the testing population into a high‐risk (or test‐positive) group and a low‐risk (or test‐negative) group. Further investigations and diagnostic tests should be considered for those in the high‐risk group. There are four important parameters in assessing the performance of a screening test, namely the sensitivity, specificity, positive predictive value (PPV) and negative predictive value (NPV). Sensitivity, or detection rate or true positive rate, refers to the proportion of affected subjects that are correctly identified by the screening test, while specificity refers to the proportion of negatives that are correctly identified as such. Specificity, or true negative rate, equals (1 – false‐positive rate). Sensitivity and specificity are determined by the screening test itself. A high sensitivity indicates a lower chance of missing an affected subject, while a high specificity will lead to less false alarms and therefore less harm. PPV refers to the proportion of screened high‐risk subjects who are indeed affected by the condition or disease, while NPV refers to the proportion of screened low‐risk subjects who are indeed free of the condition. Both PPV and NPV are not only affected by the sensitivity and specificity of the test but also by the prevalence of the condition in the testing population. For example, the PPV for a screening test with a sensitivity of 95% and a specificity of 95% will be 1.87% (1 in 53), 16.1% (1 in 6) and 67.9% (1 in 1.5) when the disease prevalence in the testing population is 0.1, 1 and 10%, respectively. Screening for fetal Down’s syndrome has become part of routine antenatal care in many countries. Down’s syndrome has been considered the commonest genetic cause of intellectual disability. Without screening, the incidence is about 1 in 600 to 1 in 800 live births. The incidence of Down’s syndrome increases with maternal age, being relatively stable before age 30 but increasing exponentially after age 35. About 30% of affected pregnancies result in miscarriage or intrauterine fetal death and therefore the incidence of Down’s syndrome decreases with advancing gestational age. In over 95% of individuals with Down’s syndrome the condition is due to trisomy 21 – the presence of one extra chromosome 21 – which is typically due to an error, namely non‐disjunction, during cell division. About 95% of the non‐disjunctions are meiotic errors, of which over 90% are maternal in origin. The remaining 5% of non‐disjunctions are due to mitotic errors. Although trisomy 21 is not an inherited condition, a history of an affected pregnancy increases the chance of having another affected pregnancy by about 0.75%. Previous trisomy 21 not only increases the risk of trisomy 21 (relative risk or RR, 2.2) but also other different trisomies such as trisomy 13 or 18 (RR 1.4) in subsequent pregnancies [1]. This suggests that some individuals are more prone to non‐disjunction during meiosis, or some trisomies are due to underlying maternal gonadal mosaicism. About 2–3% of Down’s syndrome cases are due to Robertsonian translocations, in which part of chromosome 21 is translocated to another chromosome, most commonly chromosome 14. About two‐thirds of these cases are de novo events, while one‐third are inherited from one of the parents who is a carrier of a balanced translocation. If the mother is the carrier, there is about a 10–15% chance of recurrence in future pregnancies. If the father is the carrier, the risk is much lower, estimated to be at most 3% although some believe that the additional risk is negligible. If an individual is a carrier of a very special form of balanced Robertsonian translocation involving two chromosomes 21, there will be 100% risk of having pregnancies with Down’s syndrome. About 2–3% of Down’s syndrome are mosaic. The phenotype may be milder, especially among those with low percentage of the abnormal cell line. All individuals with Down’s syndrome have similar facial characteristics and some degree of intellectual disability, most common in the moderate degree range. About 40% are in the mild range with IQ between 50 and 70. Other medical problems are common, including hearing loss (up to 70%), obstructive sleep apnoea (50%), congenital heart diseases (50%), hypothyroidism, otitis media, cataracts and visual problems. They are at higher risk of developing leukaemia and Alzheimer’s disease. In the past, the outcome of individuals with Down’s syndrome was poor, mostly due to the lack of medical care and support. In the early 1900s, affected individuals seldom survived to their teenage years. However, the prognosis of individuals with Down’s syndrome has improved significantly over the last century. Currently in developed countries the average lifespan of affected individuals is already over 50, and many of them are living with their families or independently with varying degree of support. Such improvement relies on the availability of appropriate and adequate medical care, early interventional programmes, education, financial support, social support and employment opportunities. Such interventions not only