CHAPTER 21 Disorders of male reproduction
Introduction
There has been rapid progress during the past 20 years in our understanding of male reproductive physiology, with wide-ranging contributions from cell and molecular biologists, urologists and endocrinologists, whose efforts have contributed to establishing the discipline of andrology. However, this specialty is not established in the UK, and men with reproductive disorders often present to the gynaecologist through the intermediary of their female partners. It is therefore important for gynaecologists to have some knowledge of male reproductive function and its disorders in order to recognize potential problems, as well as an understanding of the available diagnostic techniques and treatment options.
This chapter aims to provide the practising gynaecologist with an overview of clinical andrology with an emphasis on male infertility. A description of normal physiology is given as the foundation for explaining pathophysiological mechanisms and as a basis for formulating rational treatment where possible.
Physiology
The testis can be thought of as having two major interconnected functions in the adult: the production of testosterone, which maintains a wide range of physiological processes; and the production of spermatozoa and thereby fertility.
Spermatogenesis
Spermatogenesis takes place in several hundred tightly coiled seminiferous tubules arranged in lobules (Figure 21.1) (Dym 1977), which constitute some 80% of testicular volume in man. Testis volume therefore reflects spermatogenesis more than testosterone production. Each tubule resembles a loop draining at both ends into a network of tubules, the rete testis, and thence into the epididymis, a single but highly coiled tube which, in turn, drains into the unconvoluted and muscular-walled vas deferens.
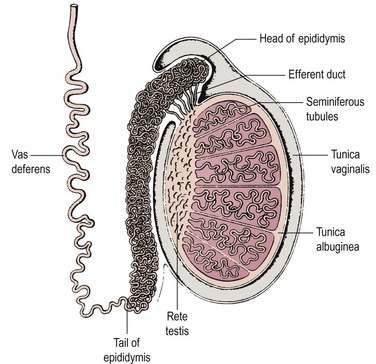
Figure 21.1 Human testis, epididymis and vas deferens showing efferent ducts leading from the rete testis to the caput epididymis and the cauda epididymis, continuing to become the vas deferens.
From Dym M (1977). In Histology pp. 981 eds L Weiss and RO Greep, with permission of Elsevier.
The walls of the seminiferous tubules are composed of germ cells and Sertoli cells around a central lumen, surrounded by peritubular myoid cells and a basement membrane (Figure 21.2). Spermatogenesis is a continuous sequence of closely regulated events, highly organized in space and time, whereby cohorts of undifferentiated diploid germ cells (spermatogonia) multiply and, while maintaining the population of stem cell spermatogonia, are then transformed into haploid spermatozoa. The following events can be observed in the seminiferous epithelium during normal spermatogenesis.
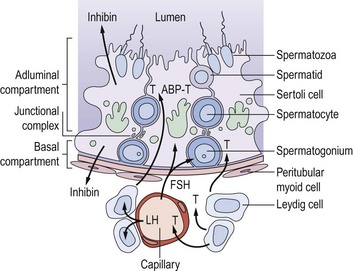
Figure 21.2 Diagrammatic representation of the anatomical and functional relationship between germ cells, Sertoli cells and Leydig cells. Note the division of the seminiferous epithelium into adluminal and basal compartments by the tight junctions between adjacent Sertoli cells and the bidirectional secretion of Sertoli cell products (e.g. inhibin) into the lumen and interstitial space. ABP-T, androgen-binding protein testosterone; FSH, follicle-stimulating hormone; LH, luteinizing hormone; T, testosterone.
Cohorts of undifferentiated germ cells, joined to each other by cytoplasmic bridges, progress through these different steps in synchrony so that several generations of developing germ cells are usually observed at any one part of the seminiferous epithelium at any one time. The total time taken for a cohort of spermatogonia to develop into spermatozoa is 74 days, during which time at least three further generations of spermatogonia have also successively, at intervals of 16 days, initiated their development. In the human, spermatogenesis is arranged in a helical manner, so that cross-sections show more than one stage of spermatogenesis within individual seminiferous tubules. This organizational arrangement differs from that in many other species (e.g. rodents), in which spermatogenesis is arranged longitudinally and thus only one stage of spermatogenesis is seen in a cross-section of a tubule.
