27Fetal Monitoring During Labour
Sara Paterson‐Brown1 and Tracey A. Johnston2
1 Queen Charlotte’s Hospital Imperial NHS Trust, London, UK
2 Birmingham Women’s and Children’s NHS Foundation Trust, Birmingham, UK
What this chapter cannot do is provide a comprehensive manual of fetal physiology, fetal heart rate interpretation and fetal monitoring techniques. While the main principles of these are discussed here, the reader is referred to other learning tools for fetal monitoring, including standard textbooks, the Royal College of Obstetricians and Gynaecologists (RCOG) e‐learning program [1] and the online K2 fetal monitoring training system [2]. These e‐learning systems are divided into sections which cover maternal and fetal physiology and pathophysiology, fetal heart rate and cardiotocography, and include a wealth of cases that can be worked through, asking the reader to interpret and make decisions which can be compared with those of experts.
Fetal physiology
Normal
The fetus is sustained by the uteroplacental unit and under normal circumstances provision is surplus to requirements, enabling the fetus to thrive, grow and build up energy reserves. Aerobic metabolism of glucose provides the fetus with its energy, and the oxygen which is vital for this process is usually plentiful. In normal pregnancy there is therefore more oxygen supplied by the uteroplacental unit than needed by the fetus and energy reserves in the form of glycogen are stored by the fetus.
Fetal compromise
The fetus can be compromised by a reduction in oxygen supply, an increase in oxygen requirements (e.g. in sepsis) or a reduced capacity to transport oxygen (severe fetal anaemia, e.g. rhesus disease, fetomaternal haemorrhage or some intrauterine infections such as parvovirus). The severity and duration of the insult will vary according to the cause, and similarly the effects on the fetus are equally wide‐ranging, but the principal fetal responses are described below.
- Acute. If oxygen delivery falls, there is reduced oxygen in the fetal circulation (hypoxaemia); if levels fall further, hypoxia occurs (reduced oxygen in the fetal tissues). Two things occur in response to hypoxaemia and hypoxia: the circulation adapts to maintain supplies to vital organs (brain and heart) and anaerobic metabolism utilizing glucose released from the stored glycogen reserves provides continued energy supplies. The main consequences of anaerobic metabolism, which is almost 20 times less efficient than aerobic metabolism, are that fetal reserves are used up relatively quickly and the lactic acid, which is the waste product of this metabolism, results in a metabolic acidosis that can be harmful.
- Chronic. If there is uteroplacental disease and fetal supplies are limited, then glycogen stores may not accumulate and fetal reserves will be lacking. In chronic cases of reduced supply, the fetal circulation adapts as mentioned above with cranial sparing, and the peripheral circulation is effectively reduced. Depending on the severity and duration of this compromise, the pathophysiology results in decreased renal perfusion which in turn produces oligohydramnios (and, when more severe, anhydramnios) and reduced fetal growth. If there is further deterioration, anaerobic metabolism occurs and a metabolic acidosis develops.
- Acute on chronic. If an acute insult occurs in a fetus already compromised, it can withstand less hypoxia than a well‐nourished fetus because it has less reserve and may have already made adaptations to conserve vital cerebral perfusion.
Thus the response and vulnerability of any fetus to a hypoxic insult will be largely dictated by (i) the condition of the fetus at the onset of that insult, (ii) the severity of that insult, and (iii) the duration of that insult. The fetal condition can deteriorate extremely quickly and defining the period of time beyond which damage will be sustained is impossible due to the individual circumstances of each fetus and each insult, but a normal well‐grown and mature fetus that has good reserves can tolerate a short period of severe hypoxia, whereas the same insult can be catastrophic for a growth‐restricted fetus.
