Fig. 2.1
This schematic of the ovary depicts the developing follicle and oocyte in the ovarian cortex. The outermost layer comprises a thin layer of epithelial cells known as the germinal epithelium , which gives rise to primordial oocytes during fetal growth. Just below there is a strong connective tissue layer known as the tunica albuginea . The medulla, located at the center of the ovary, houses blood vessels and ligaments that are vital to the survival and function of the ovary
2.3 Overview of Oogenesis
In humans, oogenesis begins approximately 3 weeks after fertilization . PGCs arise from the yolk sac and migrate via amoeboid movements, through the hindgut, to the genital ridge [6–9]. PGCs undergo rapid mitotic division during this migration. The genital ridge, formed at around 3.5–4.5 weeks gestation, is composed of mesenchymal cells overlaid with coelomic epithelial cells. Upon arrival, the PGCs give rise to oogonia or germ stem cells (GSCs) that continue to proliferate to further expand the germ cell pool. The number of oogonia increases from 600,000 by the eighth week of gestation to over ten times that number by 20 weeks. At around 7 weeks’ gestation, these cells form the primitive medullary cords and the sex cords, respectively.
Follicle formation begins at around week 16–18 of fetal life. Oogonia are enveloped by somatic epithelial cells derived from genital ridge mesenchymal cells, forming primordial follicles. The oogonia then cease mitotic activity and enter meiosis [8, 10, 11].
Once meiosis has been initiated, the oogonial germ cell is termed a primary oocyte. The surrounding mesenchymal somatic cells secrete the follicle’s basement membrane and give rise to the granulosa cell layer. By 4–5 months’ gestation, the ovary has its maximum number of oocytes, between 5 and 8 million. This number decreases dramatically to 1–2 million at birth and less than 500,000 by puberty [12]. The primordial follicles containing these immature oocytes remain essentially dormant. Through the next 35–40 years of a women’s reproductive life span, a small number of follicles are steadily released into the growing pool [13–15].
2.3.1 Oocyte Development and Meiosis
Oogenesis is the process by which the mature ovum is differentiated. It is not clear whether the signals controlling germline entry into meiosis are cell-autonomous or dependent on the mesonephric somatic cells. Meiosis is unique to germ cells. It results in the production of daughter cells with haploid DNA content as a result of a two-step division. During oogenesis, cell division is unequal. The result is a single ovum, with excess genetic material extruded as polar bodies [16]. This is quite unlike spermatogenesis , where meiosis results in four identical haploid daughter cells [17].
The four main stages of meiosis are prophase , metaphase , anaphase , and telophase (◘ Fig. 2.2). DNA replication, chromosome pairing, and recombination, steps that are integral to sexual reproduction, all occur during prophase. Prophase can be subdivided into four stages, leptotene, zygotene, pachytene, and diplotene. DNA replication is finalized in preleptotene, while in leptotene, sister chromatids search for their homologous counterparts. Interaction between homologous chromosomes is facilitated by the formation of recombination nodules. In zygotene, homologous chromosomes pair and begin to synapse. The synapses are maintained by the synaptonemal complex. The crossing over and recombination of chromosomes occur in the pachytene stage, prior to the formation of ovarian follicles . Synapsis is completed in pachytene, and by the diplotene stage, homologous chromosomes are held together mainly at sites of chiasmata. Oocytes in primordial follicles are arrested at the diplotene stage of the first meiotic prophase [1, 18].


Fig. 2.2
The stages of meiosis I and II leading to the ovulation of a haploid ovum are depicted. Unlike with spermatogenesis , meiosis during oogenesis results in disproportionate cell division with the production of a single ovum with extraneous genetic material being extruded in the first and second polar bodies. During this division, the majority of the cytoplasm, containing important proteins, organelles, and growth factors, remains within the oocyte
The nucleus in the prophase oocyte is called the germinal vesicle . Ooplasmic factors prevent the resumption of meiosis in the prophase oocyte until it reaches a specific diameter and stage [13, 19–22]. This stage, referred to as “meiotic competence ,” occurs in the antral follicle . Once meiosis resumes, there is rapid progression through the metaphase, anaphase, and telophase stages of the first meiotic division. The oocyte then arrests at metaphase 2 of the second meiosis until sperm entry. Oocyte morphology at different stages of maturity is shown in ◘ Fig. 2.3.