Sertoli cell function
Sertoli cells have extensive cytoplasm which spans the full height of the seminiferous epithelium from basement membrane to lumen (Figure 21.2). Where adjacent Sertoli cells come into contact with each other near the basement membrane, special occluding junctions are formed which divide the seminiferous epithelium into a basal (outer) compartment, which interacts with the systemic circulation, and an adluminal (inner) compartment enclosed by a functional permeability barrier, the blood–testis barrier (Figure 21.2). Spermatogonia divide by mitosis in the basal compartment, while the two reduction divisions of the spermatocytes and spermiogenesis are confined to the avascular microenvironment of the adluminal compartment created by the blood–testis barrier. The developing germ cells are therefore completely dependent on Sertoli cells for metabolic support. In response to appropriate trophic stimuli [of which follicle-stimulating hormone (FSH) and testosterone are the best described], Sertoli cells secrete a wide range of substances including growth factors and a distinctive tubular fluid high in potassium and low in protein which bathes the mature spermatozoa.
Sertoli cells contribute directly to the feedback regulation of pituitary gonadotrophin secretion. The existence of an endocrine product of the testis termed ‘inhibin’ was postulated for many decades. Inhibin B is a dimeric glycoprotein secreted by Sertoli cells which has a physiological role in the regulation of FSH secretion. Inhibin B concentrations reflect the functional activity of the seminiferous tubule and show a positive relationship with sperm production. Its production requires and reflects the interaction of the germ cell population with the Sertoli cells, and is absent in men with Sertoli cell only syndrome. The measurement of inhibin B may be of clinical value as a marker of the activity of the seminiferous epithelium (O’Connor and De Kretser 2004). Inhibin A, the product of the dominant follicle and the corpus luteum in women, is not present in the circulation in men.
Unlike the actively dividing germ cells, Sertoli cells do not proliferate in the adult testis. Spermatogenesis is a cyclical process which is critically dependent on changes in Sertoli cell function associated with the constantly changing combination of germ cells in contact with its cytoplasm. Changes in the germ cell complement in contact with any one Sertoli cell occur at a fixed sequence and interval. Thus, the synchronization of these repetitive cyclical changes in Sertoli cell function, associated with the variations in germ cell metabolic requirements as they divide and differentiate, has now become one of the central tenets of our conceptualization of normal spermatogenesis (Sharpe 1990). Although pituitary gonadotrophins provide obligatory trophic support for testicular function as a whole, the classic concept that luteinizing hormone (LH) stimulates Leydig cell steroidogenesis and FSH controls functions in the seminiferous tubules is far too simplistic in the light of our current understanding of spermatogenesis. There is now good evidence that the interstitial and tubular compartments are not functionally distinct, but that there is a close and complex inter-relationship between them. Thus, testosterone from the interstitial Leydig cells stimulates Sertoli cell functions either directly or via the peritubular cells, as does Leydig-cell-derived insulin-like factor 3, itself critical for testicular descent (Ivell et al 2005). Altered tubular/Sertoli cell function, on the other hand, can induce changes in Leydig cell steroidogenesis, although the identity of the intercompartmental regulator(s) is unknown.
The Leydig cell
The adult human testis contains some 500 million Leydig cells clustered in the interstitial spaces adjacent to the seminiferous tubules. The biosynthesis of testosterone in Leydig cells is under the control of LH which binds to specific surface membrane receptors. Steroidogenesis is stimulated through a cyclic adenosine monophosphate (cAMP)–protein kinase C mechanism which mobilizes cholesterol substrate and promotes the conversion of cholesterol to pregnenolone by splitting the C21 side chain. The subsequent steps in the biosynthetic pathway involve the weakly androgenic intermediates dehydroepiandrosterone and androstenedione before testosterone, the principal secretory product, is obtained. Testosterone is secreted into the spermatic venous system, testicular lymphatics and tubular fluid.
Testosterone is the most important circulating androgen in the adult male, since most dihydrotestosterone is formed locally in androgen-responsive target tissues. When circulating in plasma, testosterone is bound to sex-hormone-binding globulin (SHBG) and albumin. The latter binds to all steroids with low affinity, while SHBG, a glycoprotein synthesized in the liver, has a high affinity but a low capacity for testosterone. In men, 60% of circulating testosterone is bound to SHBG, 38% is bound to albumin and 2% is free. Free and albumin-bound testosterone constitute the bioavailable fractions of circulating testosterone, but recent evidence suggests that SHBG-bound testosterone may also be extractable in some tissues, although the functional significance of this is unclear. The plasma concentration of SHBG is regulated by factors including steroid hormones, its synthesis being increased by oestrogens and reduced by testosterone. It is also related to body weight, being lower in the obese. These relationships are the same as in women.