Metabolic acidaemia
Paired umbilical cord blood samples at delivery enable direct measurements of uteroplacental function (umbilical vein) and fetal acid–base status (umbilical artery). There are now a number of published series of routine paired cord samples [2–5] and results largely conform and are approximated in Table 27.1. The base deficit in the umbilical artery indicates the degree of fetal metabolic acidosis and values above 12 mmol/L suggest that there has been significant hypoxia and subsequent fetal damage may occur [6]. If venous pH and base deficit are normal, this rules out chronic hypoxia. The mean pH difference between artery and vein is 0.08 and if this difference is less than 0.03, it is likely that both samples have been taken from the umbilical vein. Timing of cord clamping after delivery can also influence cord samples [7].
Table 27.1 Routine paired umbilical cord values.
pH (median) | pH (2.5–97.5 centile) | Median base deficit (mmol/L) | Base deficit (mmol/L) (2.5–97.5 centile) | |
Umbilical vein | 7.35 | 7.17–7.48 | 3.7 | 9.0–0.5 |
Umbilical artery | 7.25 | 7.05–7.38 | 4.3 | 11.1–0.5 |
Fetal and neonatal consequences of hypoxia
The damage that results from hypoxic insults varies according to the vulnerability of the fetus and the nature and duration of the insult, ranging from minimal clinical signs through brain damage to death. While neonatal complications tend to increase as cord arterial pH falls [8], especially at values below 7.0 [9–11], many born with such values do well [9,10,12] while approximately one‐quarter suffer neurological morbidity or mortality [13].
Death
Intrapartum deaths do occur as a result of hypoxia, with an incidence in the UK of approximately 0.22 per 1000 [14], and intrapartum‐related deaths (both stillbirths and neonatal deaths) account for 1 in 12 perinatal deaths [15]. While scrutiny of these cases has identified elements of substandard care, many cases are managed well and yet death still results. Confidential enquiries have shown that approximately half of intrapartum stillbirths are considered to be avoidable [16,17], with errors in care extending from a failure to recognize problems in the antenatal period and/or in labour to recognizing but failing to act on signs of fetal compromise [17].
Neonatal pathology
The paired umbilical cord gas results provide an objective measure of in utero fetal metabolism, reflecting the presence or absence of intrauterine hypoxia, but although they are associated with neonatal outcomes they are not predictive and do not indicate neonatal resuscitative needs. Indeed many babies born with low cord gases are vigorous at birth. On the other hand, the Apgar score is a subjective assessment of the need for neonatal resuscitation but does not indicate the cause of the problem. Resuscitation therefore occurs according to clinical need and the possible cause may then be clarified by the cord gases. A variety of neonatal problems can follow on from intrauterine hypoxia, including hypoglycaemia, disturbed thermoregulation and necrotizing enterocolitis, but the main worry is that of hypoxic brain injury due to the long‐term morbidity associated with it.
Hypoxic–ischaemic encephalopathy
Neonatal encephalopathy is associated with a multitude of causes but hypoxic–ischaemic encephalopathy (HIE) is neonatal encephalopathy specifically caused by peripartum hypoxia. The centiles listed in Table 27.1 demonstrate that 2.5% of neonates are born with a cord arterial pH below 7.05. The incidence of more severe acidaemia (cord arterial pH <7.0) is approximately 3.7 per 1000 live births, while that of HIE at term is approximately 2.5 per 1000 live births [13]. The diagnosis of HIE requires evidence of intrapartum hypoxia and although there are various definitions [18–20], in essence there needs to be evidence of all of the following:
- an intrapartum insult (e.g. a sentinel event in labour or a pathological fetal heart recording);
- a metabolic acidosis at birth (pH <7.0 and base excess >12 mmol/L);
- moderate or severe neonatal encephalopathy;
- specific features on imaging in the neonatal period.
A spectrum of permanent brain injury can occur as a result of HIE [21] ranging from spastic quadriplegic or dyskinetic cerebral palsy [18,20] to learning difficulties without motor impairment [22]. The incidence of cerebral palsy is 1–2 per 1000 deliveries and although the majority of these are due to antenatal complications, at least 10–15% result from events in labour [13].
Treatment and outcome of HIE
Severe acidaemia at birth (pH <7.0) does not always result in HIE, which in turn does not automatically result in cerebral palsy, but the more severe the metabolic acidosis at delivery and the more severe the HIE, the worse the prognosis for the baby. Individual clinical features and responses of the baby help inform the likely outcome as does cerebral imaging.