are important determining factors for the prognosis of affected individuals, but also affect the well‐being of the family as a whole. In developed countries with adequate medical and social support, most families with children with Down’s syndrome are able to find enough resources to help them in coping with the additional demands and challenges, resulting in an ordinary family life in themselves. However, about 30–40% of families still have significant stress and distress. Even with all necessary social and medical support, Down’s syndrome remains a condition with multiple medical problems that poses significant stress and challenges to the family. At present, it is medically possible to identify affected pregnancy in the antenatal period, and pregnancy termination of affected pregnancies is legal in many countries. Almost all published studies have confirmed that prenatal Down’s syndrome screening is cost‐effective. On the other hand, Down’s syndrome is a non‐lethal condition, and there is continuous debate about the ethics of terminating affected pregnancies. It is beyond the scope of this chapter to elaborate on the ethics of Down’s syndrome screening. The discussion will therefore focus on the scientific basis and details of implementation of Down’s syndrome screening in the first trimester of pregnancy. Not all women want to undergo screening so any programme must recognize those wishing to ‘opt out’. In the UK this group comprises 10–40% of the pregnant population, depending on local demographics. In this case these women can have an early scan but the fetal nuchal translucency (NT) is not measured. First‐trimester combined screening test, or first‐trimester combined screening (FTCS), is the commonest form of first‐trimester screening, in which the risk of Down’s syndrome is estimated based on the measurement of fetal NT and maternal serum markers, including pregnancy‐associated plasma protein A (PAPP‐A) and free β‐human chorionic gonadotrophins (fb‐hCG). The typical changes associated with fetal Down’s syndrome are increased NT and maternal fb‐hCG, and a reduction in maternal PAPP‐A. Most first‐trimester combined screening programmes are performed between 11 and 13+6 weeks of gestation, or at a crown–rump length of 45–84 mm. Down’s syndrome screening is a risk estimation process. This starts with the estimation of the a priori risk or pre‐test odds of Down’s syndrome of a test subject based on her age, gestational age and history of affected pregnancies. Then, multiple markers are measured. The deviation of each marker from the expected value of a normal pregnancy is calculated, based on which a likelihood ratio (LR) is estimated. The LR tells how much more likely a pregnancy is affected by Down’s syndrome given the value of the measurement. LR of 1 means that there is no change in risk. LR above 1 denotes an increased risk, while LR below 1 denotes a reduced risk. An individualized risk or post‐test odds is calculated based on the following formula: Most Down’s syndrome screening algorithms use a similar approach, although more complicated mathematical modelling may be involved to take into account the interactions between different markers. A cut‐off value of the individualized risk is chosen to categorize a test subject as high or low risk. There are many ways to determine the cut‐off value. Moving the cut‐off will change the sensitivity and specificity at the same time. For example, assuming that the sensitivity and specificity are 75% and 95%, respectively, at a cut‐off of 1 in 250, moving the cut‐off to 1 in 400 may increase the sensitivity to 85% but also reduce the specificity to 80%. Moving the cut‐off to 1 in 100 may reduce the sensitivity to 65% but also increase the specificity to 98%. These numbers are only hypothetical, and the true effect in a screening programme can be analysed by the ROC (receiver operating characteristic) curve. The choice of cut‐off is therefore a compromise between high sensitivity and high specificity. It is important to realize that using the same screening test with the same cut‐off in populations with different maternal age may result in different sensitivities and specificities. For example, using a fixed cut‐off, the same screening test will result in higher sensitivity and lower specificity among those aged 35 or above compared to those aged 25 or below. Some argue that there should not be a recommended cut‐off, and screened subjects should simply make their own judgement based on their own individualized risk to determine if further diagnostic tests are necessary. However, most screened subjects would probably be less confused with a suggested cut‐off and a simple result of high or low risk. NT is one of the best single markers of fetal Down’s syndrome. NT is due to a thin layer of fluid beneath the fetal nuchal skin. It is present in all fetuses in the late first and early second trimester, and then gradually disappears. NT must be differentiated from nuchal fold thickness, which is a mid‐second trimester marker measuring the fetal nuchal skin but not fluid collection. When properly