Jessie Dorais
C. Matthew Peterson
The endocrine disorders encountered most frequently in gynecologic patients are those related to disturbances in the regular occurrence of ovulation and accompanying menstruation. The most prevalent are those characterized by androgen excess, often with insulin resistance, including what is arguably the most common endocrinopathy in women—polycystic ovary syndrome (PCOS). Other conditions leading to ovulatory dysfunction, hirsutism, or virilization, and common disorders of the pituitary and thyroid glands associated with reproductive abnormalities, are reviewed in this chapter.
Hyperandrogenism
Hyperandrogenism most often presents as hirsutism, which arises as a result of androgen excess related to abnormalities of function in the ovary or adrenal glands, constitutive increase in expression of androgen effects at the level of the pilosebaceous unit, or a combination of the two. By contrast, virilization is rare and indicates marked elevations in androgen levels. An ovarian or adrenal neoplasm that may be benign or malignant commonly causes virilization.
Hirsutism
Hirsutism, the most frequent manifestation of androgen excess in women, is defined as excessive growth of terminal hair in a male distribution. This refers particularly to midline hair, side burns, moustache, beard, chest or intermammary hair, and inner thigh and midline lower back hair entering the intergluteal area. The response of the pilosebaceous unit to androgens in these androgen responsive areas transforms vellus hair (fine, nonpigmented, short) that is normally present into terminal hair (coarse, stiff, pigmented, and long).
Androgen effects on hair vary in relation to specific regions of the body surface. Hair that shows no androgen dependence includes lanugo, eyebrows, and eyelashes. The hair of the limbs and portions of the trunk exhibits minimal sensitivity to androgens. Pilosebaceous units of the axilla and pubic region are sensitive to low levels of androgens, such that the modest androgenic effects of adult levels of androgens of adrenal origin are sufficient for substantial expression of terminal hair in these areas. Follicles in the distribution associated with male patterns of facial and body hair (midline, facial, inframammary) require higher levels of androgens, as seen with normal testicular function or abnormal ovarian or adrenal androgen production. Scalp hair is inhibited by gonadal androgens, in varying degrees, as determined by age and genetic determination of follicular responsiveness, resulting in the common frontal-parietal balding seen in some males and in virilized females. Hirsutism results from both increased androgen production and skin sensitivity to androgens. Skin sensitivity depends on the genetically determined local activity of 5α-reductase, the enzyme that converts testosterone to dihydrotestosterone (DHT), the bioactive androgen in hair follicles.
Hair demonstrates cyclic activity between growth (anagen), involution (catagen), and resting (telogen) phases. The durations of both the growth and resting phases vary according to region of the body, genetic factors, age, and hormonal effects. The cycles of growth, rest, and shedding are normally asynchronous, but when synchronous entry into telogen phase is triggered by major metabolic or endocrine events, such as pregnancy and delivery, or severe illness, dramatic (although transient) hair loss may occur in the following months (telogen effluvium).
Hirsutism is a relative, rather than absolute, designation. What is normal in one setting may be considered abnormal in others; social and clinical reactions to hirsutism can vary significantly, reflecting ethnic variation in skin sensitivity to androgens and cultural ideals. Androgen-dependent hair (excluding pubic and axillary hair) occurs in only 5% of premenopausal white women and is considered abnormal by white women of North America, whereas considerable facial and male pattern hair in other areas may be more common and more often considered acceptable and normal among such groups as the Inuit and women of Mediterranean background.
Hypertrichosis and Virilization
Two conditions should be distinguished from hirsutism. Hypertrichosis is the term reserved for androgen-independent terminal hair in nonsexual areas, such as the trunk and extremities. This may be the result of an autosomal-dominant congenital disorder, a metabolic disorder (such as anorexia nervosa, hyperthyroidism, porphyria cutanea tarda), or medications (e.g., acetazolamide, anabolic steroids, androgenic progestins, androgens, cyclosporine, diazoxide, dehydroepiandrosterone (DHEA), heavy metals, interferon, methyldopa, minoxidil, penicillamine, phenothiazines, phenytoin, streptomycin, reserpine, valproic acid). Virilization is a marked and global masculine transformation that includes coarsening of the voice, increase in muscle mass, clitoromegaly (normal clitoral dimensions ± standard deviation [SD] are 3.4 + 1 mm width by 5.1 + 1.4 mm length) and features of defeminization (loss of breast volume and body fat contributing to feminine body contour) (1). Although hirsutism accompanies virilization, the presence of virilization indicates a high likelihood of more serious conditions than are common with hirsutism alone and should prompt evaluation to exclude ovarian or adrenal neoplasm. Although rare, these diagnoses become likely when onset of androgen effects is rapid or sufficiently pronounced to produce the picture of virilization.
The history should focus on the age of onset and rate of progression of hirsutism or virilization. A rapid rate of progression or virilization is associated with a more severe degree of hyperandrogenism and should raise suspicion of ovarian and adrenal neoplasms or Cushing syndrome. This is true whether rapid progression or virilization occurs before, during, or after puberty. Anovulation, manifesting as amenorrhea or oligomenorrhea, increases the probability that there is underlying hyperandrogenism. Hirsutism occurring with regular cycles is more commonly associated with normal androgen levels and thus is attributed to increased genetic sensitivity of the pilosebaceous unit and is termed idiopathic hirsutism. When virilization is present, anovulation virtually always occurs.
In determining the extent of hirsutism, a sensitive and tactful approach by the physician is mandatory and should include questions regarding the use and frequency of shaving and/or chemical or mechanical depilatories. Typically, clinical evaluation of the degree of hirsutism is subjective. Most physicians arbitrarily classify the degree of hirsutism as mild, moderate, or severe. Objective assessment is helpful, especially in establishing a baseline from which therapy can be evaluated. The Ferriman–Gallwey Scoring System for Hirsutism quantitates the extent of hair growth in the most androgen sensitive sites. It is a scoring scale of androgen-sensitive hair in nine body areas rated on a scale of 0 to 4 (2). A total score higher than 8 is defined as hirsutism (Fig. 31.1) (3). Although widely used, this scoring system has limitations, one of which is the fact that the scale does not include the sideburn, buttocks, and perineal areas. Substantial hirsutism may be confined to one or two areas without exceeding the cutoff value in total hirsutism score. The score does not reflect the extent to which hirsutism affects a woman’s well being (3,4).
Figure 31.1 Ferriman-Gallwey hirsutism scoring system. Each of the nine body areas most sensitive to androgen is assigned a score from 0 (no hair) to 4 (frankly virile), and these separate scores are summed to provide a hormonal hirsutism score. (Reproduced from Hatch R, Rosefield RL, Kim MH, et al. Hirsutism: implications, etiology, and management. Am J Obstet Gynecol 1981;140:815–830. ©Elsevier.)
