Red Cell Alloimmunization
There are currently 30 different blood group systems and 328 red cell antigens recognized by the International Society of Blood Transfusion (Storry, 2011). Although some of these are immunologically and genetically important, many are so rare as to be of little clinical significance. Any individual who lacks a specific red cell antigen may produce an antibody when exposed to that antigen. Such antibodies can prove harmful to that individual if she receives an incompatible blood transfusion, and they may be harmful to her fetus during pregnancy. Accordingly, blood banks routinely screen for erythrocyte antigens. These antibodies may also be harmful to a mother’s fetus during pregnancy. As noted, maternal antibodies formed against fetal erythrocyte antigens may cross the placenta to cause fetal red cell lysis and anemia.
Typically, a fetus inherits at least one red cell antigen from the father that is lacking in the mother. Thus, the mother may become sensitized if enough fetal erythrocytes reach her circulation to elicit an immune response. Even so, alloimmunization is uncommon for the following reasons: (1) low prevalence of incompatible red cell antigens; (2) insufficient transplacental passage of fetal antigens or maternal antibodies; (3) maternal-fetal ABO incompatibility, which leads to rapid clearance of fetal erythrocytes before they elicit an immune response; (4) variable antigenicity; and (5) variable maternal immune response to the antigen.
In population-based screening studies, the prevalence of red cell alloimmunization in pregnancy is approximately 1 percent (Howard, 1998; Koelewijn, 2008). Most cases of severe fetal anemia requiring antenatal transfusion are attributable to anti-D, anti-Kell, or anti-c alloimmunization.
Alloimmunization Detection
A blood type and antibody screen are routinely assessed at the first prenatal visit, and unbound antibodies in maternal serum are detected by the indirect Coombs test (Chap. 9, p. 174). With positive results, specific antibodies are identified, their immunoglobulin subtype is determined as either IgG or IgM, and the titer is quantified. Only IgG antibodies are of concern because IgM antibodies do not cross the placenta. Selected antibodies and their potential to cause fetal hemolytic anemia are listed in Table 15-1. The critical titer is the level at which significant fetal anemia could potentially develop. This may be different for each antibody, is determined individually by each laboratory, and usually ranges between 1:8 and 1:32. If the critical titer for anti-D antibodies is 1:16, a titer ≥ 1:16 indicates the possibility of severe hemolytic disease. An important exception is Kell sensitization, which is discussed on page 308.
TABLE 15-1. Minor Red Cell Antigens and Their Relationship to Fetal Hemolytic Disease
CDE (Rh) Blood Group Incompatibility
The rhesus system includes five red cell proteins or antigens: C, c, D, E, and e. No “d” antigen has been identified, and Rh D-negativity is defined as the absence of the D antigen. Although most people are Rh D positive or negative, more than 200 D antigen variants exist (Daniels, 2013).
CDE antigens are clinically important. Rh D-negative individuals may become sensitized after a single exposure to as little as 0.1 mL of fetal erythrocytes (Bowman, 1988). The two responsible genes—RHD and RHCE—are located on the short arm of chromosome 1 and are inherited together, independent of other blood group genes. Their incidence varies according to racial and ethnic origin. Nearly 85 percent of non-Hispanic white Americans are Rh D-positive, as are approximately 90 percent of Native Americans, 93 percent of African Americans and Hispanic Americans, and 99 percent of Asian individuals (Garratty, 2004).
The prevalence of Rh D alloimmunization complicating pregnancy ranges from 0.5 to 0.9 percent (Howard, 1998; Koelewijn, 2008; Martin, 2005). Without anti-D immune globulin prophylaxis, an Rh D-negative woman delivered of an Rh D-positive, ABO-compatible infant has a 16-percent likelihood of developing alloimmunization. Two percent will become sensitized by the time of delivery, 7 percent by 6 months postpartum, and the remaining 7 percent will be “sensibilized”—producing detectable antibodies only in a subsequent pregnancy (Bowman, 1985). If there is ABO incompatibility, the Rh D alloimmunization risk is approximately 2 percent without prophylaxis (Bowman, 2006). The reason for the differing rates relative to ABO blood type results from erythrocyte destruction of ABO-incompatible cells and thus limitation of sensitizing opportunities. Rh D sensitization also may occur following first-trimester pregnancy complications, prenatal diagnostic procedures, and maternal trauma (Table 15-2).
