Treatment
Effect on spermatogenesis and transport
Risk of infertility
Surgery
Removal of both testes
Impaired production
100 %
Removal of one testis
Low
Damage to hypothalamic/pituitary gonadotropin producing area
Low – spermatogenesis may be stimulated with exogenous gonadotropin
Retroperitoneal lymph node dissection
Impaired transport
Variable – retrograde ejaculation; sperm production not impaired
Radiation therapy
Irradiation of testes
Impaired production
Fertility very unlikely if testes dose > 7.5 Gy
Irradiation of hypothalamic/pituitary gonadotropin producing area
Dose-response relationship unclear; dose < 30 Gy do not appear to produce damage
Chemotherapy
Alkylating agents
Impaired production
Cyclophosphamide equivalent dose (CED):
<4 g/m2 – risk of azoospermia < 15 %
>4 g/m2 – risk of oligo- or azoospermia >50 %
Males
Testicular surgery can affect production of sperm and hormones or interfere with transport of sperm [7]. Injury of the gonadotropin-releasing hormone area of the hypothalamus and/or the gonadotropin-producing anterior pituitary can also result in impaired spermatogenesis and sex steroid production [8]. Impaired transport may occur from damage to autonomic nervous system control of urethral sphincters and/or vasodilation secondary to retroperitoneal lymph node dissection or prostatectomy [9].
Testicular tissue is extremely radiosensitive with only small amounts of direct radiation required to cause significant impairment in spermatogenesis (Fig. 1.1). The immature stem cells and spermatogonia are most sensitive. Radiation to the testes markedly reduces the number of spermatocytes 2–3 weeks post-therapy with declines in ejaculated sperm counts by approximately 10 weeks. Azoospermia is typically present at 18 weeks post-therapy [10]. Spermatogenesis is significantly impaired at lower direct testicular radiation doses than is hormone production. However, both spermatogenesis and hormone production can be impaired after higher doses of radiation therapy delivered to the hypothalamic/pituitary area.


Fig. 1.1
Spermatogenesis following single-dose radiation. Howell. Spermatogenesis after cancer treatment: damage and recovery (J Natl Cancer Inst Monogr 2005;34:12–17)
The effect of chemotherapy on spermatogenesis is dependent on the type of chemotherapeutic agent. Normal sperm count typically recovers by 12 weeks post-therapy in patients treated with non-alkylating agents [11]. However, spermatogenesis is very sensitive to damage by alkylating agents including nitrogen mustard, procarbazine, cyclophosphamide, ifosfamide, chlorambucil, and busulfan, with long-lasting effects on fertility [12]. The risk of azoospermia is approximately 10 % when the cyclophosphamide equivalent dose is less than 4 g/m2, whereas approximately one-quarter of individuals who receive more than this dose will retain a normal sperm concentration [13].
Females
Surgery for ovarian tumors impairs fertility and hormone production by decreasing the number of follicles present. Fertility-sparing surgery is the standard of care for the management of early-stage, low-grade ovarian tumors in women who have not completed their childbearing. Surgical options include cystectomy for tumors of low malignant potential (borderline tumors) and unilateral oophorectomy for malignancies [14–16]. When cystectomy is performed, the remaining ovary is typically able to compensate if no postoperative chemotherapy or radiation is given. Pelvic surgery for non-gynecologic malignancies can also have a deleterious effect on ovarian function by cytokine production and formation of pelvic adhesions, with subsequent impaired folliculogenesis, ovulation, and tubal transport [17, 18].
All chemotherapeutic agents affect the mature follicle through DNA damage with subsequent apoptosis and temporary amenorrhea [19]. If the primordial follicle pool is unaffected, folliculogenesis will resume after completion of cancer treatment with resumption of menses. Alkylating agents such as cyclophosphamide, busulfan, and nitrogen mustard and heavy metals such as cisplatin have a deleterious effect on the primordial follicles, diminishing the reserve pool [20]. Although patients who receive these therapies may resume menses due to follicular maturation of the remaining follicles, they subsequently experience accelerated folliculogenesis and apoptosis with primary ovarian insufficiency [21].
