Fig. 1.1
The hypothalamic-pituitary-gonadal axis. The hypothalamus signals the anterior pituitary to release LH and FSH to, respectively, stimulate testosterone production and spermatogenesis in the testicles. This system is regulated through feedback inhibition
Infertility caused by failure of the HPG axis is termed hypogonadotropic hypogonadism or secondary testicular failure. This implies that a lack of gonadotropic activity is the source of decreased testicular function. The etiology of endocrine dysfunction may be congenital or acquired with the latter occurring in cancer.
The function of the HPG axis can be inhibited by factors produced by tumors. Human chorionic gonadotropin (hCG) is a tumor marker that is produced by testicular germ cell tumors. As this hormone shares a similar chemical structure with the hormones produced by the anterior pituitary, hCG may affect fertility indirectly through the negative feedback loop. Testicular cancer patients with elevated hCG exhibit lower LH, FSH, and motile sperm count compared to those with normal hCG [1]. Alpha-fetoprotein has also been associated with decreased total sperm count in patients with nonseminomatous testicular cancer [2]. The impact of these tumor markers on fertility is not fully understood as other studies demonstrate that there may not be an association between tumor markers and spermatogenesis [3].
An excess of physiologic factors can also inhibit the HPG axis. Prolactin, produced by the anterior pituitary gland, acts as an inhibitory factor to the HPG axis by limiting hypothalamic GnRH secretion. Hyperprolactinemia has been associated with sexual dysfunction and premature ejaculation [4]. Hyperprolactinemia can occur in cancer, such as from a functional prolactinoma, or as a side effect of treatment. Nasopharyngeal and intracerebral non-pituitary tumors are treated by radiation with potential impact upon the pituitary gland and hypothalamus. Hyperprolactinemia has been identified in patients treated with radiotherapy directed at head and neck cancers through suspected neurovascular damage and demyelination of cranial structures [5, 6]. Radiation injury to these structures has also been reported with decreased function at gonadotrophs as well as somatotrophs and corticotrophs [5–7]. These patients can exhibit signs of gonadotroph dysfunction with reduced levels of LH and FSH [5–7] and the symptoms can worsen with concurrent chemotherapy [6].
Testosterone Production and Function
Testosterone significantly contributes to the development and function of the male reproductive system. LH stimulates Leydig cells in the testicle. Testosterone is then released into systemic circulation, where it is bound by sex-hormone-binding globulin and albumin until it is released to act on target tissues. Testosterone is converted to dihydrotestosterone by 5α-reductase. These two androgens play a significant role in sexual development and maturation throughout embryonic development, puberty, and adulthood.
As Leydig cells produce testosterone, androgen-binding protein sequesters this hormone to maintain intratesticular testosterone levels that are 40–100 times higher than serum levels [8]. Intratesticular testosterone then binds androgen receptors in Sertoli cells to promote spermatogenesis [9]. The HPG axis further contributes to this process as FSH determines the expression of androgen receptors in Sertoli cells [10]. Changes to the hormonal environment within the testicle can impact spermatogenesis. Suppression of intratesticular testosterone levels can decrease sperm count by up to 98% [8]. Knockout mice without Sertoli cell androgen receptors are infertile and demonstrate arrested spermatogenesis, decreased spermatocyte and spermatid production, and increased germ cell apoptosis [11–13].
Gonadotoxic chemotherapy can damage Leydig cells. Patients treated with chemotherapy for testicular cancer [14, 15] and lymphoma [16] can have decreased testosterone levels and corresponding increases in LH. Long-term follow-up in men treated for childhood Hodgkin’s lymphoma demonstrates normalization of testosterone levels but increased LH levels, which suggests permanent Leydig cell damage and higher LH requirements [17].
The Germinal Epithelium
The long, convoluted seminiferous tubules coiled within the testicle serve as the site of spermatogenesis. The lumen of these tubules is surrounded by the germinal epithelium (Fig. 1.2), which is comprised of columnar Sertoli cells anchored to the basement membrane and germ cells. These germ cells are located between Sertoli cells at varying stages in development as spermatogonia, spermatocytes, and spermatids. The population of Sertoli cells directly correlates with sperm production [18] as they provide structural and nutritional support to developing spermatozoa.