TABLE 15-2. Causes of Fetomaternal Hemorrhage Associated with Red Cell Antigen Alloimmunizationa
Pregnancy Loss
Ectopic pregnancy
Spontaneous abortion
Elective abortion
Fetal death (any trimester)
Procedures
Chorionic villus sampling
Amniocentesis
Fetal blood sampling
Other
Delivery
Trauma
Placental abruption
Unexplained vaginal bleeding during pregnancy
External cephalic version
The Rh C, c, E, and e antigens have lower immunogenicity than the Rh D antigen, but they too can cause hemolytic disease. Sensitization to E, c, and C antigens complicates approximately 0.3 percent of pregnancies in screening studies and accounts for about 30 percent of red cell alloimmunization cases (Howard, 1998; Koelewijn, 2008). Anti-E alloimmunization is the most common, but the need for fetal or neonatal transfusions is significantly greater with anti-c alloimmunization than with anti-E or anti-C (Hackney, 2004; Koelewijn, 2008).
The Grandmother Effect. In virtually all pregnancies, small amounts of maternal blood enter the fetal circulation. Real-time polymerase chain reaction (PCR) has been used to identify maternal Rh D-positive DNA in peripheral blood from preterm and full-term Rh D-negative newborns (Lazar, 2006). Thus, it is possible for an Rh D-negative female fetus exposed to maternal Rh D-positive red cells to develop sensitization. When such an individual reaches adulthood, she may produce anti-D antibodies even before or early in her first pregnancy. This mechanism is called the grandmother theory because the fetus in the current pregnancy is jeopardized by maternal antibodies that were initially provoked by his or her grandmother’s erythrocytes.
Alloimmunization to Minor Antigens
Because routine administration of anti-D immunoglobulin prevents anti-D alloimmunization, proportionately more cases of hemolytic disease are caused by red cell antigens other than D—also known as minor antigens (see Table 15-1). Kell antibodies are among the most frequent. Duffy group A antibodies—anti-Fya—are also fairly common, as are anti-MNSs and anti-Jka—Kidd group (Geifman-Holtzman, 1997). Most cases of sensitization to minor antigens result from incompatible blood transfusions (American College of Obstetricians and Gynecologists, 2012). If an IgG red cell antibody is detected and there is any doubt as to its significance, the clinician should err on the side of caution, and the pregnancy should be evaluated for hemolytic disease.
There are only a few blood group antigens that pose no fetal risk. Lewis antibodies—Lea and Leb, as well as I antibodies, are cold agglutinins. They are predominantly IgM and are not expressed on fetal red cells. Another antibody that does not cause fetal hemolysis is Duffy group B—Fyb.
Kell Alloimmunization. Approximately 90 percent of whites and up to 98 percent of African Americans are Kell negative. Kell type is not routinely determined, and approximately 90 percent of Kell sensitization cases result from transfusion with Kell-positive blood. Thus, transfusion history is important.
If Kell sensitization develops from maternal–fetal incompatibility, it may develop more rapidly and may be more severe than with sensitization to Rh D and other blood group antigens. This is because Kell antibodies attach to fetal bone marrow erythrocyte precursors and prevent a hemopoietic response to anemia. With fewer erythrocytes produced, there is less hemolysis. Because of these vicissitudes, severe anemia may not be predicted by either the maternal Kell antibody titer or the amnionic fluid bilirubin level (p. 310). One option is to use a lower critical titer—1:8—for Kell sensitization (Moise, 2012). The American College of Obstetricians and Gynecologists (2012) has recommended that antibody titers not be used to monitor Kell-sensitized pregnancies. Van Wamelen and colleagues (2007) have advocated MCA Doppler studies beginning at 16 to 17 weeks’ gestation for pregnancies with anti-Kell titers ≥ 1:2.