Radiation injury to the ovaries is dose dependent. Specifically, younger age confers protection from post-therapy infertility because of the larger pool of primordial follicles present at the time of radiation treatment. Radiation doses greater than 2 Gy result in a loss of 50 % of ovarian follicles, described as the LD50 [22, 23]. Doses greater than or equal to 15 Gy and 6 Gy in adult and prepubertal patients, respectively, result in infertility. Irreversible damage to the uterus occurs at abdominopelvic doses greater than 30 Gy [24]. Radiation exposure to the hypothalamus and pituitary gland greater than 30 Gy affects production of gonadotropins with decreased folliculogenesis, decreased production of estrogen, and infertility [22].
Risk stratification from chemotherapy is based on the cumulative dose of alkylating agents received due to high risk of gonadotoxicity. The alkylating agent dose (AAD) and the cyclophosphamide equivalent dose (CED) risk-stratification systems allow calculation of risk (Fig. 1.1 and Table 1.2). Using the AAD, a score of 1, 2, or 3 is given for the cumulative dose of each alkylating agent that falls within the first, second, or third cumulative dose tertile, respectively. The scores for individual agents are summed [25]. Patients with a score of 3 or 4 are at increased risk of infertility with a relative risk of pregnancy of 0.72 and 0.65, respectively [26]. The CED is calculated by summing the cyclophosphamide equivalents for cyclophosphamide, ifosfamide, procarbazine, chlorambucil, BCNU, CCNU, melphalan, ThioTEPA, nitrogen mustard, and busulfan [27] (Table 1.2). A CED > 7.5g/m2 is associated with a relative risk of premature menopause of 4.19 (95 % is 2.18–8.08).

Table 1.2
Estimating risk: alkylating agent dose (AAD) (Green. J Clin Oncol. 27:2677–2685)
Tertile distribution of alkylating agents in cumulative dose | |||
---|---|---|---|
Cumulative dose by rertile | |||
Alkylating agent | First | Second | Third |
BCNU, mg/m2 | 1–300 | 301–529 | 530–3,370 |
Busulfan, mg/m2 | 1–317 | 318–509 | 510–6,845 |
CCNU, mg/m2 | 1–361 | 362–610 | 611–3,139 |
Chlorambucil, mg/m2 | 1–165 | 166–634 | 635–3,349 |
Parenteral cyclophosphamide, mg/m2 | 1–3,704 | 3,705–9,200 | 9,201–58,648 |
Oral cyclophosphamide, mg/m2 | 1–4,722 | 4,723–10,636 | 10,637–143,802 |
Ifosfamide, mg/m2 | 1–16,771 | 16,772–55,758 | 55,759–192,391 |
Melphalan, mg/m2 | 1–39 | 40–137 | 138–574 |
Nitrogen mustard, mg/m2 | 1–44 | 45–64 | 65–336 |
Procarbazine, mg/m2 | 1–4,200 | 4,201–7,000 | 7,001–58,680 |
Intrathecal thiotepa, mg | 1–80 | 81–320 | 321–914 |
Thiotepa, mg/m2 | 1–77 | 78–220 | 221–3,749 |

Fig. 1.2
Cyclophosphamide equivalent dose calculation
The AAD is based on drug dose distribution from the specific cohort of patients from which the drug dose distribution was derived, whereas the CED is derived from actual drug doses and therefore has applicability independent of the study population [27]. Risk stratification by alkylating agent should be performed prior to therapy to guide implementation of fertility preservation therapies based on risk. However, treatment regimens may change during the course of therapy, and in such instances, cumulative dose and risk assessment may be recalculated post-therapy.
Minimizing Risk
Several agents have been proposed as potentially fertoprotective, or conferring protection against the damaging effects of chemotherapy and radiation (Table 1.3). Gonadotropin-releasing hormone agonists (GnRHa) are the most studied; however, results are conflicting. Several newer agents are currently being evaluated which show some promise. These agents include imatinib, bone marrow-derived mesenchymal stem cells (BMMSC), sphingosine-1-phosphate (S1P), tamoxifen, granulocyte colony-stimulating factor (G-CSF), and AS101. GnRHa, tamoxifen, and G-CSF are the only agents that have been used in humans. Other therapies have shown promise in rodent and primate studies; however, concerns remain about interference with chemotherapeutic efficacy and perpetuation of damaged DNA cell lines with resultant fetal loss and/or malformation. Further studies are required to determine efficacy and safety in humans.