Fig. 2.3
Various stages of oocyte maturation : a immature oocyte in prophase I of meiosis I; b metaphase I oocyte; c cumulus:oocyte complex exhibiting morphology typically associated with mature oocytes at the time of ovulation; d mature metaphase II oocyte
2.3.2 Follicle Development
Folliculogenesis within the ovary is a very complex process with a high rate of follicle loss. Follicles periodically leave the resting primordial pool to join the growing pool but, in the absence of follicle-stimulating hormone (FSH) , undergo atresia. After puberty, once the hypothalamus–pituitary–ovarian axis has been activated during the follicular phase, elevated FSH levels rescue the growing cohort of follicles. The ovarian paracrine signaling induces the continued growth of follicles from this cohort in a process called initial recruitment [23]. The recruited growing follicles, known as primary follicles , will subsequently grow into secondary and antral follicles. ◘ Figure 2.4 illustrates the different stages of follicle development. Ultimately, a single follicle will be selected from this cohort to become the dominant follicle. It will release a mature oocyte after exposure to increased levels of LH (luteinizing hormone). Almost 90% of follicles undergo apoptosis or programmed cell death without ever becoming meiotically competent [24].


Fig. 2.4
Depicted is a sequential illustration of folliculogenesis within the human ovary. The process begins with the oocyte enclosed within single layer of granulosa cells known as the primordial follicle and ends with a fully developed multilayered follicle with antrum containing a secondary oocyte
Critical to this process is the interaction between the somatic cell components and the oocyte itself. Follicle growth from the primordial to the preovulatory stage can be divided into two distinct stages based on responsiveness to the gonadotropins, FSH and LH. The first stage is relatively slow in humans, taking over 120 days [13, 25] and is not directly dependent on gonadotropin levels. Key growth mediators at this early stage may include TGF-β(beta), activin, bone morphogenetic proteins (BMPs), anti-Müllerian hormone (AMH ), insulin, estrogen, and androgens. Follicle and oocyte diameters increase, follicles growing in size from 30 to 40 μm in primordial follicles to 100–200 μm in pre-antral follicles (see ◘ Fig. 2.4). The single layer of squamous granulosa cells present in the primordial follicles starts to proliferate and the oocyte becomes surrounded by several layers of cuboidal granulosa cells. Precursor thecal cells are recruited from surrounding stroma and a basement membrane forms around the follicle.
The second stage of follicular growth is far more rapid. The follicle is now responsive to the gonadotropins, FSH and LH. Granulosa cell secretions result in the formation of a fluid-filled cavity or antrum. During the early antral stage of follicle development, follicle size increases from 200 μm to 2–5 mm. Follicle size increases to 20 mm by the time of oocyte ovulation.
The formation of a fluid-filled antrum and synthesis of steroid hormones mark the transition to the antral stage of follicle development. During this stage and with the influence of FSH, granulosa cells differentiate and become capable of aromatizing androgen, secreted by thecal cells, into estrogen. The local estrogenic environment, combined with high FSH levels, promotes further granulosa cell proliferation and an increase in FSH receptors. This, in turn, makes the follicles even more sensitive to FSH. The negative feedback of rising estrogen levels on the hypothalamus–pituitary axis limits FSH secretion. Thus, only follicles with increased FSH receptors will be able to continue to develop, while other follicles in the cohort will undergo atresia. It is through this mechanism that a single dominant follicle is selected. Continued growth of the selected follicle occurs despite the midcycle fall in FSH concentrations as a result of granulosa cells now acquiring LH receptors [26–28]. While granulosa cells of the early antral follicle are only responsive to FSH, late antral stage follicles become responsive to both FSH and LH and continue to secrete high levels of estradiol [29, 30]. The layers of specialized granulosa cells bordering the oocyte are known as cumulus cells , which are also called corona radiata . These cells not only support cytoplasmic maturation, but are pivotal in maintenance of meiotic arrest and induction of ovulation. The preovulatory follicle, also known as a Graafian follicle , measures over 18 mm in size, and oocyte diameter is close to its final size of about 120 μm [31]. The multilayer follicle is enclosed in a basement membrane that separates it from the underlying vascularized thecal cell layer.