ABO Blood Group Incompatibility
Incompatibility for the major blood group antigens A and B is the most common cause of hemolytic disease in newborn infants, but it does not cause appreciable hemolysis in the fetus. Approximately 20 percent of newborns have ABO blood group incompatibility, however, only 5 percent are clinically affected, and the resulting anemia is usually mild. The condition differs from Rh CDE incompatibility in several respects. First, ABO incompatibility is often seen in firstborn infants, whereas sensitization to other blood group antigens is not. This is because most group O women have developed anti-A and anti-B isoagglutinins before pregnancy from exposure to bacteria displaying similar antigens. Second, ABO alloimmunization can affect future pregnancies, but unlike CDE disease, it rarely becomes progressively more severe. Last, most anti-A and anti-B antibodies are immunoglobulin M (IgM), which do not cross the placenta. Fetal red cells also have fewer A and B antigenic sites than adult cells and are thus less immunogenic. For these reasons, ABO alloimmunization is generally a pediatric disease rather than an obstetrical concern. There is no need to monitor for fetal hemolysis or to deliver the fetus early. Careful neonatal observation is essential, because hyperbilirubinemia may require treatment with phototherapy or occasionally transfusion (Chap. 33, p. 644).
Management of the Alloimmunized Pregnancy
An estimated 25 to 30 percent of fetuses from Rh D-alloimmunized pregnancies will have mild to moderate hemolytic anemia, and without treatment, up to 25 percent will develop hydrops (Tannirandorn, 1990). If alloimmunization is detected and the titer is below the critical value, the titer is generally repeated every 4 weeks for the duration of the pregnancy (American College of Obstetricians and Gynecologists, 2012). Importantly, however, if a woman has had a prior pregnancy complicated by alloimmunization, serial titer assessment is inadequate for surveillance of fetal anemia. In these instances, pregnancy is assumed to be at risk and is followed as discussed on page 310. Once a titer has reached a critical value, there is no benefit to repeating it. The pregnancy is at risk even if the titer decreases, and further evaluation is still required.
Determining Fetal Risk
The presence of maternal anti-D antibodies reflects her sensitization, but it does not necessarily indicate that the fetus will be affected or is even Rh D-positive. For example, in a non-Hispanic white couple in which the woman is Rh D-negative, there is an 85-percent chance that the man is Rh D-positive, but in 60 percent of these cases he will be heterozygous at the D-locus (American College of Obstetricians and Gynecologists, 2012). If he is heterozygous, then only half of his children will be at risk for hemolytic disease. Another consideration is that if a woman became sensitized in a prior pregnancy, her antibody titer might rise to high levels during the current pregnancy even if the current fetus is Rh D-negative. This is termed an amnestic response. Additionally, alloimmunization to a red cell antigen other than Rh D may have occurred following a blood transfusion in the past, and if that antigen is not present on paternal erythrocytes, the pregnancy is not at risk.
Initial evaluation of alloimmunization begins with determining the paternal erythrocyte antigen status. Provided that paternity is certain, if the father is negative for the red cell antigen to which the mother is sensitized, the pregnancy is not at risk. In an Rh D alloimmunized pregnancy in which the father is Rh D-positive, it is helpful to determine prenatal paternal zygosity for the Rh D antigen, and DNA-based analysis will establish this. If the father is heterozygous or if paternity is in question, the patient should be offered assessment of fetal antigen type. In the Unites States, this has traditionally been done with amniocentesis and PCR testing of uncultured amniocytes to assess fetal blood type (Chap. 13, p. 277) (American College of Obstetricians and Gynecologists, 2012). This test has a positive-predictive value of 100 percent and negative-predictive value of approximately 97 percent (Van den Veyver, 1996). Chorionic villus sampling is not generally performed because it is associated with greater risk for fetomaternal hemorrhage and may worsen alloimmunization. Fetal testing for other antigens is also available from reference laboratories using amniocentesis specimens. Examples include testing for E/e, C/c, Duffy, Kell, Kidd, and M/N.