performed, NT alone enables the detection of 70–80% of pregnancies affected by Down’s syndrome at a 5% false‐positive rate (FPR). Increased NT is also detected in about 70–75% of fetuses with trisomy 13 and trisomy 18, and 80–90% of those with Turner’s syndrome. Overall, about 5–8% of those with increased NT will have chromosomal abnormalities. Risk assessment by NT should only be undertaken by those who have been trained and accredited, with continuous auditing of performance and recertification. The most commonly used protocol is listed in Table 6.1, which should be followed strictly. Unlike maternal biochemistry, NT is not significantly affected by maternal characteristics. Table 6.1 The standard protocol for proper first‐trimester nuchal translucency (NT) measurement used in prenatal Down’s syndrome screening. Risk assessment by maternal serum biochemistry using PAPP‐A and fb‐hCG alone has a performance comparable to that of screening using NT alone, with a sensitivity of about 70% at 5% FPR. Maternal serum markers are significantly affected by maternal characteristics, including ethnicity, maternal weight, medical disease such as diabetes and the mode of conception. Most risk calculation algorithms provide adjustment for these factors, to maximize the test performance. All laboratories performing Down’s syndrome screening biochemistry should have continuous internal and external quality assurance programmes (such as the United Kingdom National External Quality Assessment Service, UKNEQAS) in place to ensure the stability of their assay. It is important for all clinicians involved in prenatal screenings to ensure that the biochemical laboratories they are using are well qualified and have satisfactory quality control. Combining NT, PAPP‐A and fb‐hCG as the FTCS, the sensitivity is about 90% at 5% FPR. Screening centres should participate in appropriate quality assurance programmes. The common variations include the following. In addition to thickened NT, other sonographic markers, including absence of nasal bone (NB), tricuspid regurgitation (TR), abnormal waveform of ductus venosus (DV), increased frontomaxillary facial angle, the presence of aberrant right subclavian artery and many others, have been found to be associated with fetal Down’s syndrome. Algorithms have been developed to use solely sonographic markers in calculating the individualized risk of Down’s syndrome, commonly incorporating NT, NB, DV, TR and fetal heart rate, achieving a test performance similar to that of the FTCS programme. The advantage of this approach is that the test result is immediately available, and the assessment is fetus‐specific. It is particularly useful in cases of multiple pregnancies, or a vanished twin pregnancy. The disadvantage is that ultrasound is a highly operator‐dependent test, for which quality control is more difficult than for biochemistry‐based tests. Since the throughput of an accredited sonographer is much lower than that of an accredited biochemical laboratory, an ultrasound‐only approach is more difficult to implement in populated‐based screening programmes. Furthermore, this approach has not been tested as vigorously in different ethnic populations as the FTCS. The effect of ethnicity on some of the markers, such as NB, is substantial. FTCS is not feasible if there are insufficient accredited sonographers for NT measurement. Under such circumstances, first‐trimester screening can only be performed based on biochemistry alone. Compared with second‐trimester screening, this offers earlier screening but there is no major additional benefit in terms of test performance. Even with biochemistry alone, a simple dating scan will improve the screening performance by confirming the number of fetuses and accurate dating. Different markers, both sonographic and biochemical, are tested at different gestational ages, typically in both first and second trimesters. Only one individualized risk is calculated when information from all markers is available. The advantage is improved performance, with estimated sensitivity above 90% and specificity above 98%. However, this approach involves more visits, more complicated logistic and administrative arrangements, higher chance of dropout, and high chance of deviation from protocol based on results from the first part of the study. All women who are screened positive should be counselled and referred for a confirmatory diagnostic test. The final decision whether to have a diagnostic test or not is wholly a decision of the couple, which is a balance between the risk of losing a normal baby due to the diagnostic test and the chance of having an affected child. This balance could be difficult for many couples and is influenced by many factors, including (i) their reproductive history, (ii) how high the individualized risk is, (iii) how the result of the diagnostic test will affect their decision and well‐being, (iv) the risk of the diagnostic test, (v) the type of diagnostic test and (vi) the reporting time. There are two diagnostic tests to choose from, amniocentesis and chorionic villous sampling.