2.3.3 Oocyte Growth
Coordinated growth of this diplotene-arrested oocyte and follicle is dependent on bi-directional communication between the oocyte and the surrounding granulosa cells [32, 33]. This communication occurs via gap junctions connecting the granulosa cells and the oocyte [33–39]. These membrane channels enable the sharing of small essential molecules, including inorganic ions, second messengers, small metabolites, and secreted paracrine factors that allow growth of both cell types [39–42].
The oocyte is unable to transport certain amino acids, carry out biosynthesis of cholesterol, or undergo glycolysis without a supply of the necessary factors by the granulosa cells . Similarly, evidence suggests that granulosa cell proliferation as well as select other metabolic processes require oocyte-derived secretions [43–46]. In vitro studies on cultured follicles demonstrate that severing of gap junctions and intercellular communications triggers premature ovulation and eventual degeneration of the released oocyte [47].
Gap junctions are comprised of a variety of connexin proteins [37]. The basic structure of connexins consists of four membrane-spanning domains, two extracellular loops, a cytoplasmic loop, and cytoplasm N-and C-termini. Different connexins contain different properties, providing increased complexity in the regulation of designated molecules. Gene knock-out experiments in the mouse model have identified specific gap junction proteins and their critical role in folliculogenesis. Absence of connexin-37 interferes with antral follicle formation [48, 49], while follicles in mice lacking the gene for connexin-43 arrest in the early pre-antral stage and are unable to produce meiotically competent oocytes [50]. Phosphorylation and several different protein kinases appear also to be associated with the activation and regulation of gap junctions [51, 52].
The oocyte is metabolically active from its early growth stage, synthesizing the maternal RNA pool necessary to support early embryonic events after fertilization . Oocytes synthesize over 400 different proteins. Shortly after follicle activation and entry into the growing pool, the oocyte secretes a thick glycoprotein coat [53, 54]. This matrix coat, known as the zona pellucida , encircles the oocyte and is composed of three zona pellucida proteins, ZP1, ZP2, and ZP3. Thickness of the zona pellucida increases as the oocyte grows, reaching about 15 μm. The zona pellucida plays an important role in protecting the oocyte/embryo as it traverses the reproductive tract. The zona mediates sperm binding, confers species specificity, and serves as a block to polyspermic fertilization . Release of cortical granules by the oocyte cytoplasm at the time of fertilization results in a hardening of the zona and deters additional sperm from penetrating into the oocyte.
2.3.4 Resumption of Meiosis and Ovulation
Meiotic progression of the oocyte is highly dependent on a delicate balance between factors keeping the oocyte in meiotic rest and factors promoting oocyte maturation [55–61]. Cyclic AMP is one of the intracellular signaling molecules that keep the oocyte in meiotic arrest. Cyclic AMP, produced by granulosa cells, is transported via gap junctions to the oocytes. As long as the cAMP threshold is maintained, meiosis is inhibited.
Meiotic competence is also linked to oocyte size, presumably because increasing volume corresponds to increasing cytoplasmic accumulation of synthesized proteins. Oocytes that measure less than 70–80 μm have lower rates of meiotic competence compared to 100 μm fully grown follicles , which are usually able to resume meiosis. Activation of maturation or M-phase promoting factor (MPF ) is required to resume meiosis [62, 63]. MPF levels increase with oocyte growth and eventually reach a threshold, at which point the oocyte becomes meiotically competent. MPF is composed of a regulatory unit, cyclin B, and its protein kinase CDK1 (also called p34cdc2 ).
The onset of the LH surge triggers a cascade of events culminating in the ovulation of the oocyte from the Graafian follicle and initiation of oocyte maturation. LH induces a shift in steroidogenesis by granulosa cells to progesterone [64]. Cumulus cell expansion just prior to ovulation results in the severing of cumulus: oocyte connections, thus reducing intracellular cAMP levels in the oocyte levels and therein reducing its inhibitory influence on meiosis. Subsequent MPF activation drives the oocyte towards meiosis, starting first with breakdown of the germinal vesicle (GVBD ) . Neosynthesis of cyclin B may be a rate-limiting step, accounting for the observed lag time between resumption of meiosis and GVBD, as well as transition from metaphase I to metaphase II.