More recently, noninvasive fetal Rh D blood typing has been performed using cell-free fetal DNA from maternal plasma (Chap. 13, p. 279). Accuracy is reported to be as high as 99 to 100 percent (Minon, 2008; Tynan, 2011). In a metaanalysis, only 3 percent of samples had inconclusive results (Geifman-Holtzman, 2006). Fetal Rh D blood typing with cell-free fetal DNA is routinely used in parts of Europe. There are two potential indications in Rh D negative pregnant women: (1) in women with Rh D alloimmunization, testing can identify fetuses who are also Rh D negative and do not require anemia surveillance, and (2) in women without Rh D alloimmunization, anti-D immune globulin might be withheld if the fetus is Rh D negative. However, concerns have been raised that use of the test to limit anti-D immune globulin administration could lead to an increased prevalence of Rh D alloimmunization (Goodspeed, 2013; Szczepura, 2011). As of 2013, cell-free fetal DNA testing for fetal Rh type has not been widely adopted in the United States.
Management of the alloimmunized pregnancy is individualized and may consist of maternal antibody titer surveillance, sonographic monitoring of the fetal MCA peak systolic velocity, amnionic fluid bilirubin studies, or fetal blood sampling. Accurate pregnancy dating is critical. The gestational age at which fetal anemia developed in prior pregnancies is important because anemia tends to occur earlier and be sequentially more severe.
Middle Cerebral Artery Doppler Velocimetry. In most specialized centers, serial measurement of the peak systolic velocity of the fetal middle cerebral artery has replaced amniocentesis for the detection of fetal anemia. The anemic fetus shunts blood preferentially to the brain to maintain adequate oxygenation. The velocity increases because of increased cardiac output and decreased blood viscosity (Moise, 2008a). The technique, which is discussed in Chapter 10 (p. 221), should be used only with adequate training and experience (American College of Obstetricians and Gynecologists, 2012).
In a landmark study, Mari and coworkers (2000) measured the MCA peak systolic velocity serially in 111 fetuses at risk for anemia and in 265 normal control fetuses. Threshold values > 1.5 multiples of the median (MoM) for given gestational ages correctly identified all fetuses with moderate or severe anemia. This provided a sensitivity of 100 percent, with a false-positive rate of 12 percent.
The MCA peak systolic velocity is followed serially, and values are plotted on a curve like the one shown in Figure 15-1. If the velocity is between 1.0 and 1.5 MoM and the slope is increasing—such that the value is approaching 1.5 MoM—surveillance is generally increased to weekly Doppler interrogation. If the MCA peak systolic velocity exceeds 1.5 MoM, further evaluation by fetal blood sampling is necessary to assess need for fetal transfusion. The false-positive rate increases significantly beyond 35 weeks, due to the normal increase in cardiac output that develops at this gestational age (Moise, 2008a; Zimmerman, 2002).
FIGURE 15-1 Doppler measurements of the peak systolic velocity in the middle cerebral artery in 165 fetuses at risk for severe anemia. The blue line indicates the median peak systolic velocity in normal pregnancies, and the red line shows 1.5 multiples of the median. (Redrawn from Oepkes, 2006, with permission.)
Amnionic Fluid Spectral Analysis. More than 50 years ago, Liley (1961) demonstrated the utility of amnionic fluid spectral analysis to measure bilirubin concentration. This permitted an estimate of hemolysis severity and indirect assessment of anemia. Amnionic fluid bilirubin is measured by a spectrophotometer and is demonstrable as a change in optical density absorbance at 450 nm—ΔOD450. The likelihood of fetal anemia is determined by plotting the ΔOD450 value on a graph that is divided into several zones. The original Liley graph is valid from 27 to 42 weeks’ gestation and contains three zones. Zone 1 indicates an Rh D-negative fetus or one with only mild disease. Zone 2 indicates fetal anemia, with hemoglobin concentrations of 11.0 to 13.9 g/dL for values in lower zone 2 and those of 8.0 to 10.9 g/dL for upper zone 2. Zone 3 indicates severe anemia, with hemoglobin concentration < 8.0 g/dL.
The Liley graph was subsequently modified by Queenan and associates (1993) to include gestational ages as early as 14 weeks (Fig. 15-2). The naturally high amnionic fluid bilirubin level at midpregnancy results in a large indeterminate zone. Here, bilirubin concentrations do not accurately predict fetal hemoglobin concentration. For this reason, if evaluation indicated that severe fetal anemia or hydrops before 25 weeks was likely, then fetal blood sampling was often performed.