Fig. 1.2
The germinal epithelium. The germinal epithelium within the seminiferous tubules acts as the site of spermatogenesis. Sperm precursors (spermatogonia, spermatocytes, spermatids) are sandwiched between Sertoli cells and gradually move toward the lumen of the seminiferous tubules as they progress through development
Sertoli cells are interlocked by tight junctions forming the blood-testis barrier. This barrier divides the germinal epithelium into basal and adluminal compartments. The adluminal compartment contains developing spermatozoa in an environment that is sealed by the blood-testis barrier. This limits the entry of ions, metabolites, and potentially toxic substances from systemic circulation and also protects spermatozoa from the immune system. After spermatocytes undergo genetic recombination during meiosis, they display antigens that are no longer in concordance with those of host tissue. This makes developing spermatozoa appear foreign to the immune system and therefore vulnerable to being attacked. The blood-testis barrier serves an important function by creating an immune-privileged site to protect spermatozoa from the immune response.
Hormonal signaling from FSH promotes seminiferous tubule growth, protein production, and spermatogenesis. The production of spermatozoa starts with a diploid spermatogonium germ cell that undergoes a series of mitotic and meiotic divisions to ultimately produce four haploid spermatozoa. These cells migrate from the basement membrane toward the lumen of the seminiferous tubules as division and development progress from spermatogonium to spermatocytes to spermatids. Spermatids then undergo spermiogenesis to differentiate into a reproductive cell specialized for motility and fertilization. These newly transformed spermatozoa are finally shed into the lumen of the seminiferous tubules and deposited into the epididymis. Spermatozoa then undergo structural and functional changes to complete maturation within the epididymis [19]. Spermatozoa dysfunction can occur in patients with cancer as these cells are sensitive to genetic disruption, oxidative damage, and immunologic response. A more extensive description of the structural and functional integrity of spermatozoa can be found in Chap. 2.
Primary testicular failure occurs when parenchymal tissue within the testicle is no longer able to produce sperm or testosterone. This is also known as hypergonadotropic hypogonadism as the HPG axis produces FSH and LH at elevated levels due to a lack of feedback inhibition from a nonfunctional testicle. Infertility from primary testicular failure can result from baseline testicular dysfunction, pathologic disruption from cancer, or direct damage to or removal of reproductive tissue from cancer treatment.
Baseline Testicular Dysfunction
Sperm production depends on both the quality and quantity of testicular tissue. The quality of testicular tissue can be altered in men with poor testicular development. Cryptorchidism represents a failure of testicular descent that can affect testicular development. This congenital anomaly has been identified as a risk factor for both impaired fertility [20, 21] and testicular cancer [22, 23] regardless of the location of the testis and whether cryptorchidism was unilateral or bilateral.
There is a small window of opportunity for surgical intervention to lower the risk of cancer and maintain fertility. Prepubertal orchiopexy has been found to significantly decrease the risk of developing testicular cancer [23–25] but may be too late for fertility preservation. Biopsies of cryptorchid testes from patients ranging from 3 months to 5 years of age demonstrate a decline in fertility potential with age [20]. Fertility potential is determined by a measured spermatic index, which is calculated as number of germ cells within a given number of tubular cross sections. Regardless of whether the testis in question is located within the abdomen or the inguinal canal, there is a risk of decreased fertility by 9 months of age followed by a sharp decline in spermatic index by 15–18 months of age [20]. A separate study also demonstrated a significant difference in testicular volume at long-term follow-up in patients that underwent orchiopexy by age 2 compared to those that underwent surgery at an older age [21].