First Trimester Antenatal Screening
First‐trimester Down’s syndrome screening
Overview
Uptake of screening and ethical issues
First‐trimester combined screening programmes
The measurement must be taken at a gestation between 11 weeks and 13 weeks 6 days
The measurement must be taken when the fetal crown–rump length is between 45 and 84 mm
The image must be taken in a good sagittal section, with the baby lying horizontally
The image must be magnified to include the fetal head and upper thorax only
The measurement should be taken when the neck is in a neutral position
The NT must be clearly shown
The calliper is placed on the line that defines the NT thickness: the crossbar of the calliper should be such that it is hardly visible as it merges with the white line of the border, not in the nuchal fluid
The measurement should be taken where the NT is thickest
The NT should be measured three times and the largest one is taken as the NT of the fetus
The amnion should not be confused with the skin, which will overestimate the NT
Variations of first‐trimester combined screening
Approach using ultrasound markers alone
Biochemistry‐based approach
Integrated or sequential screening
Special conditions
Common questions and misconceptions about first‐trimester screening of Down’s syndrome
Screen‐positive results
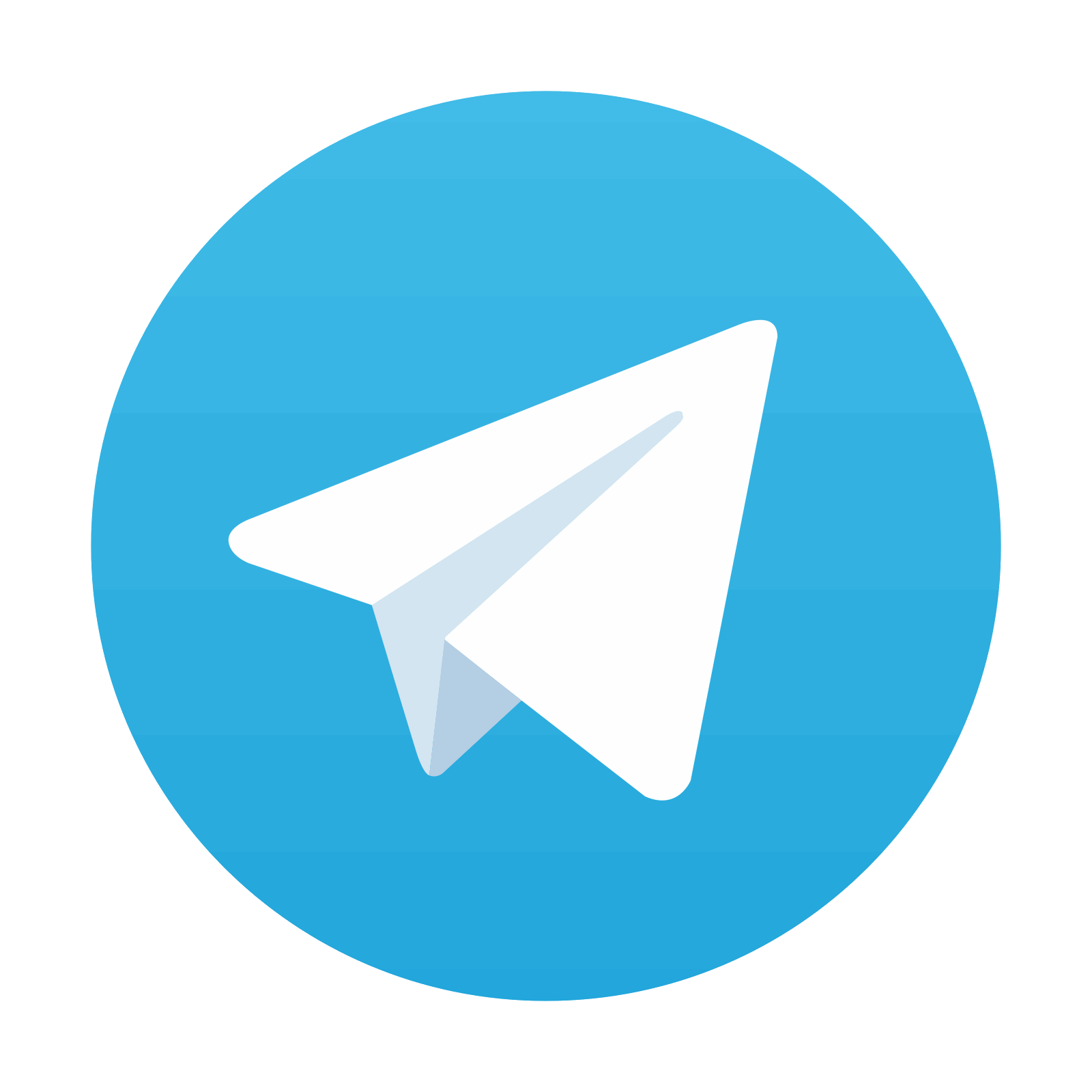
Stay updated, free articles. Join our Telegram channel

Full access? Get Clinical Tree
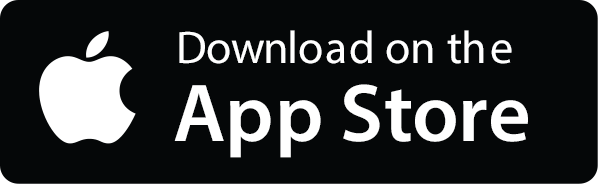
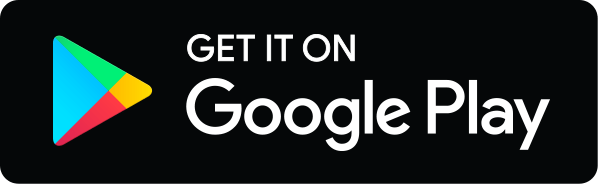