FIGURE 15-2 Proposed amnionic fluid ΔOD450 management zones in pregnancies from 14 to 40 weeks. (Redrawn from Queenan, 1993, with permission.)
Middle cerebral artery velocimetry is noninvasive and does not confer the risks for pregnancy loss or increased alloimmunization associated with amniocentesis. Importantly, it is also more accurate than ΔOD450 assessment, particularly early in pregnancy. Oepkes and coworkers (2006) compared MCA Doppler velocimetry and amnionic fluid bilirubin studies. They found that MCA Doppler had significantly greater sensitivity and accuracy. For these reasons, amnionic fluid spectral analysis is currently used only when Doppler velocimetry is not readily available. It also may be considered if the MCA peak systolic velocity exceeds 1.5 MoM after 35 weeks’ gestation. In the latter situation, if ΔOD450 assessment indicates only mild hemolysis, delivery at 37 to 38 weeks has been recommended (American College of Obstetricians and Gynecologists, 2012; Moise, 2008b).
Fetal Blood Transfusion
If there is evidence of severe fetal anemia, either because of elevated MCA peak systolic velocity or development of fetal hydrops, management is strongly influenced by gestational age. The preterm fetus is usually evaluated with fetal blood sampling, as described in Chapter 14 (p. 300). Some recommend fetal transfusion until 30 to 32 weeks’ gestation and delivery at 32 to 34 weeks. To decrease neonatal morbidity from prematurity, others suggest intrauterine transfusion up to 36 weeks followed by delivery at 37 to 38 weeks (American College of Obstetricians and Gynecologists, 2012).
Intravascular transfusion into the umbilical vein under sonographic guidance is the preferred method of fetal transfusion. Peritoneal transfusion may be necessary with severe, early-onset hemolytic disease in the early second trimester, a time when the umbilical vein is too narrow to readily permit needle entry (Fox, 2008; Howe, 2007). With hydrops, although peritoneal absorption is impaired, some prefer to transfuse into both the peritoneal cavity and the umbilical vein.
Transfusion is generally recommended if the fetal hematocrit is < 30 percent. Once hydrops has developed, however, the hematocrit is generally 15 percent or lower. The red cells transfused are type O, Rh D negative, cytomegalovirus-negative, packed to a hematocrit of about 80 percent to prevent volume overload, irradiated to prevent fetal graft-versus-host reaction, and leukocyte-poor. The fetal-placental volume allows rapid infusion of a relatively large quantity of blood. Before transfusion, a paralytic agent such as vecuronium may be given to the fetus to minimize movements and potential needle-stick trauma. In a nonhydropic fetus, the target hematocrit is generally 40 to 50 percent. The volume transfused may be estimated by multiplying the estimated fetal weight in grams by 0.02 for each 10-percent increase in hematocrit needed (Giannina, 1998). In the severely anemic fetus, less blood is transfused initially, and another transfusion is planned for about 2 days later.
Subsequent transfusions usually take place every 2 to 4 weeks, depending on the hematocrit. The sensitivity of the MCA peak systolic velocity to detect severe anemia appears to be lower following an initial transfusion, such that it may not be reliable (Scheier, 2006). One schedule is to perform a second transfusion in 10 days, the third 2 weeks later, and any additional transfusions 3 weeks later (Moise, 2012). Following transfusion, the fetal hematocrit generally decreases by approximately 1 volume percent per day. A more rapid initial decline may be seen with hydropic fetuses.
Outcomes. Procedure-related complications have been reported in up to 9 percent of transfused pregnancies (van Kamp, 2005). These include fetal death in approximately 3 percent, neonatal death in about 2 percent, need for emergent cesarean delivery in 6 percent, and infection in 1 percent. Considering that fetal transfusion is potentially lifesaving in severely compromised fetuses, these risks should not dissuade therapy.
The overall survival rate following fetal transfusion approximates 90 percent (Lindenberg, 2013; Van Kamp, 2005). If transfusion is required before 20 weeks, survival rates are lower but may reach 80 percent at experienced centers (Canlorde, 2011; Lindenberg, 2013). Van Kamp and colleagues (2001) reported that if hydrops had developed, the survival rate approached 75 to 80 percent. However, of the nearly two thirds with resolution of hydrops following transfusion, more than 95 percent survived. The survival rate was below 40 percent if hydrops persisted.