As males undergo puberty, some may develop baseline spermatogenic dysfunction with testicular dysgenesis syndrome . Testicular dysgenesis can manifest with a variation of alterations to the germinal epithelium including testicular microlithiasis, poor Sertoli cell differentiation, and a significant reduction of germ cells. In hypospermatogenesis , there is a decrease in the number of germ cells. Normal spermatogenesis can still occur but at a lower rate with a subsequent reduction in sperm count. Germinal aplasia , also known as Sertoli cell-only syndrome, exhibits a germinal epithelium with Sertoli cells but a total absence of germ cells. At the end of the spectrum, patients with end-stage testes have scarred germinal epithelium with a thickened basement membrane and no viable Sertoli or germ cells.
Although the neoplastic process and multidisciplinary treatment of testicular cancer may impair fertility, a proportion of men with testicular cancer may already have underlying testicular dysgenesis and impaired fertility. Testicular biopsies of men with testicular dysgenesis syndrome have demonstrated both carcinoma in situ and reduced sperm counts [26, 27]. Spermatogenesis can also be disrupted in the contralateral testis . Biopsies of the contralateral testis at the time of orchiectomy demonstrate impaired spermatogenesis and dysgenic features, such as immature tubules, microcalcifications, and Sertoli cell-only patterns [3, 28, 29].
Spermatogenesis can also be influenced by the quantity of viable reproductive tissue. Decreased testicular volume is associated with lower sperm concentration and proportion of sperm with normal morphology or forward motility [30]. Comparison of semen analyses before and after radical orchiectomy demonstrates a statistically significant decrease in sperm count in patients with nonseminomatous and seminomatous testicular germ cell tumors [31–33]. After orchiectomy, the degree of sperm count decline may be correlated with higher tumor stage and history of cryptorchidism [34]. Orchiectomy does not appear to permanently affect testosterone production although there is a corresponding increase in LH and FSH as well as a decrease in inhibin. Compensatory testicular hypertrophy in the remaining testicle and normal spermatogenesis have both been demonstrated within 2–3 years following orchiectomy in patients with early-stage seminoma despite the initial negative impact on fertility [32].
Systemic and Local Disruption from Cancer
The temperature of the scrotum is physiologically cooler than core body temperature. Heat exchange through vasculature, evaporative heat loss through scrotal skin, and positional changes via the cremasteric reflex maintain this temperature gradient. High-grade fevers of 39–40 °C can cause a temporary change in sperm concentration, total count, and motility [35, 36]. Systemic inflammation in cancer can clinically manifest as constitutional symptoms or the B-symptoms of fevers, night sweats, and weight loss associated with lymphoma. Patients with Hodgkin’s lymphoma experiencing B-symptoms have elevated erythrocyte sedimentation rates suggestive of inflammation. These patients have poor sperm quality on semen analysis prior to treatment [37, 38] and there is a dose-dependent effect by which fever affects sperm count and motility [38].
Scrotal temperature can also rise as a result of altered blood flow in addition to changes in core body temperature. Doppler ultrasound has demonstrated hypervascularity at cancerous lesions in men with lymphoma [39]. Venous drainage may also be impaired by tumors. Varicoceles may arise when pelvic masses, retroperitoneal masses, or an intravascular thrombus cause compression or obstruction of the testicular vein, renal vein, or inferior vena cava. As decreased venous drainage limits blood flow and countercurrent heat exchange, patients with varicoceles may experience increased scrotal temperature [40]. Varicoceles have been linked with infertility as sperm morphology, motility, and concentration levels are affected in men with varicoceles and elevated scrotal temperature [40, 41]. Sperm protein expression may also be affected in high-grade varicoceles and improvements in sperm motility and concentration have been demonstrated following varicocelectomy [42].
Local changes to testicular parenchyma and altered semen analysis can occur in patients with testicular cancer. Histological evaluation of surgical specimens after orchiectomy demonstrates that tumors can cause local structural damage prior to orchiectomy. The degree of parenchymal disruption has been associated with larger tumor size [3], close vicinity to the tumor [43, 44], and malignant tumors [44]. The subtype of testicular germ cell tumor may also influence spermatogenesis. Both seminomatous and nonseminomatous testicular cancers were found to have a significant impact on semen parameters. Some studies have identified lower sperm concentration [34] and total count [2] in patients with seminomatous cancers compared to those with nonseminomatous ones. In contrast, other studies have found that patients with nonseminomatous tumors have greater alterations in sperm concentration, morphology, and motility [33, 45].