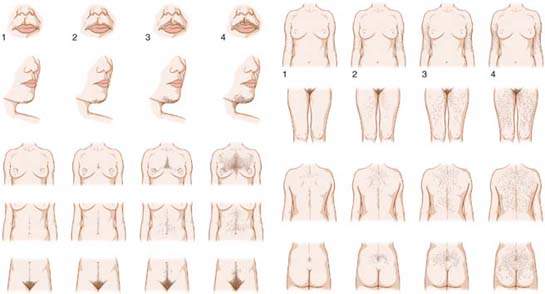
Figure 31.2 Major steroid biosynthesis pathway.
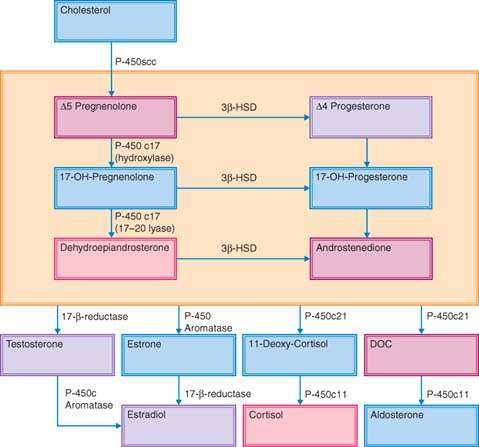
A family history should be obtained to disclose evidence of idiopathic hirsutism, PCOS, congenital or adult onset adrenal hyperplasia (CAH or AOAH), diabetes mellitus, and cardiovascular disease. A history of drug use should be obtained. In addition to drugs that commonly cause hypertrichosis, anabolic steroids and testosterone derivatives may cause hirsutism and even virilization. During the physical examination, attention should be directed to obesity, hypertension, galactorrhea, male-pattern baldness, acne (face and back), and hyperpigmentation. With virilization, the presence of an androgen-producing ovarian neoplasm or Cushing syndrome must be considered. In many cases of Cushing syndrome, the patient’s presenting symptom is hirsutism. This devastating disorder may masquerade as other disorders such as AOAH and PCOS. Before making these diagnoses, the physician should search for the physical signs of the syndrome such as “moon face,” plethora, purple striae, dorsocervical and supraclavicular fat pads, and proximal muscle weakness. A moon-shaped face, upper body obesity, muscle weakness, and the development of a pad of fat between the shoulder blades are particularly notable to both patients and diagnosticians considering the diagnosis of Cushing syndrome.
Role of Androgens
Androgens and their precursors are produced by both the adrenal glands and the ovaries in response to their respective trophic hormones, adrenocorticotropic hormone (ACTH) and luteinizing hormone (LH), respectively (Fig. 31.2). Biosynthesis begins with the rate-limiting conversion of cholesterol to pregnenolone by side-chain cleavage enzyme. Thereafter, pregnenolone undergoes a two-step conversion to the 17-ketosteroid DHEA along the Δ-5 steroid pathway. This conversion is accomplished by CYP17, an enzyme with both 17α-hydroxylase and 17,20-lyase activities. In a parallel fashion, progesterone undergoes transformation to androstenedione in the Δ-4 steroid pathway. The metabolism of Δ-5 to Δ-4 intermediates is accomplished via a Δ-5-isomerase, 3β-hydroxysteroid dehydrogenase (3β-HSD).
Adrenal 17-Ketosteroids
Secretion of adrenal 17-ketosteroids increases prepubertally and independently of pubertal maturation of the hypothalamic–pituitary–ovarian axis. This alteration in adrenal steroid secretion is termed adrenarche and is characterized by a dramatic change in the response of the adrenal cortex to ACTH and with preferential secretion of Δ-5 steroids, including 17-hydroxypronenolone, DHEA, and dehydroepiandrosterone sulfate (DHEAS). The basis for this action is related to the increase in the zona reticularis and in the increased activity of the 17-hydroxylase and the 17,20-lyase enzymes. Independent of the increase in ovarian androgen secretion accompanying puberty, the increase in adrenal androgens owing to adrenarche can account for significant increases in pubic and axillary hair and sweat production by the axillary pilosebaceous units.
Testosterone
Approximately half of a woman’s serum testosterone is derived from peripheral conversion of secreted androstenedione and the other half is derived from direct glandular (ovarian and adrenal) secretion. The ovaries and adrenal glands contribute almost equally to testosterone production in women. The contribution of the adrenals is achieved primarily through secretion of androstenedione.
Approximately 66% to 78% of circulatory testosterone is bound to sex hormone–binding globulin (SHBG) and is considered biologically inactive. Most of the proportion of serum testosterone that is not bound to SHBG is weakly associated with albumin (20% to 32%). A small percentage (1% to 2%) of testosterone is entirely unbound or free. The fraction of circulating testosterone that is unbound by SHBG has an inverse relationship with the SHBG concentration. Increased SHBG levels are noted in conditions associated with high estrogen levels. Pregnancy, the luteal phase, use of estrogen (including oral contraceptives), and conditions causing elevated thyroid hormone levels and cirrhosis of the liver are associated with reduced fractions of free testosterone caused by elevated SHBG levels. Conversely, levels of SHBG decrease and result in elevated free testosterone fractions in response to androgens, androgenic disorders (PCOS, adrenal hyperplasia or neoplasm, Cushing syndrome), androgenic medications (i.e., progestational agents with androgenic biologic activities, such as danazol, glucocorticoids, and growth hormones), hyperinsulinemia, obesity, and prolactin.
Laboratory Assessment of Hyperandrogenemia
In hyperandrogenic states, increases in testosterone production are not proportionately reflected in increased total testosterone levels because of the depression of SHBG levels that occurs concomitant with increasing androgen effects on the liver. Therefore, when moderate hyperandrogenism, characteristic of many functional hyperandrogenic states, occurs, elevations in total testosterone levels may remain within the normal range, and only free testosterone levels will reveal the hyperandrogenism. Severe hyperandrogenism, as occurs in virilization and that results from neoplastic production of testosterone, is reliably detected by measures of total testosterone. Therefore, in practical clinical evaluation of the hyperandrogenic patient, determination of the total testosterone level in concert with clinical assessment is frequently sufficient for diagnosis and management. When more precise delineation of the degree of hyperandrogenism is desired, measurement or estimation of free testosterone levels can be undertaken and will more reliably reflect increases in testosterone production. These measurements are not necessary in evaluating the majority of patients, but they are common in clinical research studies and may be useful in some clinical settings. Because many practitioners measure some form of testosterone level, they should understand the methods used and their accuracy. Although equilibrium dialysis is the gold standard for measuring free testosterone, it is expensive, complex, and usually limited to research settings. In a clinical setting, free testosterone levels can be estimated by assessment of testosterone binding to albumin and SHBG.