Lindenberg and associates (2012) recently reviewed long-term outcomes following intrauterine transfusion in a cohort of more than 450 alloimmunized pregnancies. Alloimmunization was secondary to Rh D in 80 percent, Kell in 12 percent, and Rh c in 5 percent. Approximately a fourth of affected fetuses had hydrops, and more than half also required exchange transfusion in the neonatal period. The overall survival rate approximated 90 percent. Among nearly 300 children aged 2 to 17 years who participated in neurodevelopmental testing, fewer than 5 percent had severe impairments. These included severe developmental delay in 3 percent, cerebral palsy in 2 percent, and deafness in 1 percent.
Prevention of Rh D Alloimmunization
Anti-D immune globulin has been used for more than four decades to prevent Rh D alloimmunization and is one of the success stories of modern obstetrics. In countries without access to anti-D immune globulin, nearly 10 percent of Rh D-negative pregnancies are complicated by hemolytic disease of the fetus and newborn (Zipursky, 2011). With immunoprophylaxis, however, the alloimmunization risk is reduced to < 0.2 percent. Despite longstanding and widespread use, its mechanism of action is not completely understood.
As many as 90 percent of alloimmunization cases occur from fetomaternal hemorrhage at delivery. Routine postpartum administration of anti-D immune globulin to at-risk pregnancies within 72 hours of delivery decreases the alloimmunization rate by 90 percent (Bowman, 1985). Additionally, provision of anti-D immune globulin at 28 weeks’ gestation reduces the third-trimester alloimmunization rate from approximately 2 percent to 0.1 percent (Bowman, 1988).
Whenever there is doubt whether to give anti–D immunoglobulin, it should be given. Even if not needed, it will cause no harm, but failing to give it when needed can have severe consequences.
Current preparations of anti-D immune globulin are derived from human plasma donated by individuals with high-titer anti-D antibodies. Formulations prepared by cold ethanol fractionation and ultrafiltration can only be administered intramuscularly, because they contain plasma proteins that could result in anaphylaxis if given intravenously. However, newer formulations, prepared using ion exchange chromatography, may be administered either intramuscularly or intravenously. This is important for treatment of significant fetomaternal hemorrhage, which is discussed subsequently. Both preparation methods effectively remove viral particles, including hepatitis and human immunodeficiency viruses. Depending on the preparation, the half-life of anti-D immune globulin ranges from 16 to 24 days, which is why it is given both in the third trimester and following delivery. The standard intramuscular dose of anti-D immune globulin—300 μg or 1500 international units (IU)—will protect the average-sized mother from a fetal hemorrhage of up to 30 mL of fetal whole blood or 15 mL of fetal red cells.
In the United States, anti-D immune globulin is given prophylactically to all Rh D-negative, unsensitized women at approximately 28 weeks, and a second dose is given after delivery if the infant is Rh D-positive (American College of Obstetricians and Gynecologists, 2010). Before the 28-week dose of anti-D immune globulin, repeat antibody screening is recommended to identify individuals who have become alloimmunized (American Academy of Pediatrics and American College of Obstetricians and Gynecologists 2012). Following delivery, anti-D immune globulin should be given within 72 hours. Importantly, if immune globulin is inadvertently not administered following delivery, it should be given as soon as the omission is recognized, because there may be some protection up to 28 days postpartum (Bowman, 2006). Anti-D immune globulin is also administered after pregnancy-related events that could result in fetomaternal hemorrhage (see Table 15-2).
Anti-D immune globulin may produce a weakly positive—1:1 to 1:4—indirect Coombs titer in the mother. This is harmless and should not be confused with development of alloimmunization. Additionally, as the body mass index increases above 27 to 40 kg/m2, serum antibody levels decrease by 30 to 60 percent and may be less protective (MacKenzie, 2006; Woelfer, 2004). Rh D-negative women who receive other types of blood products—including platelet transfusions and plasmapheresis—are also at risk of becoming sensitized, and this can be prevented with anti-D immune globulin. Rarely, a small amount of antibody crosses the placenta and results in a weakly positive direct Coombs test in cord and infant blood. Despite this, passive immunization does not cause significant fetal or neonatal hemolysis.