Patients with lymphoma and leukemia also demonstrate changes in semen parameters prior to treatment. Altered sperm concentration, motility, and morphology have been reported in patients with Hodgkin’s lymphoma [46–49], non-Hodgkin’s lymphoma (NHL) [48], and leukemia [50]. Patients with NHL demonstrate better sperm quality with regard to motility and morphology on pretreatment semen analysis compared to those with Hodgkin’s lymphoma [51]. Testicular size or hormone levels do not appear to affect these parameters [51]. Elevated erythrocyte sedimentation rate and more advanced disease are associated with poorer semen quality even before treatment in patients with Hodgkin’s lymphoma [49].
Gonadotoxicity from Chemotherapy
Gonadotoxic agents can damage the germinal epithelium within the seminiferous tubules. The germinal epithelium can be particularly sensitive to treatments that target rapidly dividing cells as spermatogenesis is an ongoing process in postpubertal men. This includes both the chemotherapy regimens (Table 1.1) and radiation treatments used in treating cancer.
Table 1.1
Chemotherapy regimens
Regimen | Agents | Indication |
---|---|---|
CHOP | Cyclophosphamidea Doxorubicin (Hydroxydaunorubicin) Vincristine (Oncovin) Prednisone | Non-Hodgkin’s lymphoma |
ABVD | Doxorubicin (Adriamycin) Bleomycin Vinblastine Dacarbazine | Hodgkin’s lymphoma |
MOPP/MVPP | Mechlorethaminea Vincristine (Oncovin) Procarbazinea Prednisone | Hodgkin’s lymphoma |
BEP | Bleomycin Etopisode Ciplatin | Testicular cancer |
The mechanism of various chemotherapy agents is to target a particular stage in the cell cycle to prevent cells from replicating, regardless of whether these cells belong to malignant tumors or benign host tissue. The germinal epithelium can be especially sensitive to chemotherapy. The damage inflicted upon germ cells and Sertoli cells becomes evident in the sperm count, motility, and altered morphology at 3 months to 1 year after the initiation of chemotherapy [52–54]. This corresponds to the 2–3-month cycle of spermatogenesis. The gonadotoxic effects of chemotherapy in lymphoma patients may be even more pronounced in patients with testicular infiltration [55] and more widespread disease compared to those with localized disease [56].
Some chemotherapy agents can be more gonadotoxic than others (Table 1.2). Alkylating agents , in particular, have been found to cause more damage to the germinal epithelium. These agents damage cells by attaching an alkyl group to DNA and interfering with replication. Testicular biopsies of patients treated with cyclophosphamide demonstrate significant damage to the germinal epithelium [57]. A dose-dependent effect on sperm concentration and gonadal damage has been demonstrated in adult males treated with alkylating agents for lymphoproliferative disorders [58, 59] and testicular cancer [60]. The gonadotoxicity of cyclophosphamide may be related to gonadal activity as a greater degree of damage has been reported in sexually mature males than in prepubertal males [58].
Table 1.2
Chemotherapy agents with high risk of gonadoxicity
Alkylating agents |
Cyclophosphamide |
Chlorambucil |
Procarbazine |
Mechlorethamine |
Busulfan |
Platinum agents |
Cisplatin |
Carboplatin |
Gonadal dysfunction may be permanent and has been demonstrated in patients previously treated with alkylating agents. Regardless of pubertal status during treatment, childhood cancer survivors treated with high doses of cyclophosphamide for sarcoma [61], leukemia, Hodgkin’s lymphoma, NHL, and testicular cancer [62, 63] are found to have persistent gonadal dysfunction with decreased sperm count at follow-up ranging from 4 to 42 years since treatment.