Testosterone, that is nonspecifically bound to albumin (AT), is linearly related to free testosterone (FT) by the equation:
AT = Ka [A] × FT,
where AT is the albumin-bound testosterone, Ka is the association constant of albumin for testosterone, and [A] is the albumin concentration.
In many cases of hirsutism, albumin levels are within a narrow physiologic range and thus do not significantly affect the free testosterone concentration. When physiologic albumin levels are present, the free testosterone level can be estimated by measuring the total testosterone and SHBG. In individuals with normal albumin levels, this method has reliable results compared with those of equilibrium dialysis. It provides a rapid, simple, and accurate determination of the total and calculated free testosterone level and the concentration of SHBG.
The bioavailable testosterone level is based on the relationship of albumin and free testosterone and incorporates the actual albumin level with the total testosterone and SHBG. This combination of total testosterone, SHBG, and albumin level measurements can be applied to derive a more accurate estimate of available bioactive testosterone and thus the androgen effect derived from testosterone. Bioactive testosterone determined in this manner provides a superior estimate of the effective androgen effect derived from testosterone (5).
Pregnancy can alter the accuracy of measurements of bioavailable testosterone. During pregnancy, estradiol, which shares with testosterone a high affinity for SHBG, occupies a large proportion of SHBG binding sites, so that measurement of SHBG levels can overestimate the binding capacity of SHBG for testosterone. Derived estimates of free testosterone, as opposed to direct measure by equilibrium dialysis, are therefore inaccurate during pregnancy. Testosterone measurements in pregnancy are primarily of interest when autonomous secretion by tumor or luteoma is in question, and for these, total testosterone determinations provide sufficient information for diagnosis.
For testosterone to exert its biologic effects on target tissues, it must be converted into its active metabolite, DHT, by 5α-reductase (a cytosolic enzyme that reduces testosterone and androstenedione). Two isozymes of 5α-reductase exist: type 1, which predominates in the skin, and type 2, or acidic 5α-reductase, which is found in the liver, prostate, seminal vesicles, and genital skin. The type 2 isozyme has a 20-fold higher affinity for testosterone than type 1. Both type 1 and 2 deficiencies in males result in ambiguous genitalia, and both isozymes may play a role in androgen effects on hair growth. Dihydrotestosterone is more potent than testosterone, primarily because of its higher affinity and slower dissociation from the androgen receptor. Although DHT is the key intracellular mediator of most androgen effects, measurements of circulating levels are not clinically useful.
The relative androgenicity of androgens is as follows:
Until adrenarche, androgen levels remain low. Around 8 years of age, adrenarche is heralded by a marked increase in DHEA and DHEAS. The half-life of free DHEA is extremely short (about 30 minutes) but extends to several hours if DHEA is sulfated. Although no clear role is identified for DHEAS, it is associated with stress and levels decline steadily throughout adult life. After menopause, ovarian estrogen secretion ceases, and DHEAS levels continue to decline, whereas testosterone levels are maintained or may even increase. Although postmenopausal ovarian steroidogenesis contributes to testosterone production, testosterone levels retain diurnal variation, reflecting an ongoing and important adrenal contribution. Peripheral aromatization of androgens to estrogens increases with age, but because small fractions (2% to 10%) of androgens are metabolized in this fashion, such conversion is rarely of clinical significance.
Laboratory Evaluation
The 2008 Endocrine Society Clinical Practice Guidelines suggest testing for elevated androgen levels in women with moderate (Ferriman–Gallwey hirsutism score 9 or greater) or severe hirsutism or hirsutism of any degree when it is sudden in onset, rapidly progressive, or associated with other abnormalities such as menstrual dysfunction, infertility, significant acne, obesity, or clitoromegaly. These guidelines suggest against testing for elevated androgen levels in women with isolated mild hirsutism because the likelihood of identifying a medical disorder that would change management or outcome is extremely low (Fig. 31.3) (4). Medications that cause hirsutism are listed and should be considered (Table 31.1).
When laboratory testing for the assessment of hirsutism is indicated, either a bioavailable testosterone level (includes a total testosterone, SHBG, and albumin level) or a calculated free testosterone level (if albumin levels are assumed to be normal) provides the most accurate assessment of the androgen effect derived from testosterone. In clinical situations requiring a testosterone evaluation, the addition of 17-hydroxyprogesterone will screen for adult onset adrenal hyperplasia, when indicated (Table 31.2). When hirsutism is accompanied by absent or abnormal menstrual periods, assessment of prolactin and thyroid-stimulating hormone (TSH) values are required to diagnose an ovulatory disorder. Hypothyroidism and hyperprolactinemia may result in reduced levels of SHBG and may increase the fraction of unbound testosterone levels, occasionally resulting in hirsutism. In cases of suspected Cushing syndrome, patients should undergo screening with a 24-hour urinary cortisol (most sensitive and specific) assessment or an overnight dexamethasone suppression test. For this test, the patient takes 1 mg of dexamethasone at 11 p.m., and a blood cortisol assessment is performed at 8 a.m. the next day. Cortisol levels of 2 μg/dL or higher after overnight dexamethasone suppression require a further workup for evaluation of Cushing syndrome. Elevated 17-hydroxyprogesterone (17-OHP) levels identify patients who may have AOAH, found in 1% to 5% of hirsute women. The 17-OHP levels can vary significantly within the menstrual cycle, increasing in the periovulatory period and luteal phase, and may be modestly elevated in PCOS. Standardized testing requires early morning testing during the follicular phase.
According to the Endocrine Society clinical guidelines, patients with morning follicular phase 17-OHP levels of less than 300 ng/dL (10 nmol/L) are likely unaffected. When levels are greater than 300 ng/dL but less than 10,000 ng/dL (300 nmol/L), ACTH testing should be performed to distinguish between PCOS and AOAH. Levels greater than 10,000 ng/dL (300 nmol/L) are virtually diagnostic of congenital adrenal hyperplasia.
Precocious pubarche precedes the diagnosis of adult onset congenital adrenal hyperplasia in 5% to 20% of cases. Measurement of 17-OHP should be performed in patients presenting with precocious pubarche, and a subsequent ACTH stimulation test is recommended if basal 17-OHP is greater than 200 ng/dL. A study using a 200 ng/dL threshold for basal 17-OHP plasma levels to prompt ACTH stimulation testing offered 100% (95% confidence interval [CI], 69–100) sensitivity and 99% (95% CI, 96–100) specificity for the diagnosis of adult onset congenital adrenal hyperplasia within the cohort with precocious puberty (6).