After the breakdown of the germinal vesicle, bivalents start to become organized and then align at the metaphase plate, forming a meiotic spindle. The oocyte remains arrested at the metaphase II stage until sperm penetration. An intracellular Ca2+ signal triggered by either the binding of sperm to oocyte receptors or else the release of a soluble sperm-derived factor during oocyte: sperm fusion initiates the destruction of endogenous cyclin [63]. The oocyte is now able to complete meiosis, with chromosome segregation beginning at the metaphase–anaphase transition. Defective chromosome segregation at this stage can lead to aneuploidy in the resulting egg and embryo.
2.4 Additional Regulatory Mechanisms
Clear maintenance of the primordial follicle pool, follicle recruitment, follicle atresia and selection of a dominant follicle, combined with controlling oocyte maturation, and synchronous growth of the follicular unit, is a complex process. In this section, we discuss a few of the most important ovarian paracrine factors involved in the coordination of events during folliculogenesis (◘ Table 2.1).
Table 2.1
Human oogenesis and folliculogenesis beginning with the primordial germ cells (key modulators and their points of action are shown)
Developmental event | Key regulators |
---|---|
Primordial germ cells | |
3 wk pc | BMP4, BMP8b |
Formation | c-KIT, SCF |
Migration and proliferation | |
Colonization of the forming gonad | |
Post-migratory survival | |
Oogonia | |
Proliferation by mitosis | |
Oocyte meiotic prophase 1 | |
Preleptotene: replication of DNA | |
Leptotene: start of homologous pairing | |
Zygotene | |
Pairing of homologs | |
Synapsis | |
Pachytene | |
Crossing over | |
Recombination | |
DNA repair | |
Diplotene: meiotic arrest | cAMP |
Primordial follicle | Activins, AMH , TGF β, BMPs, insulin, estrogen , androgens, c-KIT |
16–18 wk pc | |
Diplotene-arrested oocyte | |
Single flat granulose cell layer | |
Primary follicle | Activins, AMH |
Cuboidal granulose cell layer | |
Pre-antral secondary follicle | Activins, Inhibins, AMH |
Late folliculogenesis | |
Granulosa proliferation | GDF-9, BMP-15 |
Theca precursor formation | |
Antral/Graafian follicle | Inhibins, AMH , cAMP , FSH/FSH receptor, LH/LH receptor |
Formation of antrum | |
Formation of preovulatory follicles and corpus luteum | |
Cumulus expansion | LH signaling, PGE2 |
Meiotic resumption | MPF |
Ovulation | COX-2, LH |
Oocyte maturation and fertilization | Ca2+ |
wk pc weeks post coitum, AMH anti-Müllerian hormone, BMP bone morphogenetic protein, GDF growth differentiation factor, FSH follicle-stimulating hormone, LH luteinizing hormone, SCF stem cell factor, COX–2 cyclooxygenase isoform, PGE2 prostaglandin E(2)
2.4.1 cAMP
As mentioned earlier, FSH and LH, working in concert, are two of the most important hormones involved in folliculogenesis. An important component of this system is the second messenger cAMP , which amplifies the signal induced by FSH and LH. This amplification allows for a much larger response to the FSH and LH hormones than they are able to create alone [65]. Two important sets of enzymes control intracellular cAMP homeostasis: adenylyl cyclases, which generate cAMP, and the phosphodiesterases (PDEs) , which break down cAMP. Binding of FSH and LH to their respective receptors, along with adenylyl cyclase, activates the generation of cAMP from ATP in follicular granulose cells. The cAMP molecules activate a cascade of protein kinases, dissociating their catalytic component, which then works to phosphorylate transcription factors. These factors bind to DNA upstream of genes at positions known as cAMP response elements , regulating various follicular events such as the growth of the dominant follicle [66]. In the oocyte, a high concentration of this second messenger prevents meiosis.
2.4.2 TGF-β Growth Factor Family
Several key regulators involved in folliculogenesis belong to the transforming growth factor-β (TGF-β ) family, many of which utilize gap junctions for communications. Important members of this group include AMH , growth differentiation factor-9 (GDF-9), BMPs, activin, and inhibin.
2.4.3 Anti-Müllerian Hormone
AMH is secreted by granulosa cells when a woman is reproductively active. In studies utilizing the mouse model, AMH has been shown to have two important roles during folliculogenesis. First, AMH inhibits the recruitment of additional primordial follicles when there is already a growing cohort of follicles. By this means, AMH prevents women from speeding too quickly through their oocyte reserve. Second, AMH decreases the response of these growing follicles to FSH. Studies in humans have suggested that each follicle has a unique FSH threshold that must be met in order to proceed to the preovulatory stage. AMH may influence how responsive a follicle is to the FSH surge during cyclic recruitment [67].