The greatest advance in this field is that of controlled total body cooling of term neonates with HIE. The cooling needs to start within 6 hours of birth and has been shown to improve intact neonatal survival (relative risk of cerebral palsy 0.67, 95% CI 0.47–0.96) with number needed to treat of 8 (95% CI 5–17) [23,24]. The obstetrician’s role in this process is one of early communication and frank discussion with neonatal colleagues to help clarify the obstetric history and the likely diagnosis, which should help to promote early cooling or referral to a centre that provides this treatment if it is not available locally (see Chapter 32).
Labour
The rest of this chapter focuses on the normal physiology of labour and the fetal responses to labour, before highlighting abnormal labour patterns that increase fetal challenges and the problems which can arise from them. Without knowledge of the physiology and pathophysiology of labour, the anticipation and recognition of early signs of hypoxia may be missed. Failure to recognize the fetus at risk on entry to labour or failure to look for and then recognize early signs of hypoxia in labour can compromise the opportunity for prompt and timely action that may be crucial in avoiding irreversible damage.
The challenges of normal labour
Uterine contractions
In normal labour, the fetus is ‘stressed’ due to uterine contractions. The uteroplacental circulation is a low‐pressure system and its perfusion ceases at the height of a uterine contraction in the first stage of labour (where intrauterine pressures reach about 90 mmHg) and throughout practically all of a contraction when the woman is pushing in the second stage of labour (intrauterine pressures up to 250 mmHg). Oxygen delivery to the fetus therefore effectively ceases during these periods.
A fetus in a normal pregnancy has adequate reserves of oxygen and can cope with this brief, temporary insult as long as the uterus relaxes enough between contractions for gas transfer to occur thus replenishing the supply of oxygen. The interval between the contractions is crucial to allow this recovery (Fig. 27.1), with 60–90 seconds of uterine relaxation needed between contractions [1].

Fig. 27.1 Number of uterine contractions versus their duration in each 10‐min period and how this influences the period of fetal recovery during uterine relaxation (r), both similar at approximately 4 min.
A less well‐nourished fetus does not have the same oxygen reserves even when the uterus is relaxed, and may have reduced or minimal energy reserves, so may not manage the episodic oxygen deprivation associated with each uterine contraction.
Head compression
The fetal head is subjected to pressure as it is compressed onto the cervix and through the pelvis by uterine contractions, and latterly this is exacerbated by maternal expulsive efforts. Head compression can produce a reflex bradycardia due to changes in intracranial pressure. This is a normal autonomic response, but clearly during any bradycardic episode the fetal circulation is reduced, so once again a baby already compromised will be more affected by this temporary reduction in oxygen delivery to the tissues due to the slower heart rate.
Cord compression
The umbilical cord lies in random fashion around the fetus in utero and can wrap around or lie in close proximity to any part of the fetus so that during a contraction it can become squashed. This is increasingly likely once the membranes have ruptured. The normal autonomic responses of a healthy fetus to an episode of cord compression is the result of baroreceptor and chemoreceptor stimulation and proceed as follows.
- Initially, the larger, less muscular, umbilical vein obstructs resulting in decreased cardiac return, which stimulates baroreceptors that cause a tachycardia.
- Hypoxia secondary to the umbilical vein obstruction stimulates chemoreceptors to trigger a parasympathetic response which produces a bradycardia.
- With further compression, umbilical artery obstruction raises peripheral resistance, exacerbating the bradycardia.
- As the contractions wear off, the process is reversed.