Table 1.3
Potential fertoprotective agents during cancer treatments
Potential fertoprotective agents during cancer treatments Protective agent | Mechanism of action on ovary | Studies demonstrating protective effect | Studies demonstrating no effect | Interactions with cytotoxic treatments |
---|---|---|---|---|
GnRH analog | Direct effect on ovary is unclear; suppresses hypothalamic-pituitary-ovarian axis, possible ovarian quiescence | Rodent: Meirow et al. (2004), Li et al. (2013) Primate: Ataya et al. (1995) Human: Badawy et al. (2009), Severrisdottir et al. (2009), Del Mastro et al. (2011), Demeestere et al. (2013) | Human: Gerber et al. (2011), Munster et al. [28], Elgindy et al. (2013), Demeestere et al. (2013) | No interference with treatment drugs |
Imatinib | Inhibit c-Abl kinase apoptosis pathway | Rodent: Gonfolini et al. (2009) | Rodent: Kerr et al. [29] | May interfere with apoptotic action of chemotherapy drugs |
Bone marrow mesenchymal stem cells | Tissue differentiation, angiogenesis, anti-apoptosis | NTD | May cause chemotherapy drug resistance with cisplatin | |
S1P | Inhibit sphingomyelin apoptosis pathway | Primate: Zelinski et al. [35] Human xenograft: Zelinski et al. [35] | Rodent: Kaya et al. [34] | May interfere with apoptotic action of chemotherapy drugs |
Tamoxifen | Anti-apoptotic activity; antioxidant activity via IGF-1 axis; possible H-P-O axis suppression | Human: Sverrisdottir et al. [38] | Adjuvant therapy; no interference with treatment drugs | |
AS101 | Inhibits P13K/PTEN Akt follicle activation pathway; anti-apoptosis | Rodent: Kalich-Philosoph et al. [39] | NTD | No interference w/treatment drugs May have additive/synergistic interaction w/treatment drugs |
Growth-colony stimulating factor (G-CSF) | Unclear: possibly angiogenesis; anti-apoptosis | Rodent: Skaznik-Wikiel et al. [40] | NTD | No interference with treatment drugs |
Gonadotropin-Releasing Hormone Agonist
Gonadotropin-releasing hormone agonist (GnRHa) therapy is the most studied fertoprotective agent, but with conflicting results [41–43]. Reasons include endpoints that do not predict long-term ovarian function such as resumption of menstruation, follicle-stimulating hormone (FSH) and estradiol levels, and a lack of data on pregnancy outcomes. Additionally, studies that used different GnRHa therapies had short follow-up periods and few were randomized [28, 44]. A recent prospective study with 257 patients used similar endpoints with inclusion of pregnancy outcome and survival and showed improved pregnancy outcomes with goserelin in hormone receptor negative breast cancer patients receiving adjuvant therapy [45]. Ultimately, meta-analysis of GnRHa therapy has shown ovarian protection in breast cancer patients, but widespread use of GnRHa therapy as an ovarian protective agent in all malignancies cannot be recommended at this time [42]. For this reason, additional agents have been evaluated for efficacy.
Imatinib
Imatinib is a competitive tyrosine kinase inhibitor used for cancer treatment. Chemotherapy induces c-Abl-mediated upregulation of tumor suppressor protein p63 (a homolog of p53) with resultant apoptosis. Rodent studies show that when given prior to cisplatin, imatinib is a potent inhibitor of c-Abl-mediated upregulation and blocks apoptosis of cells. Mice treated with imatinib prior to cisplatin show reduced primordial follicular loss and normal progeny [46, 47]. However, other studies show no protection with imatinib in two independent mice strains [29]. Additionally, the authors show that genetic effects on the oocyte result in early embryonic mortality and marked aneuploidy. There remain concerns regarding whether imatinib- and cisplatin-treated oocytes that do not undergo apoptosis harbor DNA damage that may result in miscarriage or birth defects [48, 49]. The question also remains whether imatinib reduces the efficacy of cisplatin on the primary tumor target while upregulating the effects of cisplatin in other cell types [50].