Hormonal Control of Spermatogenesis
The hormonal control of spermatogenesis requires the actions of the pituitary gonadotrophins LH and FSH. There is general agreement that both LH and FSH are needed for the initiation of spermatogenesis during puberty. However, the specific roles and relative contributions of the two gonadotrophins in maintaining spermatogenesis are unclear (Liu et al 2002).
FSH initiates function in immature Sertoli cells, prior to the onset of spermatogenesis, by stimulating the formation of the blood–testis barrier and the secretion of tubular fluid and other specific secretory products via FSH receptors which activate intracellular cAMP. Once spermatogenesis is established in the adult testis, Sertoli cells become less responsive to FSH. Evidence for the non-essential role of FSH is provided by individuals with inactivating mutations of the FSH receptor. Such men have been documented to have complete spermatogenesis but with low sperm concentrations (Tapanainen et al 1997). However, it can be shown, in animals immunized against FSH and in experimentally induced hypogonadotrophic men given gonadotrophin replacement, that both testosterone (depending on LH) and FSH are required for quantitatively normal spermatogenesis in the adult (testosterone-replete) testis by determining the number of spermatogonia available by meiosis. However, testosterone on its own can maintain qualitatively normal spermatogenesis once it has been initiated (Matsumoto et al 1984). FSH therefore acts either by increasing spermatogonial mitosis or by decreasing the number of cells that degenerate at each cell division. Testosterone is essential for the subsequent stages from meiosis to spermiogenesis.
Hypothalamic–pituitary–testicular axis
The secretion of gonadotrophins from the anterior pituitary gland is controlled by gonadotrophin-releasing hormone (GnRH) released into the pituitary portal circulation from axon terminals in the hypothalamic median eminence. These neurosecretory neurones in the medial basal hypothalamus are responsive to a wide variety of sensory inputs as well as to gonadal negative feedback. GnRH stimulates the secretion of both LH and FSH. In the adult male, GnRH is released episodically into the pituitary portal circulation at a frequency of approximately every 140 min; each volley of GnRH elicits an immediate release of LH, producing the typical pulsatile pattern of LH in the systemic circulation (Figure 21.3; Wu et al 1989). Although also secreted episodically, FSH and testosterone pulses are not apparent in normal men because of the slower secretion of newly synthesized rather than stored hormone and the longer circulating half-lives. The intermittent mode of GnRH stimulation, within a narrow physiological range of frequency, is obligatory for sustaining the normal pattern of gonadotrophin secretion. Continuous or high-frequency GnRH stimulation paradoxically desensitizes the pituitary gonadotrophin response in men as in women, because of depletion of receptors and refractoriness of postreceptor response mechanisms. It has recently been demonstrated that the secretion of GnRH is dependent on the newly-described kisspeptin (also known as ‘metastin’). Mutations of the kisspeptin receptor result in pubertal failure. Kisspeptin-containing neurones impinge directly on GnRH neurones in the hypothalamus, and there is emerging evidence that they also mediate effects of metabolic signals such as leptin on the reproductive axis (Seminara and Crowley 2008).
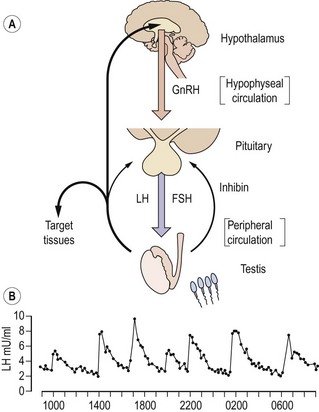
Figure 21.3 (A) Functional relationships in the hypothalamic–pituitary–testicular axis. Gonadotrophin-releasing hormone (GnRH) is secreted into the hypophyseal circulation in an episodic manner, represented by a luteinizing hormone (LH) pulse in the peripheral circulation. (B) Peripheral blood LH concentration sampled in an adult male at 10-min intervals for 24 h from 0900 to 0900 h.