Because increased testosterone production is not reliably reflected by total testosterone levels, the clinician may choose to rely on typical male pattern hirsutism as confirmation of its presence, or may elect measures that reflect levels of free or unbound testosterone (bioavailable or calculated free testosterone levels). Total testosterone does serve as a reliable marker for testosterone-producing neoplasms. Total testosterone levels greater than 200 ng/dL should prompt a workup for ovarian or adrenal tumors.
Although the ovary is the principal source of androgen excess in most of PCOS patients, 20% to 30% of patients with PCOS will demonstrate supranormal levels of DHEAS. Measuring circulating levels of DHEAS has limited diagnostic value, and overinterpretation of DHEAS levels should be avoided (7).
In the past, testing for androgen conjugates (e.g., 3α-androstenediol G [3α-diol G] and androsterone G [AOG] as markers for 5α-reductase activity in the skin) was advocated. Routine determination of androgen conjugates to assess hirsute patients is not recommended, because hirsutism itself is an excellent bioassay of free testosterone action on the hair follicle and because these androgen conjugates arise from adrenal precursors and are likely markers of adrenal and not ovarian steroid production (8).
Figure 31.3 Evaluation of hirsute women for hyperandrogenism. Evaluation includes more than the assessment of the degree of hirsutism. When hirsutism is moderate (>9) or severe or if mild hirsutism is accompanied by features that suggest an underlying disorder, elevated androgen levels should be ruled out. Disorders to be considered include endocrinopathies, of which PCOS is the most common, and neoplasms. Plasma testosterone is best assessed in the early morning on day 4 to 10 in regularly cycling women. A 17-hydroxyprogesterone is also indicated when symptoms warrant a bioavailable testosterone measurement. *3β-hydroxysteroid dehydrogenase deficiency in severe forms presents with mineralocorticoid and cortisol deficiency. Mild forms are diagnosed with a mean post-ACTH(1-24) stimulation: 17-hydroxypregnenolone/17-hydroxyprogesterone ratio of 11 compared to 3.4 in normals. 11β-hydroxylase deficiency presents with hypertension in the first years of life in two thirds of patients. The mild form presents with vitalization or precocious puberty without hypertension. Undiagnosed adults demonstrate hirsutism, acne, and amenorrhea. Diagnosis is confirmed with an 11-desoxycortisol level >25 ng/mL 60 minutes after ACTH(1-24) stimulation. ACTH, adrenocorticotropic hormone; AOAH, adult-onset adrenal hyperplasia; DHEAS, dehydroepiandrosterone sulfate; HAIR-AN, hyperandrogenemia, insulin resistance- acanthosis nigricans. (See references 2–11,15.)
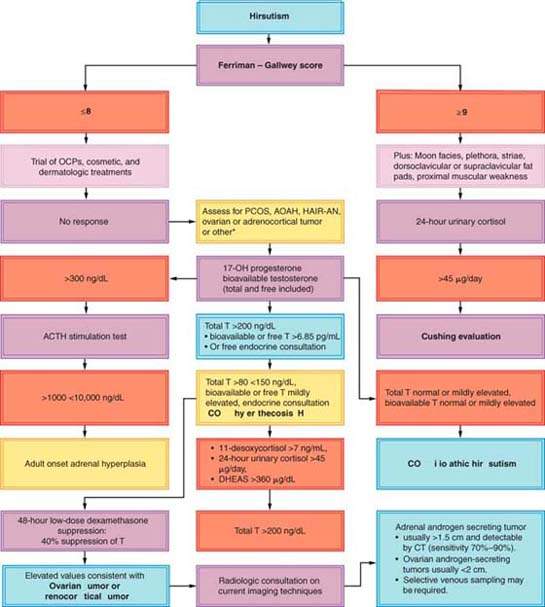
Table 31.1 Medications Associated with Hirsutism
Acetazolamide | methyldopa |
anabolic steroids | minoxidil |
androgenic progestins | penicillamine |
androgens | phenothiazines |
Cyclosporine | phenytoin |
Diazoxide | streptomycin |
DHEA | reserpine |
heavy metals | valproic acid |
Interferon |
Table 31.2 Normal Values for Serum Androgensa
Testosterone (total) | 20–80 ng/dL |
Free testosterone (calculated) | 0.6–6.8 pg/mL |
Percentage free testosterone | 0.4–2.4% |
Bioavailable testosterone | 1.6–19.1 ng/dL |
SHBG | 18–114 nmol/L |
Albumin | 3,300–4,800 mg/dL |
Androstenedione | 20–250 ng/dL |
Dehydroepiandrosterone sulfate | 100–350 μg/dL |
17-hydroxyprogesterone (follicular phase) | 30–200 ng/dL |
SHBG, sex hormone–binding globulin. aNormal values may vary among different laboratories. Free testosterone is calculated using measurements for total testosterone and sex hormone–binding globulin, whereas bioavailable testosterone is calculated using measured total testosterone, sex hormone–binding globulin, and albumin. Calculated values for free and bioavailable testosterone compare well with equilibrium dialysis methods of measuring unbound testosterone when albumin levels are normal. Bioavailable testosterone includes free plus very weakly bound (non-SHBG, nonalbumin) testosterone. Bioavailable testosterone is the most accurate assessment of bioactive testosterone in the serum without performing equilibrium dialysis. |
In the zona reticularis layer of the adrenal cortex, DHEAS is generated by SULT2A1 (9). This layer of the adrenal cortex is thought to be the primary source of serum DHEAS. DHEAS levels decline as a person ages and the reticularis layer diminishes in size. In most laboratories, the upper limit of a DHEAS level is 350 μg/dL (9.5 nmol/L). A random sample is sufficient because the level of variation is minimized as a result of the long half-life characteristic of sulfated steroids. DHEAS is used as a screen for androgen-secreting adrenocortical tumors; however, moderate elevations are a common finding in the presence of PCOS, obesity, and stress, which reduces specificity (10).