Cord compression, which is common, therefore produces a fairly classic pattern of fetal heart rate (FHR) decelerations as a normal autonomic response in a healthy fetus (Fig. 27.2, pattern ‘a’). However, as mentioned above, every time a deceleration occurs the fetal circulation is reduced and therefore adequate uterine relaxation between contractions is vital to allow recovery of the fetal circulation with subsequent tissue perfusion. Prolonged or repetitive decelerations can have a cumulative effect over time: firstly the shouldering disappears, then there is a rebound tachycardia, delayed recovery from the decelerations and a rising baseline (Fig. 27.2). In the most severe type the baseline is undefined because the next contraction occurs before it is reached and the fetal heart decelerates again. Moreover, cord compression is more common when there is reduced Wharton’s jelly to protect the umbilical vessels, as with a premature or growth‐restricted fetus, and these are therefore more likely to suffer cord compression as well as being already compromised.

Fig. 27.2 Variable decelerations associated with cord compression demonstrating a typical dip (a). A rising baseline (b), a rebound tachycardia (t) and delayed recovery (r) are all suspicious signs.
The challenges of abnormal labour
Infection
Pyrexia in labour is associated with an increased risk of fetal hypoxic damage and cerebral palsy [25,26]. The two processes of hypoxia and sepsis seem to potentiate each other rather than being simply additive, and this process can escalate quickly needing early recognition and appropriate action. One problem is that epidural anaesthesia is associated with a rise in core temperature and therefore mild pyrexias in labour are relatively common. This risks causing a rather relaxed attitude to fevers in labour rather than raising alarm bells. Clinical vigilance is needed, with proper assessment and appropriate treatment.
- If there are clinical signs of infection, even a mild pyrexia is significant.
- If there is no regional blockade, pyrexia of 37.5 °C is significant.
- Pyrexia of 38 °C with regional blockade is significant.
- Paracetamol alone is not a treatment for infection: it may lower the temperature by nature of its antipyretic effect and this is useful, but it does not mean that the infection is treated. ‘Hiding’ one of the signs of infection in this way can be dangerous.
In all these situations appropriate microbiology cultures should be taken and antibiotic treatment started. Clinical awareness of the infection and what it might mean to fetal condition, fetal reserve and the likely tolerance of the fetus to the remaining labour will all play a part in timing the delivery.
Prolonged labour
A fetus has to tolerate more uterine contractions during prolonged labour. This is common with fetal malpositions and, as such, is also associated with induced labour for post‐term pregnancies or prolonged rupture of membranes, both of which increase the risks to the fetus (added risk of uteroplacental insufficiency or infection, respectively).
Excessive uterine activity
This comprises either tachysystole (more than five contractions in 10 min) or hypertonus (prolonged contraction or increased basal tone) and both can occur spontaneously or be iatrogenic. When excessive uterine activity occurs spontaneously, it can be extremely concerning because it is likely to be associated with pathology such as infection (additional risks and vulnerability already discussed) or bleeding. In the case of bleeding (which may be concealed retroplacental separation) there is the added insult of placental separation as well as failure of adequate uterine relaxation for the fetus to contend with. This situation is very dangerous and fetal deterioration is likely to be rapid.
Conversely, iatrogenic tachysystole and hypertonus are reversible if recognized and acted upon. These can result from prostaglandin sensitivity or overdose as well as oxytocin overdose. A common obstetric and midwifery aim when stimulating labour with oxytocin is for a certain number of contractions every 10 min but as this focuses attention on the contraction and not the relaxation, it is easy to see why excessive uterine activity is a relatively common problem [27–29]. If contractions last for 1 min, then aiming for four contractions in 10 min is reasonable, but if they last 2 min squeezing four into 10 min will cause fetal hypoxia (see Fig. 27.1). The added problem here is that oxytocin tends to be used in abnormal labour (e.g. prolonged rupture of membranes, post‐term inductions, prolonged labour) so there are many other risk factors for fetal compromise. Tachysystole often goes unrecognized, with the Syntocinon infusion continuing or even being increased further until FHR abnormalities and thus hyperstimulation (tachysystole with FHR abnormalities) develops.
Uterine rupture
This results in catastrophic interruption to fetal oxygenation and delivery needs to be effected within minutes. However, even with this dramatic pathology, signs other than abnormal FHR monitoring may be subtle and the diagnosis is often not made until laparotomy [30]. Keeping the possibility in mind during problems with progress in labour or in those at increased risk may help to anticipate the diagnosis and lead to rapid action if needed.