AMH has also been used as a measure of a woman’s ovarian reserve [68]. AMH levels decrease along with the size of a woman’s follicle pool. Concomitantly, lower AMH levels fail to adequately inhibit the primordial pool, and as a result there is an increase in the rate of depletion of the follicular reserve [69].
2.4.4 Growth Differentiation Factor-9 (GDF-9) and Bone Morphogenetic Proteins
BMPs play a variety of roles in oogenesis and are produced by a variety of cells. While little is known about specific BMP function in the human ovary, results from numerous animal studies have contributed to our understanding of the biological activities of BMPs during follicular development. Evidence from the rat model proposes that BMPs influence the primordial-primary transition as well as the subsequent transition to a secondary follicle . It has also been suggested that a drop in BMP levels may be indicative of dominant follicle selection [70, 71]. In the rat, thecal cells of secondary follicles have expressed BMP-4 and BMP-7, while their respective receptors expressed on granulosa cells [71]. They serve to modulate the actions of FSH on the synthesis of the essential steroids estradiol and progesterone [72].
Oocyte paracrine signaling is responsible for activating pathways involved in regulating cumulus cell differentiation. BMP-15 and GDF-9 are two factors secreted by the oocyte to control its local microenvironment and ultimately oocyte quality [45]. BMP-15 works alongside GDF-9 to activate signaling pathways responsible for the regulation of cumulus cell differentiation and maintenance of their phenotype [45]. The absence of these oocyte-specific factors has been demonstrated to result in sterility [73, 74]. During in vitro culture of ovarian cortical slices, GDF-9 supplementation was observed to increase the number of secondary oocytes present after 7 days of growth and also enhanced follicle density after 14 days of culture. GDF-9 may also serve to prevent atresia [75].
2.4.5 Activin and Inhibin
Activin and inhibin are produced by granulosa cells in the follicle and work in an antagonistic relationship. Activin, produced by many different tissues, including the gonad and anterior pituitary, stimulates the release of FSH by acting as a transcription factor activator of the FSHβ-subunit gene [76]. Meanwhile, inhibin hinders the secretion of FSH from the pituitary, balancing the actions of activin. While it is believed that inhibin plays an important role in the production of steroids and gonadotropins, little else is known about its role in the recruitment and differentiation of thecal cells [77].
2.4.6 c-Kit and Kit Ligand
Another important signaling pathway involved in the regulation of primordial follicles involves the c-Kit receptor and its granulosa cell produced ligand (frequently referred to as KL, stem cell factor or SCF) [78]. Little is known about the specific role of this receptor/ligand combination in the early development of follicles. The presence of the mRNA encoding for c-Kit and KL has been detected in early antral follicles present in human ovarian tissue, oocytes, and granulosa cells [78]. Much of what is known about c-Kit comes from research in the mouse model. Here, c-Kit and KL play a role in PGC survival, activation, migration, proliferation of granulosa cells , recruitment of theca cells, maintenance of meiotic competence, and the development of follicles [79].
2.5 Future Directions and Challenges
Increasing our understanding of the complex regulation of ovarian folliculogenesis and the interactions between the oocyte and granulosa-thecal cell layers has contributed significantly to the advancement of infertility treatment. Medications and ovarian stimulation regimens have been designed which permit the manipulation of a women’s menstrual cycle , resulting in the production of multiple mature competent oocytes. This, combined with advances in our ability to treat male factor infertility, has dramatically altered pregnancy outcomes with in vitro fertilization over the last two decades.
One hurdle that has been challenging to overcome has been poor ovarian reserve. The continuous loss and eventual elimination of a woman’s follicle pool through accelerated atresia is considered to be the impetus leading to menopause. This is based on the belief set forth over 50 years ago that oogenesis cannot occur in the adult ovary and so, at birth, the female ovary contains a finite oocyte pool [80]. Recently, exciting data have emerged that question this basic established dogma [81, 82]. The intriguing study, first presented in 2004 by Johnson and colleagues, questions the concept of a “non-renewing oocyte pool ” [83]. An increasing body of evidence indicates that oogenesis may in fact occur in the adult mammalian ovary [82, 84, 85]. The potential existence of germ cells in the adult ovary and the development of techniques to manipulate such cells may open up new avenues for fertility treatment [86].