A study of women with androgen-secreting adrenocortical tumors (ACT-AS) (N = 44), compared to women with nontumor androgen excess (NTAE) (N = 102), sheds additional light on the choice of hormones used to screen for an adrenocortical tumor. In the study, the demographics and the prevalence of hirsutism, acne, oligomenorrhea and amenorrhea were not different in each group. Free testosterone (free T) was the most commonly elevated androgen in ACT-AS (94%), followed by androstenedione (A) (90%), DHEAS (82%), and total testosterone (total T) (76%), and all three androgens were simultaneously elevated in 56% of the cases. Serum androgen levels became subnormal in all ACT-AS patients after the tumor was removed. In nontumor androgen excess alone, the most commonly elevated androgen was androstenedione (93%), while all three androgens (T, A, and DHEAS) were elevated in only 22% of the cases. Free testosterone values above 6.85 pg/mL (23.6 pmol/L) had the best diagnostic value for ACT-AS (sensitivity 82%; CI, 57%–96%; specificity 97%, CI, 91%–100%) (Table 31.3). The large overlap of androstenedione, testosterone, and DHEAS levels between ACT-AS and androgen excess groups suggests that thoughtful consideration should be employed when choosing hormone studies for this evaluation (11).
Table 31.3 Sensitivity and Specificity of Basal Hormone Levels in the Evaluation of Female Patients with Androgen-secreting Adrenocortical Tumors (ACT-AS) and Nontumor Causes of Androgen Excess (NTAE)
The heterogeneity of hormone secretion patterns in the adrenocortical tumor group reveals the complexities of hormone level screening for adrenocortical tumors: 7 of 44 patients (15.9%) had tumors secreting androgens alone, 2 of 44 (4.5%) had tumors secreting androgens and estrogens, 28 of 44 (63.6%) had tumors secreting both androgens and cortisol, and 7 of 44 (15.9%) had tumors secreting androgens, cortisol, and estrogens. Compound S or 11-desoxycortisol was increased (≥10 ng/mL or 28.9 nmol/L) in 23 of 27 ACT-AS patients (85%); 20 of 21 patients with malignant tumors, and 3 of 6 patients with apparently benign tumors, although 11-desoxycortisol was normal and inferior to 6 ng/mL (17.3 nmol/L) in 35 of 35 nontumor androgen excess patients (100%). Youden’s index displayed that a 11-desoxycortisol level above 7 ng/mL (20.2 nmol/L) has a sensitivity of 89% (95% CI, 71%–98%) and a specificity of 100% (95% CI, 90%–100%) for the detection of ACT-AS (11,12).
When clinical signs of androgen excess reach the point of virilization or the free testosterone level is above 6.85 pg/mL (23.6 pmol/L), follow-up testing with a 11-desoxycortisol (>7 ng/mL), DHEAS (>3.6 μg/mL), and 24-hour urinary cortisol (>45 μg per day) are the most sensitive and specific for the detection of an androgen-secreting adrenocortical tumor. Careful consideration of the sensitivity and specificity, diurnal variation, and age-related variation of potentially measureable androgens will aid in choosing the most useful measurements (Table 31.3).
Polycystic Ovary Syndrome
PCOS is arguably one of the most common endocrine disorders in women of reproductive age, affecting 5% to 10% of women worldwide. This familial disorder appears to be inherited as a complex genetic trait (13). It is characterized by a combination of hyperandrogenism (either clinical or biochemical), chronic anovulation, and polycystic ovaries. It is frequently associated with insulin resistance and obesity (14). PCOS receives considerable attention because of its high prevalence and possible reproductive, metabolic, and cardiovascular consequences. It is the most common cause of hyperandrogenism, hirsutism, and anovulatory infertility in developed countries (15,16). The association of amenorrhea with bilateral polycystic ovaries and obesity was first described in 1935 by Stein and Leventhal (17). Its genetic origins are likely polygenic and/or multifactorial (18).
Diagnostic Criteria
In an international conference on PCOS organized by the National Institutes of Health (NIH) in 1990, diagnostic criteria for PCOS were based on consensus rather than clinical trial evidence. Their diagnostic criteria recommended clinical and/or biochemical evidence of hyperandrogenism, chronic anovulation, and exclusion of other known disorders. These criteria were an important initial step in standardizing diagnosis and led to a number of landmark randomized clinical trials in PCOS (19).
Table 31.4 Revised Diagnostic Criteria of Polycystic Ovary Syndrome
1990 Criteria (both 1 and 2) |
1. Chronic anovulation and |
2. Clinical and/or biochemical signs of hyperandrogenism and exclusion of other etiologies. |
Revised 2003 criteria (2 out of 3) |
1. Oligoovulation or anovulation |
2. Clinical and/or biochemical signs of hyperandrogenism |
3. Polycystic ovaries and exclusion of other etiologies (congenital adrenal hyperplasia, androgen-secreting tumors, Cushing’s syndrome) |
From Rotterdam ESHRE/ASRM-Sponsored PCOS Consensus Workshop Group. Revised 2003 consensus on diagnostic criteria and long-term health risks related to polycystic ovary syndrome. Fertil Steril 2004;81:19–25, with permission. Permission granted Elsevier 10.13.10. |
Since the 1990 NIH-sponsored PCOS conference, evolving perception is that the syndrome may constitute a broader spectrum of signs and symptoms of ovarian dysfunction than those set forth in the original NIH diagnostic criteria. The 2003 Rotterdam Consensus Workshop concluded that PCOS is a syndrome of ovarian dysfunction along with the cardinal features hyperandrogenism and polycystic ovary (PCO) morphology (Table 31.4).
It is recognized that women with regular cycles, hyperandrogenism, and PCO morphology may be part of the syndrome. Some women with the syndrome will have PCO morphology without clinical evidence of androgen excess, but will display evidence of ovarian dysfunction with irregular cycles. In this new schema, PCOS remains a diagnosis of exclusion with the need to rule out other disorders that mimic the PCOS phenotype (19).
Using the Rotterdam PCOS Diagnostic Criteria, the presence of two of the three criteria is sufficient to diagnosis PCOS: menstrual cycle anomalies (amenorrhoea, oligomenorrhea), clinical and/or biochemical hyperandrogenism, and/or the ultrasound appearance of polycystic ovaries after all other diagnoses are ruled out. Other pathologies that can result in a POCS phenotype include AOAH, adrenal or ovarian neoplasm, Cushing syndrome, hypo- or hypergonadotropic disorders, hyperprolactinemia, and thyroid disease (Fig. 31.4).
All other frequently encountered manifestations offer less consistent findings and therefore qualify only as minor diagnostic criteria for PCOS. They include elevated LH-to-FSH (follicle-stimulating hormone) ratio, insulin resistance, perimenarchal onset of hirsutism, and obesity.
Clinical hyperandrogenism includes hirsutism, male pattern alopecia, and acne (19). Hirsutism occurs in approximately 70% of patients with PCOS in the United States and in only 10% to 20% of patients with PCOS in Japan (20,21). A likely explanation for this discrepancy is the genetically determined differences in skin 5α-reductase activity (22,23).