Placental abruption
This may also be missed clinically as bleeding may be concealed and pain can be difficult to distinguish from labour, especially with a posterior placenta. Tachysystole and hypertonus are signs that are often missed because the focus of attention tends to be on the fetal heart and not the clinical signs and the tocograph. Therefore, again, the relatively later sign of an abnormal FHR pattern is often the only indication that there is something wrong.
Cord prolapse
Cord prolapse can also cause severe catastrophic cessation of fetal oxygenation requiring urgent delivery and is usually confirmed on vaginal examination after fetal heart abnormalities have been detected.
Cord compression becoming cord occlusion
Whilst an element of cord compression is relatively common in labour (and often self‐corrects with alteration in maternal position, or rotation and descent of the fetal parts) it can deteriorate and lead to prolonged cord occlusion. This is a particular risk in the second stage of labour when the woman commences pushing and the head descends. In the presence of an FHR pattern in keeping with cord compression, it is therefore sensible to have made preparations for rapid delivery should the need arise, before commencing pushing.
Detecting the fetus with hypoxia
Recognizing fetal risk
As the ability of any fetus to cope with labour depends on its condition on entry into labour, antenatal recognition of risk is vital in planning safe delivery. Any woman identified as high risk for fetal compromise in labour can then be cared for accordingly, while those of low risk can be monitored less intensively. Whichever technique is used, the aim of intrapartum monitoring of the fetus is to ‘screen’ for early signs of hypoxia in order to allow timely intervention and safe delivery before irreversible damage has been sustained. The priority with a low‐risk pregnancy would be to have a detection system with a low false‐positive rate so that unnecessary intervention is kept to a minimum; conversely, the priority for an at‐risk fetus would be to have a detection system with a low false‐negative rate and good sensitivity to identify the problem early.
The different techniques available for monitoring the fetus in labour are discussed in the following sections but it is worth pointing out that inevitably not all high‐risk cases are detected antenatally and the unknown ‘high‐risk’ fetus is especially vulnerable in labour because the index of suspicion is reduced. It is therefore important that with every woman and fetus admitted in labour, there is a deliberate effort to re‐screen using history and examination to confirm normality before proceeding with the planned birth.
Liquor and the passage of meconium
A good liquor volume is a reassuring sign that the fetus has not been subjected to chronic hypoxia in the antenatal period (discussed above). If no liquor is seen in labour after amniotomy (spontaneous or artificial), the safe assumption must be that there is oligohydramnios/anhydramnios and the case in question is an unrecognized growth‐restricted fetus. All practising midwives and obstetricians will have seen the thick ‘green‐pea soup’ meconium following the delivery of a baby who had not previously been recognized as being compromised. The clinical secret is to think about this possibility and be clear that absence of liquor indicates oligohydramnios/anhydramnios/growth restriction until proved otherwise (e.g. in the labour ward setting this can be assessed by an ultrasound scan).
The other sign in labour is that of the colour of the liquor. The National Institute for Health and Care Excellence (NICE) guidelines on intrapartum care discusses whether the presence of particulate meconium is significant or not [31,32], but working from pathophysiological principles seems a more logical way to interpret the signs.
- A term fetus may have passed meconium by nature of its maturity, but if this is ‘innocent’ the meconium should be diluted by adequate liquor.
- If the fetus is preterm, the passage of meconium is not normal and may suggest infection or hypoxia.
- If the meconium is thick, then by definition the liquor volume is reduced and reflects uteroplacental insufficiency and possible fetal compromise.
- If the liquor has been clear and then becomes meconium stained during labour, it suggests that the fetus may be compromised and this could be due to either hypoxia or infection.