Another challenge in reproductive medicine has been fertility preservation for young women diagnosed with cancer. Radiation and chemotherapy during cancer treatment can result in fertility loss through damage to the ovarian follicle reserve. Cryopreservation of ovarian tissue offers hope to patients, but how best to use this tissue to restore fertility is still problematic. Ovarian tissue transplant after the patient is in remission has been met with limited success. Additionally, the possibility of reintroducing the cancer always remains. An alternate solution is the isolation of ovarian follicles from cryopreserved tissue, followed by in vitro maturation of the follicles [87]. The long time interval necessary for human follicle growth from the primordial to the preovulatory stage (∼120 days) and the intricate signaling mechanisms necessary for proper follicle growth are quite difficult to mimic in vitro. Maintenance of the spheroid follicle architecture during prolonged growth in conventional 2D culture systems is not possible. Moreover, as the follicle flattens, oocyte:granulosa cell connections are disrupted and critical bi-directional communications between the oocyte and its surrounding somatic cells are lost. Design of 3D follicle culture models has therefore been the focus of much research [87–94].
The challenge of maturing human follicles in vitro and creating competent oocytes may take years to accomplish and will be fueled by our growing understanding of the complex interactions between the oocyte and its supporting granulosa and thecal cell components. The successful culture of human follicles in vitro will ultimately herald a new age in reproductive medicine and the treatment of infertility.
2.6 Male Gametogenesis
Male gametogenesis or spermatogenesis is a temporal event whereby relatively undifferentiated germ cell called spermatogonia slowly evolves into a highly specialized testicular spermatozoa over a span of several weeks. Spermatogenesis takes place in the testis. Spermatozoa are transported to the epididymis where they attain maturity and motility before being released into the seminal ejaculate along with the other accessory gland secretions. In this section we will cover the following topics:
- 1.
Organization of the testis, describe the structure of the testis and function mainly the production of hormone and the spermatozoa and the role of supporting cells, i.e. Leydig cells and the Sertoli cells.
- 2.
Define the terms and explain the process of spermatogenesis , the main steps involved explain the types of spermatogonia , spermatocytogenesis and the processes of mitosis and meiosis, spermiogenesis, nuclear development, release of spermatozoa in the lumen or spermiation . Explain what is the cycle or wave of seminiferous epithelium and efficiency of spermatogenesis.
- 3.
Describe the structure of spermatozoa.
- 4.
Explain the regulation of spermatogenesis , difference between the intrinsic regulation and extrinsic influences on spermatogenesis.
- 5.
Epididymis and its role in storage and maturation of sperm.
- 6.
Process of sperm entry into cervical mucus, physiological process of capacitation and acrosome reaction and subsequent fertilization .
- 7.
The new 2010 World Health Organization Guidelines and concluding remarks
Clinical Case
A 41-year-old male presents with his partner for infertility of 1 year duration. He is referred because his sperm count is low and his FSH is high but his testosterone is normal. He asks if there is anything you can do.
2.7 Organization of the Testis
The testes are ellipsoid in shape, measuring 2.5 × 4 cm in diameter and engulfed by a capsule (tunica albuginea ) of strong connective tissue [95]. Along its posterior border, the testis is loosely connected to the epididymis, which gives rise to the vas deferens at its lower pole [96]. The testis has two main functions : it produces hormones, in particular testosterone, and it produces the male gamete—the spermatozoa. The spermatozoa express unique antigens that are not formed until puberty. The blood–testis barrier develops as these autoantigens develop. The blood–testis barrier makes the testis an immunologically privileged site. The testis is incompletely divided into a series of lobules. Most of the volume of the testis is made up of seminiferous tubules , which are looped or blind-ended and packed in connective tissue within the confines of the fibrous septae (see ◘ Fig. 2.5). The fibrous septae divide the parenchyma into about 370 conical lobules consisting of the seminiferous tubules and the intertubular tissue. The seminiferous tubules are separated by groups of Leydig cells, blood vessels, lymphatics, and nerves. The seminiferous tubules are the site of sperm production. The wall of each tubule is made up of myoid cells of limited contractility and also of fibrous tissue. Each seminiferous tubule is about 180 μm in diameter, the height of the germinal epithelium measures 80 μm, and the thickness of the peritubular tissue is about 8 μm. The germinal epithelium consists of cells at different stages of development located within the invaginations of Sertoli cells, namely, spermatogonia , primary and secondary spermatocytes, and spermatids. Both ends of the seminiferous tubules open into the spaces of the rete testis. The fluid secreted by the seminiferous tubules is collected in the rete testis and delivered in the ductal system of the epididymis.