In approximately 1 percent of pregnancies, the volume of fetomaternal hemorrhage exceeds 30 mL of whole blood (Ness, 1987). A single dose of anti-D immune globulin would be insufficient in such situations. If additional anti-D immune globulin is considered only for women with risk factors—examples include abdominal trauma, placental abruption, placenta previa, intrauterine manipulation, multifetal gestation, or manual placenta removal—half of those who require more than the 1500-IU dose may be missed. Because of these observations, the American Association of Blood Banks recommends that all D-negative women be tested at delivery with a rosette test or Kleihauer-Betke test (Snyder, 1998).
The rosette test is used to identify whether fetal Rh D-positive cells are present in the circulation of an Rh D-negative woman. It is a qualitative test. A sample of maternal blood is mixed with anti-D antibodies that coat any Rh D-positive fetal cells present in the sample. Indicator red cells bearing the D-antigen are then added, and rosettes form around the fetal cells as the indicator cells attach to them by the antibodies. Thus, if rosettes are visualized, there are fetal Rh D-positive cells in that sample.
The Kleihauer-Betke test is a quantitative test used either in the setting of Rh D incompatibility or any time a large fetomaternal hemorrhage is suspected—regardless of antigen status. It is discussed on page 313.
The dosage of anti-D immune globulin is calculated from the estimated volume of the fetal-to-maternal hemorrhage, as described on page 311. One 1500-IU (300 μg) ampule is given for each 15 mL of fetal red cells or 30 mL of fetal whole blood to be neutralized. If using an intramuscular preparation of anti-D immune globulin, no more than five doses may be given in a 24-hour period. If using an intravenous preparation, two ampules—totaling 3000 IU (600 μg)—may be given every 8 hours. To determine if the administered dose was adequate, the indirect Coombs test may be performed. A positive result indicates that there is excess anti–D immunoglobulin in maternal serum, thus demonstrating that the dose was sufficient. Alternatively, a rosette test may be performed to assess whether circulating fetal cells remain.
Weak D Antigens
Women who are positive for weak D antigens, formerly called Du, are not considered at risk for hemolytic disease and do not require anti-D immune globulin (American College of Obstetricians and Gynecologists, 2010). There are, however, D-antigen variants—termed partial D antigens—that can result in Rh D alloimmunization and cause hemolytic disease (Daniels, 2013). If a D-negative woman delivers a weak D-positive infant, she should be given anti-D immune globulin. It is worth emphasizing that if there is any doubt regarding D-antigen status, then immune globulin should be given.
Fetomaternal Hemorrhage
It is likely that all pregnant women experience a small fetomaternal hemorrhage, and in two-thirds, this may be sufficient to provoke an antigen-antibody reaction. As shown in Figure 15-3, the incidence increases with gestational age, as does the volume of fetal blood in the maternal circulation. Large volumes of blood loss—true fetomaternal hemorrhage—are fortunately rare. In a series of more than 30,000 pregnancies, de Almeida and Bowman (1994) found evidence of fetomaternal hemorrhage > 80 mL in approximately 1 per 1000 births, and hemorrhage > 150 mL in 1 per 5000 births.
FIGURE 15-3 Incidence of fetal-to-maternal hemorrhage during pregnancy. The numbers at each data point represent total volume of fetal blood estimated to have been transferred into the maternal circulation. (Data from Choavaratana, 1997.)