Bone Marrow-Derived Mesenchymal Stem Cells
Bone marrow-derived mesenchymal stem cells (MSC) have been used to treat various diseases because of their self-renewal capacity and multi-potency [51, 52]. For example, stem cells have been successfully employed for tissue repair after spinal, renal, and myocardial injury [53, 54]. The potential benefit of stem cell therapy after acute tissue damage appears to be related to tissue integration and differentiation to replace damaged cells, angiogenesis, and anti-apoptosis. Adult MSC have not been unequivocally proven to differentiate into follicles; however, several rodent studies have been conducted to assess the role of MSC as an ovarian protective agent from chemotherapy. Kilic et al. showed preservation of primordial and primary follicles in in vivo rat MSC studies, suggesting that MSC may preferentially migrate to the injured follicular cells and repair the ovarian tissue by decreased programmed cell death [55]. Similarly, Fu et al. demonstrated an increase in follicle number as well as a normalization of FSH and estradiol levels after several weeks in rodents treated with MSC after cyclophosphamide therapy [30]. They also illustrated in vitro production of angiogenic and anti-apoptotic cytokines, including vascular endothelial growth factor (VEGF), insulin-like growth factor (IGF-1), and hepatocyte growth factor (HGF) from MSC. Effects of therapy on progeny were not assessed in either study. Abd-Allah et al. further demonstrated MSC protection of ovarian follicles in rabbit studies and were able to show in vivo cytokine production [31]. Despite the potential promise of MSC as an ovarian protection agent, studies of MSC injected intravenously in rodent models have shown that MSC may mediate tumor resistance to cisplatin [56]. Therefore, route, dose, and efficacy of MSC as an ovarian protective agent in primates and humans are warranted.
Sphingosine-1-phosphate (S1P)
There are several apoptotic pathways, including the pathway which triggers apoptosis of ovarian follicles. In the pathway, sphingomyelin is degraded to ceramide which has pro-apoptotic effects. Ceramide is subsequently degraded to sphingosine and then sphingosine-1-phosphate (S1P) through hydrolysis. Sphingosine-1-phosphate regulates proliferative cellular processes, including cell growth and cell differentiation, and inhibits apoptosis [33]. In vivo mice studies of ovarian tissue xenografts treated with S1P show increased vascular density and angiogenesis with reduced follicular apoptosis. However, in mice treated with S1P pre-chemotherapy, the evidence is inconclusive, with some studies showing a protective effect in the presence of dacarbazine [33] and others showing no effect in the presence of cyclophosphamide [34]. Conversely, S1P treatment prior to radiation therapy has been shown to be effective in a dose-dependent manner with preservation of both primordial and primary follicles in rats, primates, and xenografted ovarian tissue [32, 34, 35]. Male mice who received local treatment with S1P prior to radiation were also shown to have protection of early stages of spermatogenesis [57]. Limitations of S1P are that it must be injected into tissue rather than administered systemically, thus limiting its clinical usefulness. A benefit of this targeted treatment is that it may minimize interference with cancer therapy efficacy in other tissues. This has not been adequately studied, however. Lastly, consistent with concerns with other fertoprotective agents, inhibition of follicular apoptosis with S1P may result in transmission of genetically damaged DNA. Studies of offspring in mice and primates treated with S1P prior to radiation showed no propagation of DNA damage and no abnormalities in the offspring [58].