Fig. 2.5
The human testis and the epididymis . The testis shows the tunica vaginalis and tunica albuginea , seminiferous tubule septae, rete testis, and the overlying head, body, and tail of the epididymis. To the left is a diagrammatic representation of a fully mature spermatozoon (All Rights Reserved Sperm Chromatin, ed. Zini A and Agarwal A, Biological and Clinical applications in Male Infertility and Assisted Reproduction, Springer Science + Business Media 2011)
2.7.1 Supporting Cells
The supporting cells of the testes refer to cells that are part of the cellular development that leads to a mature sperm. The two most important cells are the Leydig and Sertoli cells.
2.7.2 Leydig Cells
The Leydig cells are irregularly shaped cells with granular cytoplasm present individually or more often in groups within the connective tissue [97, 98]. Leydig cells are the prime source of the male sex hormone testosterone. The pituitary hormone, luteinizing hormone (LH) , acts on Leydig cells to stimulate the production of testosterone. This acts as a negative “feedback” on the pituitary to suppress or modulate further LH secretion. Compared with the testosterone levels in the blood, the intratesticular concentration of testosterone is many times higher, especially near the basement membrane of the seminiferous tubule .
2.8 The Sertoli Cell
The seminiferous tubules are lined with highly specialized Sertoli cells that rest on the tubular basement membrane and extend into the lumen with a complex ramification of cytoplasm (see ◘ Fig. 2.6). Spermatozoa are produced at puberty, but are not recognized by the immune system that develops during the first year of life. The seminiferous tubule space is divided into basal (basement membrane) and luminal (lumen) compartments by strong intercellular junctional complexes called “tight junctions .” These anatomic arrangements, complemented by closely aligned myoid cells that surround the seminiferous tubule, form the basis for the blood–testis barrier. The blood–testis barrier provides a microenvironment for spermatogenesis to occur in an immunologically privileged site. Sertoli cells serve as “nurse” cells for spermatogenesis, nourishing germ cells as they develop. These also participate in germ cell phagocytosis. Multiple sites of communication exist between Sertoli cells and developing germ cells for the maintenance of spermatogenesis within an appropriate hormonal milieu. FSH binds to the high-affinity FSH receptors found on Sertoli cells signaling the secretion of androgen-binding protein. High levels of androgens are also present within the seminiferous tubule.


Fig. 2.6
Section of the germinal epithelium in the seminiferous tubule . Sertoli cells divide the germinal epithelium into a basal and adluminal compartment, via the Sertoli cell. Spermatozoa are released into the lumen (All Rights Reserved Sperm Chromatin, ed. Zini A and Agarwal A, Biological and Clinical applications in Male Infertility and Assisted Reproduction, Springer Science + Business Media 2011)
The two most important hormones secreted by the Sertoli cells are AMH and inhibin. AMH is a critical component of embryonic development and is involved in the regression of the Müllerian ducts. Inhibin is a key macromolecule in pituitary FSH regulation. Some of the functions of the Sertoli cell are (1) maintenance of integrity of seminiferous epithelium, (2) compartmentalization of seminiferous epithelium, (3) secretion of fluid to form tubular lumen to transport sperm within the duct, (4) participation in spermiation , (5) phagocytosis and elimination of cytoplasm, (6) delivery of nutrients to germ cells, (7) steroidogenesis and steroid metabolism, (8) movement of cells within the epithelium, (9) secretion of inhibin and androgen-binding protein, (10) regulation of spermatogenic cycle, and (11) providing a target for hormones LH, FSH, and testosterone receptors present on Sertoli cells .