Chemotherapy regimens for lymphoma vary by the specific type of disease. Treatment of NHL involves CHOP (cyclophosphamide, doxorubicin (hydroxydaunorubicin), vincristine (oncovin), prednisone). Despite the use of multiple agents, the gonadotoxicity of this regimen depends on the dose of cyclophosphamide administered. As previously discussed, damage to the germinal epithelium by cyclophosphamide may be dose dependent [58, 60–63] and permanent. There is a reported 61% recovery rate by 24 months after treatment with CHOP [54].
Patients with Hodgkin’s lymphoma may be treated with either ABVD (doxorubicin (adriamycin), bleomycin, vinblastine, dacarbazine) or MOPP/MVPP (mechlorethamine, vincristine (oncovin), procarbazine, prednisone) . Treatment with ABVD does not employ the use of an alkylating agent and subsequently demonstrates less gonadotoxic effects. Patients treated with ABVD have greater sperm concentration, motility, and vitality compared to patients treated with MOPP/MVPP and CHOP [54, 56]. Although patients demonstrate temporary impairment in semen parameters, there is a greater than 90% recovery rate by 24 months after treatment [54]. In contrast, significant gonadotoxicity has been reported with MOPP/MVPP [16, 17, 54, 56, 59] as mechlorethamine and procarbazine are both alkylating agents. Patients report decreased libido during treatment and have lower testosterone levels with corresponding increases in FSH and LH [16]. Patients treated with this chemotherapy regimen demonstrate permanent gonadal damage and infertility [16, 17, 59]. Patients treated for childhood Hodgkin’s lymphoma have persistently elevated FSH and LH levels, decreased inhibin B, and decreased sperm concentration even at a median follow-up of 15 years [17].
BEP (bleomycin, etoposide, cisplatin) is the first-line chemotherapy regimen for testicular cancer. Semen analyses in patients before and after adjuvant treatment with BEP show decreased sperm count and motility [64]. Of the toxicities of this chemotherapy regimen, gonadal cell dysfunction and Leydig cell insufficiency have been specifically linked to the cumulative dose of cisplatin [15, 60]. As a platinum-based agent, cisplatin interferes with the cell cycle by binding DNA and causing strands to cross-link. Patients treated with cisplatin-based chemotherapy have dose-dependent decreases in testosterone, corresponding rises in FSH and LH, and decreased paternity rates at follow-up ranging from 15 months to 20 years [14, 15, 60].
Gonadotoxicity from Radiation
Radiation damages cells and internal DNA beyond the point of repair to prevent replication. Radiation therapy directed at the pelvis and genitalia can cause injury to the germinal epithelium in Hodgkin’s lymphoma [65] and testicular cancer [66]. Impaired spermatogenesis and semen analysis changes become increasingly apparent by 6–12 months after radiation therapy for lymphoproliferative disorders [52] and testicular cancer [52, 53]. Gonadotoxicity may be avoided at lower levels of radiation as there is no impact on sperm count or FSH levels at radiation doses less than 20 cGy [65]. Transient dose-dependent decreases in sperm count and corresponding increases in FSH in patients with Hodgkin’s lymphoma [65] and testicular seminoma [67] can occur at doses between 20 and 100 cGy. Azoospermia has been demonstrated at even higher doses of fractionated testicular radiation at 118–228 cGy [68].