Nonclassic adrenal hyperplasia and PCOS may present with similar clinical features. It is important to measure the basal follicular phase 17-hydroxyprogesterone level in all women presenting with hirsutism to exclude the presence of nonclassic congenital adrenal hyperplasia, regardless of the presence of polycystic ovaries or metabolic dysfunction (24).
The menstrual dysfunction in PCOS arises from anovulation or oligo-ovulation and ranges from amenorrhea to oligomenorrhea. Regular menses in the presence of anovulation in PCOS is uncommon, although one report found that among hyperandrogenic women with regular menstrual cycles, the rate of anovulation is 21% (25). Classically, the disorder is lifelong, characterized by abnormal menses from puberty with acne and hirsutism arising in the teens. It may arise in adulthood, concomitant with the emergence of obesity, presumably because this is accompanied by increasing hyperinsulinemia (26).
Figure 31.4 Diagnostic algorithm for polycystic ovary syndrome. (From Rosenfield RL. Clinical practice. Hirsutism. N Engl J Med 2005;353:2578–2588, with permission.)
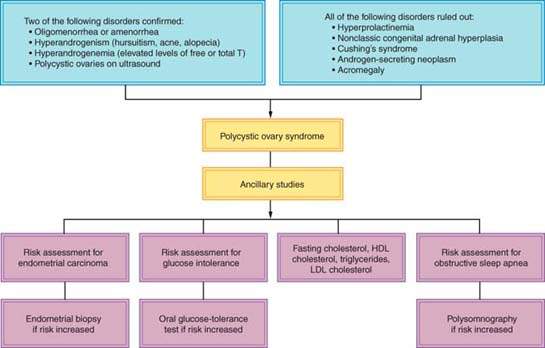
The sonographic criteria for PCO requires the presence of 12 or more follicles in either ovary measuring 2 to 9 mm in diameter and/or increased ovarian volume (>10 mL). A single ovary meeting these criteria is sufficient to affix the PCO diagnosis (19). The appearance of PCO on ultrasound scanning is common. Only a fraction of those with PCO appearance have the clinical and/or endocrine features of PCOS. A PCO appearance is found in about 23% of women of reproductive age, while estimates of the incidence of PCOS vary between 5% and 10% (27). Polycystic appearing ovaries in women with PCOS was not associated with increased cardiovascular disease risk, independent of body mass index (BMI), age, and insulin levels (28). An English study demonstrated that without symptoms of polycystic ovary syndrome, a PCO appearance alone is not associated with impaired fecundity or fertility (29).
Obesity occurs in more than 50% of patients with PCOS. The body fat is usually deposited centrally (android obesity), and a higher waist-to-hip ratio is associated with insulin resistance indicating an increased risk of diabetes mellitus and cardiovascular disease (30). Among women with PCOS, there is widespread variability in the degree of adiposity by geographic location and ethnicity. In studies in Spain, China, Italy, and the United States, the percentage of obese women with PCOS were 20%, 43%, 38%, and 69%, respectively (31).
Insulin resistance resulting in hyperinsulinemia is commonly exhibited in PCOS. Insulin resistance may eventually lead to the development of hyperglycemia and type 2 diabetes mellitus (32). About one-third of obese PCOS patients have impaired glucose tolerance (IGT), and 7.5% to 10% have type 2 diabetes mellitus (33). These rates are mildly increased in nonobese women who have PCOS (10% IGT; 1.5% diabetes, respectively), compared with the general population of the United States (7.8% IGT; 1% diabetes, respectively) (34,35).
Abnormal lipoproteins are common in PCOS and include elevated total cholesterol, triglycerides, and low-density lipoproteins (LDL); and,low levels of high-density lipoproteins (HDL), and apoprotein A-I (30,36). According to one report, the most characteristic lipid alteration is decreased levels of HDL2α (37).
Other observations in women with PCOS include impaired fibrinolysis, as shown by elevated circulating levels of plasminogen activator inhibitor, an increased incidence of hypertension over the years (which reaches 40% by perimenopause), a greater prevalence of atherosclerosis and cardiovascular disease, and an estimated sevenfold increased risk for myocardial infarction (36,38–41).
Pathology
Macroscopically, ovaries in women with PCOS are two to five times the normal size. A cross-section of the surface of the ovary discloses a white, thickened cortex with multiple cysts that are typically less than a centimeter in diameter. Microscopically, the superficial cortex is fibrotic and hypocellular and may contain prominent blood vessels. In addition to smaller atretic follicles, there is an increase in the number of follicles with luteinized theca interna. The stroma may contain luteinized stromal cells (42).
Pathophysiology and Laboratory Findings
The hyperandrogenism and anovulation that accompany PCOS may be caused by abnormalities in four endocrinologically active compartments: (i) the ovaries, (ii) the adrenal glands, (iii) the periphery (fat), and (iv) the hypothalamus–pituitary compartment (Fig. 31.5).
Figure 31.5 Pathophysiological characteristics of the polycystic ovary syndrome (PCOS). Insulin resistance results in a compensatory hyperinsulinemia, which stimulates ovarian androgen production in an ovary genetically predisposed to PCOS. Arrest of follicular development (red “X”) and anovulation could be caused by the abnormal secretion of gonadotropins such as follicle-stimulating hormone (FSH) or luteinizing hormone (LH) (perhaps induced by hyperinsulinemia), intraovarian androgen excess, direct effects of insulin, or a combination of these factors. Insulin resistance, in concert with genetic factors, may also lead to hyperglycemia and an adverse profile of cardiovascular risk factors. (From Rosenfield RL. Clinical practice. Hirsutism. N Engl J Med 2005;353:2578–2588, with permission.)
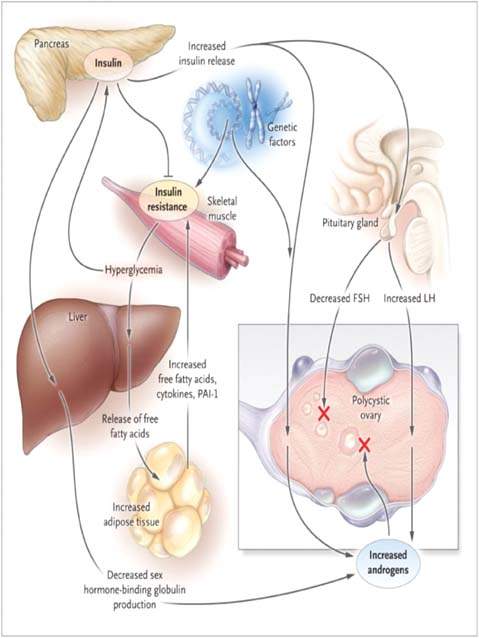
In patients with PCOS, the ovarian compartment is the most consistent contributor of androgens. Dysregulation of CYP17, the androgen-forming enzyme in both the adrenals and the ovaries, may be one of the central pathogenetic mechanisms underlying hyperandrogenism in PCOS (43). The ovarian stroma, theca, and granulosa contribute to ovarian hyperandrogenism and are stimulated by LH (44). This hormone relates to ovarian androgenic activity in PCOS in a number of ways.