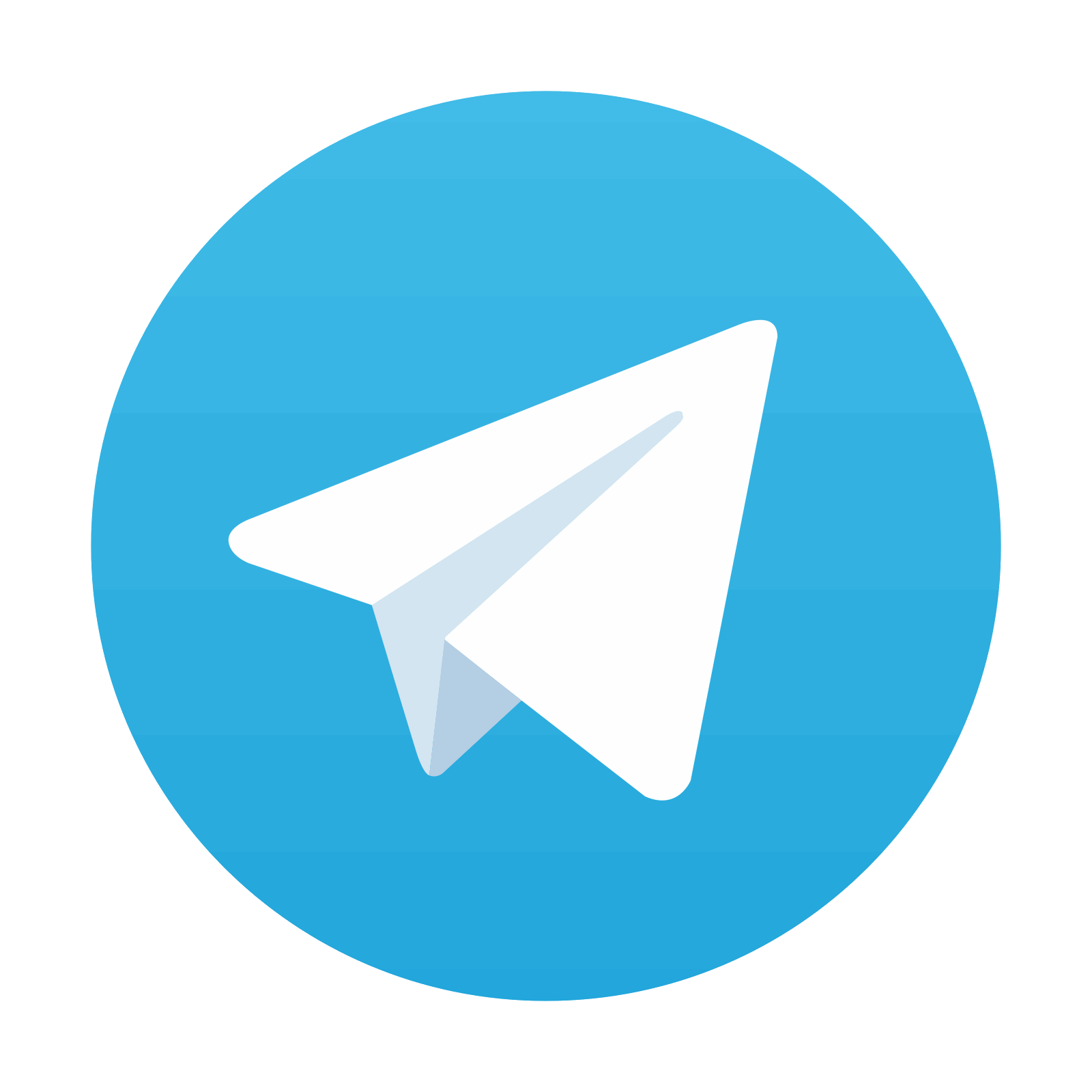
Stay updated, free articles. Join our Telegram channel

Full access? Get Clinical Tree
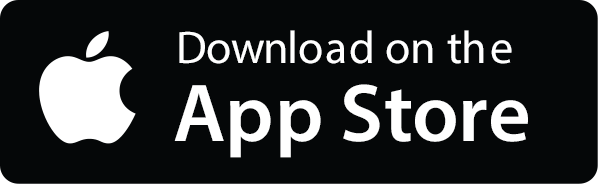
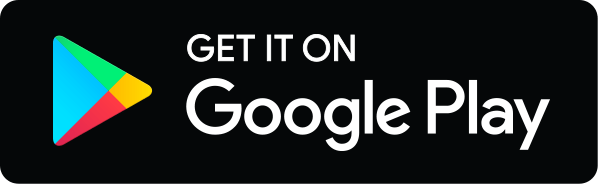