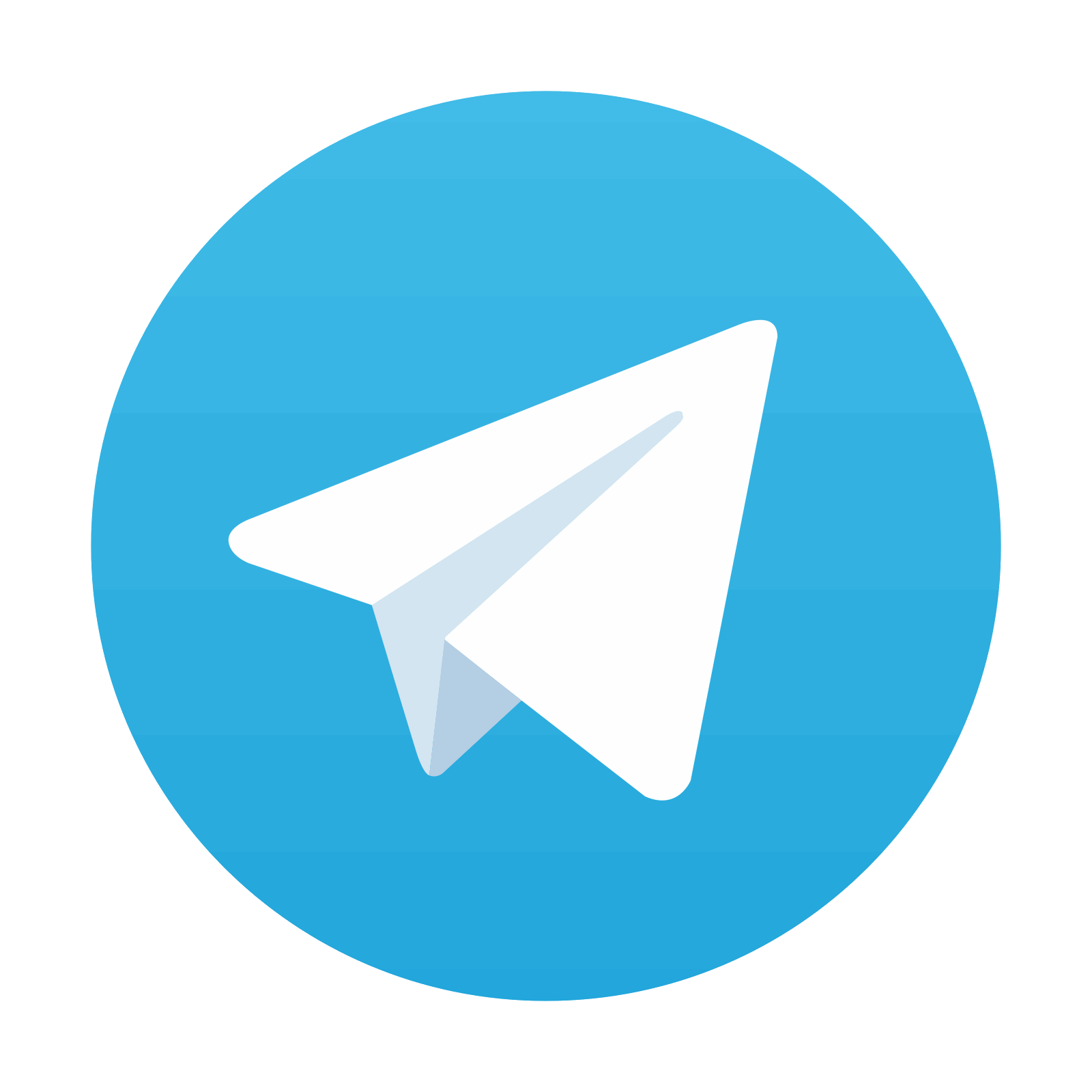
Stay updated, free articles. Join our Telegram channel

Full access? Get Clinical Tree
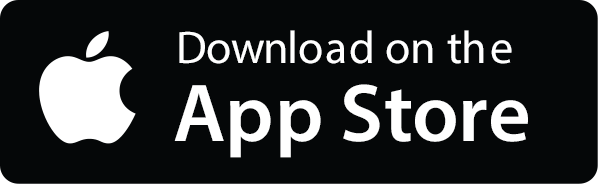
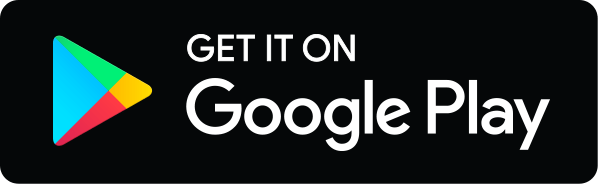