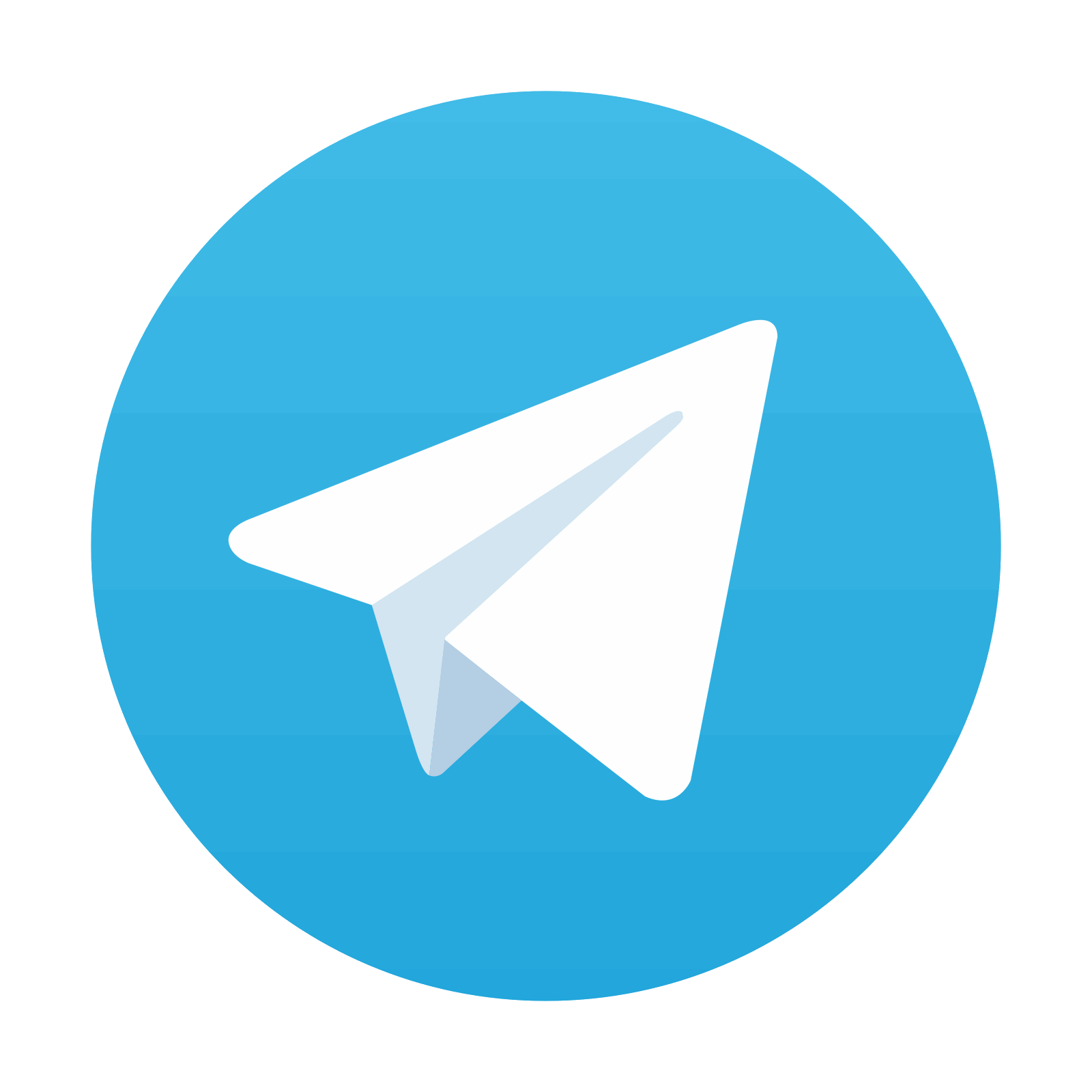
Stay updated, free articles. Join our Telegram channel

Full access? Get Clinical Tree
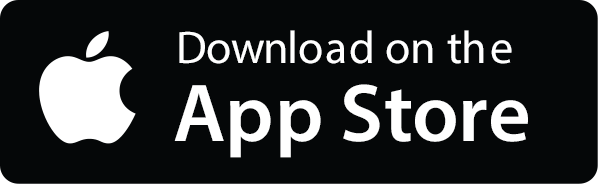
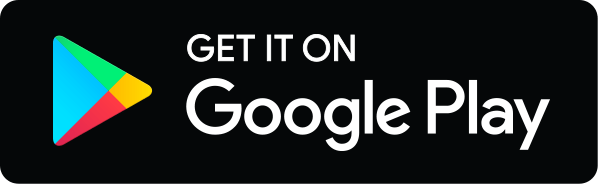