The increased testosterone levels in patients with PCOS are considered ovarian in origin. The serum total testosterone levels are usually no more than twice the upper normal range (20 to 80 ng/dL). However, in ovarian hyperthecosis, values may reach 200 ng/dL or more (48). The adrenal compartment plays a role in the development of PCOS. Although the hyperfunctioning CYP17 androgen-forming enzyme coexists in both the ovaries and the adrenal glands, DHEAS is increased in only about 50% of patients with PCOS (49,50). The hyperresponsiveness of DHEAS to stimulation with ACTH, the onset of symptoms around puberty, and the observation of 17,20-lyase activation (one of the two CYP17 enzymes) are key events in adrenarche that led to the hypothesis that PCOS arises as an exaggeration of adrenarche (48).
The peripheral compartment, defined as the skin and the adipose tissue, manifests its contribution to the development of PCOS in several ways.
The hypothalamic–pituitary compartment participates in aspects critical to the development of PCOS.
Polycystic ovary syndrome is a complex multigenetic disorder that results from the interaction between multiple genetic and environmental factors. Genetic studies of PCOS reported allele sharing in large PCOS patient populations and linkage studies focused on candidate genes most likely to be involved in the pathogenesis of PCOS. These genes can be grouped in four categories: (i) insulin resistance–related genes, (ii) genes that interfere with the biosynthesis and the action of androgens, (iii) genes that encode inflammatory cytokines, and (iv) other candidate genes (57).
Linkage studies identified the follistatin, CYP11A, Calpain 10, IRS-1 and IRS-2 regions and loci near the insulin receptor (19p13.3), SHBG, TCF7L2, and the insulin genes, as likely PCOS candidate genes (58–64). A polymorphic variant, D19S884, in FBN3 was found to be associated with risk of PCOS (65). Using theca cells derived from women with PCOS elevated mRNA levels was noted for CYP11A, 3BHSD2, and CYP17 genes with corresponding overproduction of testosterone, 17-α-hydroxyprogesterone, and progesterone. Despite the characteristically heightened steroidogenesis in POCS, the STARB gene was not overexpressed (58). Microarray data using theca cells from PCOS women did not identify any genes near the 19p13.3 locus that were differentially expressed; however, the mRNAs of several genes that map to 19p13.3, including the insulin receptor, p114-Rho-GEF, and several expressed sequence tags, were detected in both PCOS and normal theca cells. Those studies identified new factors that might impact theca cell steroidogenesis and function, including cAMP-GEFII, genes involved in all-transretinoic acid (atRA) synthesis signaling, genes that participate in the Wnt signal transduction pathway, and transcription factor GATA6. These findings suggest that a 19p13.3 locus or some other candidate gene may be a signal transduction gene that results in overexpression of a suite of genes downstream that may affect steroidogenic activity (66). Polymorphisms in major folliculogenesis genes, GDF9, BMP15, AMH, and AMHR2, are not associated with PCOS susceptibility (67).
Insulin Resistance
Patients with PCOS frequently exhibit insulin resistance and hyperinsulinemia. Insulin resistance and hyperinsulinemia participate in the ovarian steroidogenic dysfunction of PCOS. Insulin alters ovarian steroidogenesis independent of gonadotropin secretion in PCOS. Insulin and insulin-like growth factor I (IGF-I) receptors are present in the ovarian stromal cells. A specific defect in the early steps of insulin receptor–mediated signaling (diminished autophosphorylation) was identified in 50% of women with PCOS (68).
Insulin has direct and indirect roles in the pathogenesis of hyperandrogenism in PCOS. Insulin in collaboration with LH enhances the androgen production of theca cells. Insulin inhibits the hepatic synthesis of sex hormone–binding globulin, the main circulating protein that binds to testosterone, thus increasing the proportion of unbound or bioavailable testosterone (13).
The most common cause of insulin resistance and compensatory hyperinsulinemia is obesity, but despite its frequent occurrence in PCOS, obesity alone does not explain this important association (56). The insulin resistance associated with PCOS is not solely the result of hyperandrogenism based on the following:
Acanthosis nigricans is a reliable marker of insulin resistance in hirsute women. This thickened, pigmented, velvety skin lesion is most often found in the vulva and may be present on the axilla, over the nape of the neck, below the breast, and on the inner thigh (72). The HAIR-AN syndrome consists of hyperandrogenism (HA), insulin resistance (IR), and acanthosis nigricans (AN) (68,73). These patients often have high testosterone levels (>150 ng/dL), fasting insulin levels of greater than 25 μIU/mL (normal <20 to 24 μIU/mL), and maximal serum insulin responses to glucose load (75 gm) exceeding 300 μIU/mL (normal is <160 μIU/m: at 2 hours postglucose load).
Screening Strategies for Diabetes and Insulin Resistance
The 2003 Rotterdam Consensus Group recommends that obese women with PCOS and nonobese PCOS patients with risk factors for insulin resistance, such as a family history of diabetes, should be screened for metabolic syndrome, including glucose intolerance with an oral glucose tolerance test (19). The standard 2-hour oral glucose tolerance test (OGTT) provides an assessment of both the degrees of hyperinsulinemia and glucose tolerance and yields the highest amount of information for a reasonable cost and risk (7).
Multiple other testing or screening schema were proposed to assess the presence of hyperinsulinemia and insulin resistance. In one, the fasting glucose-to-insulin ratio is determined, and values less than 4.5 indicate insulin resistance. Using the 2-hour GTT with insulin levels, 10% of nonobese and 40% to 50% of obese PCOS women have impaired glucose tolerance (IGT = 2-hour glucose level ≥140 but ≤199 mg/dL) or overt type 2 diabetes mellitus (any glucose level >200 mg/dL). Some research studies utilized a peak insulin level of over 150 μIU/mL or a mean level of over 84 μIU/mL over the three blood draws of a 2-hour GTT as a criteria to diagnoses hyperinsulinemia.
The documentation of hyperinsulinemia using either the glucose to insulin ratio or the 2-hour GTT with insulin is problematic. When compared to the gold standard measure for insulin resistance, the hyperinsulemic-euglycemic clamp, it shows that the glucose-to-insulin ratio does not always accurately portray insulin resistance. When hyperglycemia is present, a relative insulin secretion deficit is present. This deficient insulin secretion exacerbates the effects of insulin resistance and renders inaccurate the use of hyperinsulinemia as an index of insulin resistance. Thus, routine measurements of insulin levels may not be particularly useful.