Fetal monitoring in labour
Searching for signs and understanding the condition of the fetus and the normal stresses or pathophysiological insults it is exposed to is the single most important part of fetal monitoring in labour. Omitting this fundamental process on admission in labour and proceeding directly to FHR monitoring assumes that all babies have the same reserves and vulnerabilities. Our earlier discussions demonstrate how wrong such assumptions are: having an informed index of suspicion for a particular fetus and an understanding of any likely pathophysiology from the antenatal period and during labour increases the chance that signs of compromise will be detected early. Waiting until events are obvious is unthinking and dangerous as it may be too late to correct. To expect any monitoring system to dictate clinical decisions is unrealistic, illogical and hazardous.
The other point worth making before exploring the different techniques for monitoring the fetus in labour is that of the margin of error between the extremes of failing to recognize a fetus in difficulty (which will therefore sustain damage/death) and mistakenly identifying a healthy fetus as being in difficulty and subjecting it and the mother to an unnecessary operative delivery. When reviewing the different techniques of monitoring it is interesting to note that the trade‐off is usually between increased operative delivery versus increased neonatal morbidity, and the relative risks of these will depend on the background prevalences of pathology; hence the recommendation from NICE that low‐risk women be monitored differently to high‐risk women [31,32]. This does not remove an obligation to discuss the advantages and disadvantages with the pregnant woman in the antenatal period, so that she is informed and involved in the decisions surrounding her care in labour, particularly the fetal monitoring plan. The long‐term outcomes of cerebral palsy and death are rare, and statistically significant differences have yet to be shown from the different monitoring modalities in low‐risk pregnancies, although neonatal seizures are a significant concern and should not be ignored.
Historically, listening to the fetal heart has been the main method of monitoring the fetus in labour, but this is a step removed from the important information, which is whether oxygen supply is adequate for fetal tissue metabolism. If not and anaerobic metabolism has commenced, then by definition fetal hypoxia exists and is likely to get worse. The problem is that there is no direct continuous measure of fetal tissue pH, and even if there were it would be invasive and as such likely to be impractical for routine monitoring. Periodic fetal blood sampling (FBS) can be done in those showing signs of possible compromise, and to date this has been used when FHR pattern abnormalities have been found (discussed in more detail later). Fetal pulse oximetry has been explored as a means of measuring fetal oxygen saturation and is used in combination with FHR monitoring but randomized trials have failed to provide convincing evidence that it is helpful [33–35]. Near‐infrared spectroscopy has also been explored [36,37] but has not yet been subjected to randomized clinical trials [38].
Fetal heart rate
FHR monitoring is the main technique used to supplement clinical scrutiny in intrapartum fetal monitoring. FHR is influenced by numerous factors that act largely through the two opposing divisions of the autonomic nervous system: the sympathetic nervous system, stimulation of which speeds up the rate, and the parasympathetic nervous system, stimulation of which slows it down. As such the heart rate is in a constant state of variation as these two influences oppose each other to maintain homeostasis. Both are responsive to the many stresses of labour, including changes in oxygen levels (via chemoreceptors), changes in fetal circulation (via baroreceptors) and infection, and these stresses are indirectly reflected in the FHR pattern. In addition, myocardial ischaemia also produces a fetal bradycardia. It is for these reasons that the FHR provides a reasonable indicator of fetal condition. This subject is well covered in the online RCOG and K2 teaching programmes [1,2].

Full access? Get Clinical Tree
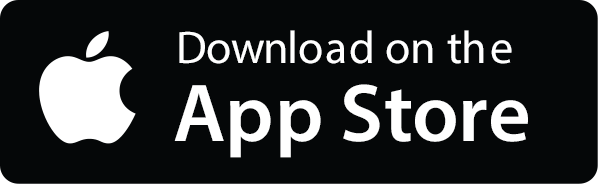
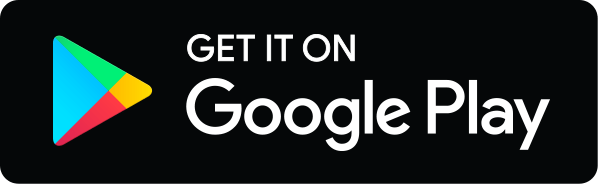