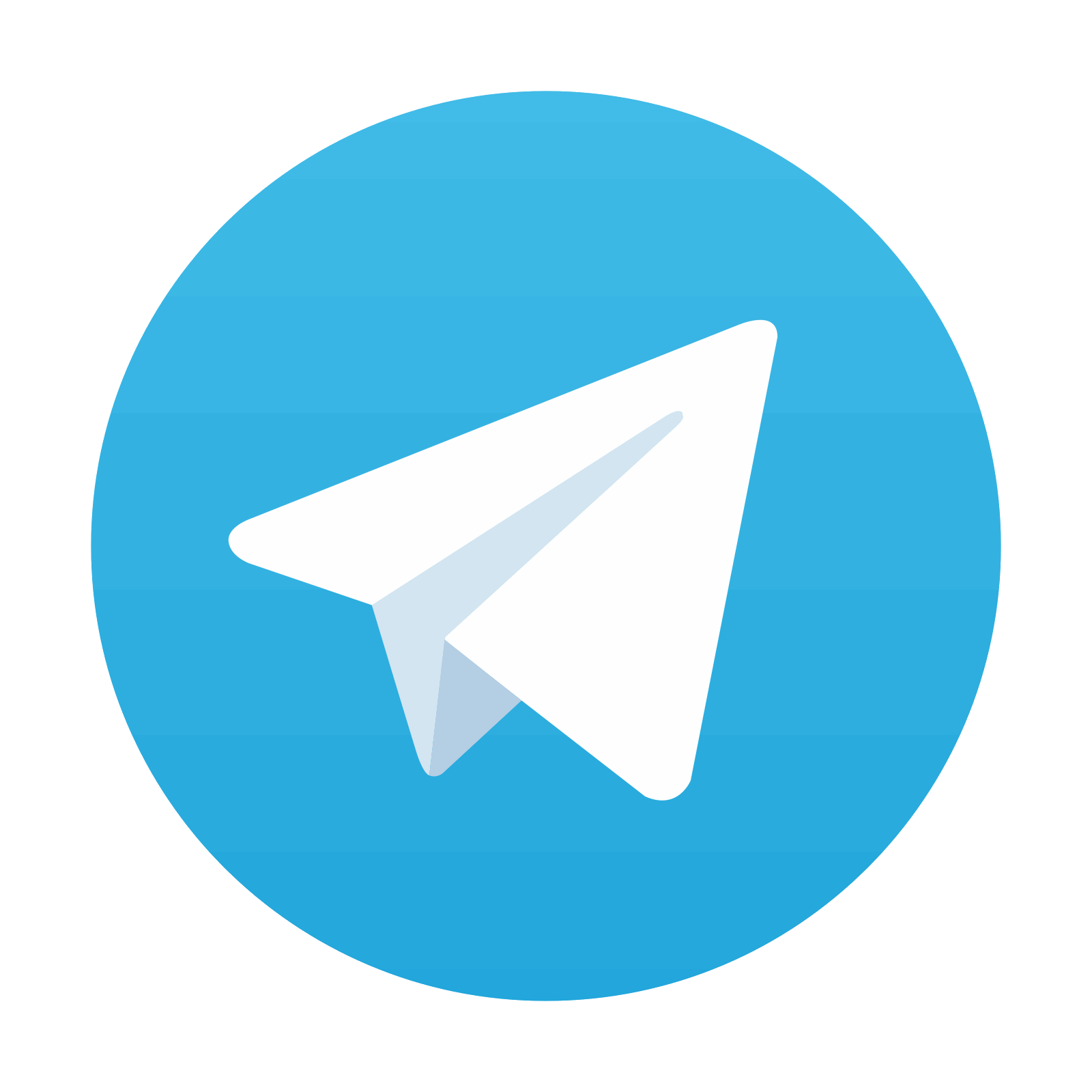
Stay updated, free articles. Join our Telegram channel

Full access? Get Clinical Tree
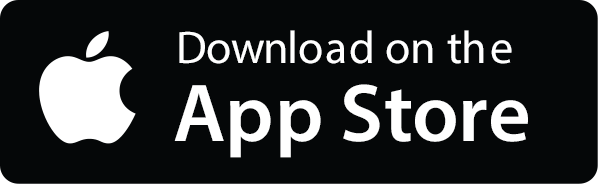
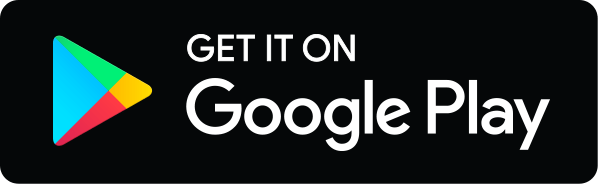