Although detection of insulin resistance, per se, is not of practical importance to the diagnosis or management of PCOS, testing women with PCOS for glucose intolerance is of value because their risk of cardiovascular disease correlates with this finding. An appropriate frequency for such screening depends on age, BMI and waist circumference, all of which increase risk.
Interventions
Two-Hour Glucose Tolerance Test Normal Glucose Ranges (World Health Organization criteria, after 75-gm glucose load)
Two-Hour Glucose Values for Impaired Glucose Tolerance and Type 2 Diabetes (World Health Organization criteria, after 75-gm glucose load)
Abnormal glucose metabolism may be significantly improved with weight reduction, which may reduce hyperandrogenism and restore ovulatory function (74). In obese, insulin-resistant women, caloric restriction that results in weight reduction will reduce the severity of insulin resistance (a 40% decrease in insulin level with a 10-kg weight loss) (75). This decrease in insulin levels should result in a marked decrease in androgen production (a 35% decrease in testosterone levels with a 10-kg weight loss) (76). Exercise reduces insulin resistance, independent from any associated weight loss, but data on the impact of exercise on the principal manifestations of PCOS are lacking.
In addition to addressing the increased risk for diabetes, the clinician should recognize insulin resistance or hyperinsulinemia as a cluster syndrome called metabolic syndrome or dysmetabolic syndrome X. Recognition of the importance of insulin resistance or hyperinsulinemia as a risk factor for cardiovascular disease led to diagnostic criteria for the dysmetabolic syndrome. The more dysmetabolic syndrome X criteria are present, the higher the level of insulin resistance and its downstream consequences. The presence of three of the following five criteria confirm the diagnosis, and an insulin-lowering agent and/or other interventions may be warranted (19).
Metabolic Syndrome Diagnostic Criteria
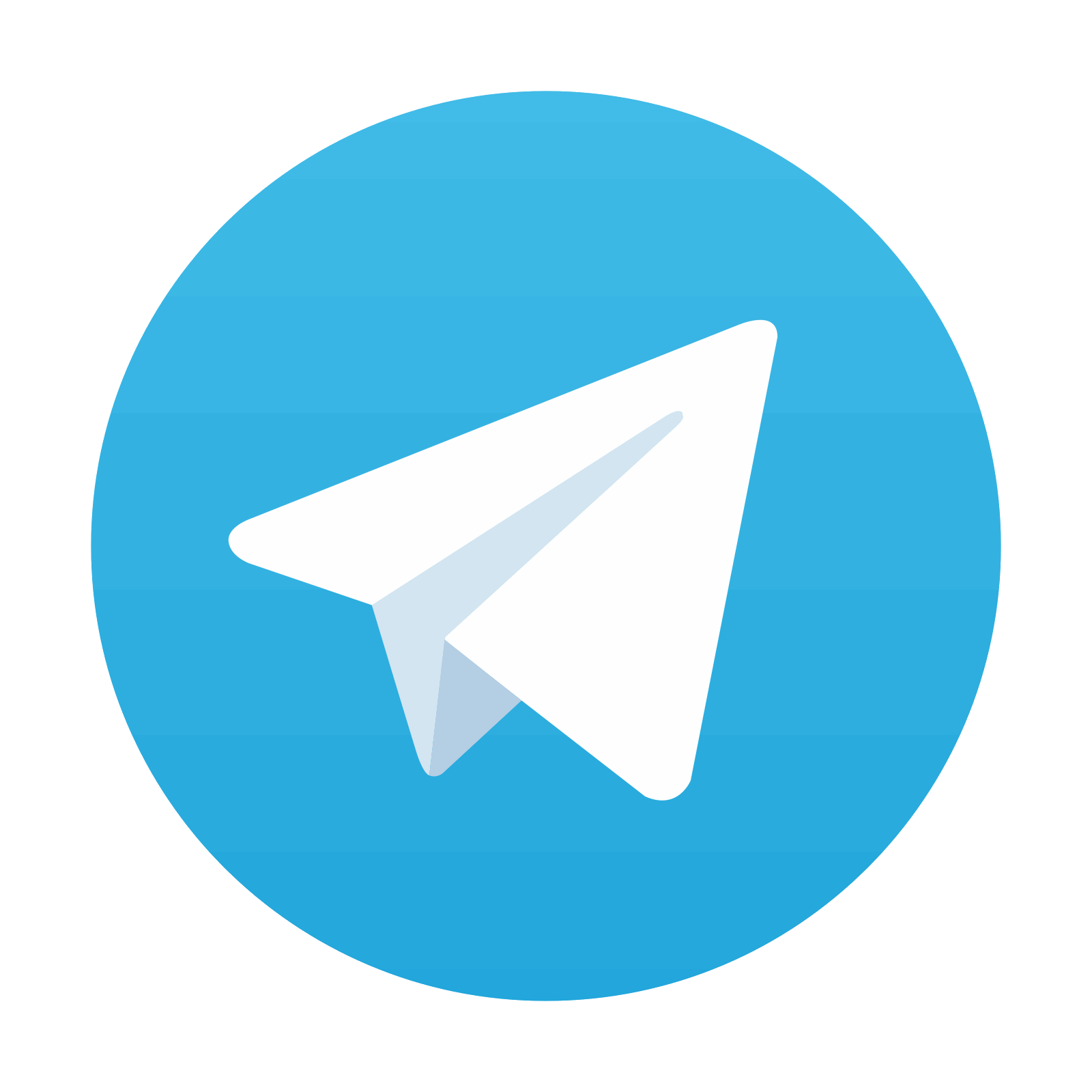
Stay updated, free articles. Join our Telegram channel

Full access? Get Clinical Tree
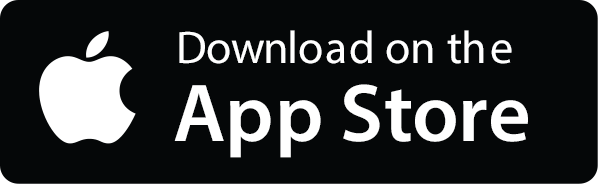
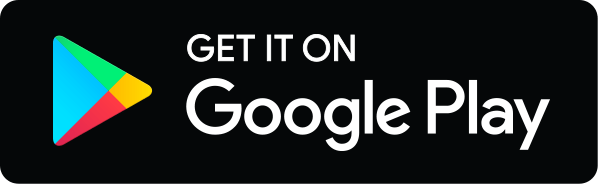