Testosterone exerts the major negative feedback action on gonadotrophin secretion. Its effect is predominantly to restrict the frequency of GnRH pulses from the hypothalamus to within the physiological range. Testosterone also acts on the pituitary to reduce the amplitude of the LH response to GnRH. It is now recognized that these inhibitory effects on GnRH and gonadotrophin secretion are, in part, mediated following conversion of testosterone to oestradiol by the enzyme P450 aromatase. This is demonstrated both by administration of aromatase inhibitors to normal men and by the finding that men with either mutant, non-functional oestrogen receptors or absent aromatase activity have markedly elevated gonadotrophin concentrations despite high-normal testosterone concentrations (Smith et al 1994, Morishima et al 1995). Interestingly, these men also showed marked osteoporosis, suggesting a distinct role for oestrogen in bone. Feedback inhibition of pituitary FSH synthesis is predominantly mediated by inhibin B and also by testosterone, particularly at high concentrations. The regulation of FSH secretion by inhibin in addition to testosterone results in the selective rise in FSH but not LH concentrations in men with various disorders of spermatogenesis.
The spermatozoon
The primary function of the spermatozoon is the delivery of a male pronucleus to the fertilized egg. The spermatozoon must conserve its DNA and transport it to the site of fertilization, where it must recognize and fuse with a receptive egg. The ejaculated spermatozoon must first escape from the seminal plasma in which it is deposited beside the cervix, and penetrate the barrier presented by cervical mucus. It must then travel through the uterus to the site of fertilization in the fallopian tube. During this journey, it must complete the process of functional maturation known as ‘capacitation’, an ordered series of events involving reorganization of cell surface components and changes in cellular metabolism and motility patterns, which are a prerequisite for successful fertilization. Having reached the oviduct, the male gamete must recognize the oocyte, penetrate the cumulus oophorus and bind to the zona pellucida. At this point, it must display a unique pattern of movement known as ‘hyperactivated motility’ and undergo the acrosome reaction. This process is initiated by a specific protein component of the zona pellucida (ZP3) and results in release of the contents of the acrosomal matrix, which include the serine protease acrosin and other hydrolytic enzymes including hyaluronidase. In addition, the acrosome reaction results in the generation of a fusogenic equatorial segment, which is the zone of fusion with the oocyte plasma membrane. For a review of sperm structure and function, the reader is referred to Grudzinskas (1995).
To enable it to undertake these complex functions, the human spermatozoon has developed a highly specialized morphology, with its various structural components tailored to specific functional attributes. The appearance of the spermatozoon was first described over 300 years ago by Anthony van Leeuwenhoek. In outline, the spermatozoon has a dense oval head capped by an acrosome and is propelled by a motile tail (Figure 21.4; Fawcett 1975). The head is made up largely of highly condensed nuclear chromatin constituting the haploid chromosome complement, complexed with highly basic proteins termed ‘protamines’. It is covered in its anterior half by the acrosome, a membrane-enclosed sac of enzymes including acrosin and hyaluronidase. The area of the sperm head immediately behind the acrosome (the equatorial region) is important as it is this part which attaches to and fuses with the egg. The shape of the human sperm head is highly pleomorphic, making the morphological definition of a normal sperm head extremely challenging. Behind the head may be found a cytoplasmic droplet which consists of the remains of the residual cytoplasm left after the morphological remodelling of the cell during spermiogenesis.
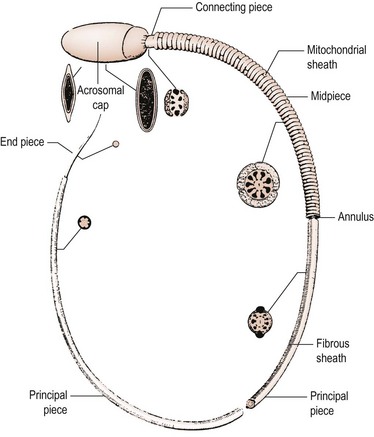
Figure 21.4 The internal structure of a spermatozoon with the cell membrane removed.
From Fawcett DW, The mammalian spermatozoon. Dev Biol; 44: 394–436, 1975; with permission of Elsevier.
The tail of flagellum is usually further divided into the midpiece, principal piece and terminal piece, joined to the head by the connecting piece. The motor apparatus of the tail is the axoneme which consists of a central pair (doublets) of microtubules of non-contractile tubulin protein enclosed in a sheath linked radially to nine outer pairs of microtubules. The axonemal complex is surrounded by columns of outer dense fibres, which are, in turn, covered by a helix of mitochondria in the midpiece and a fibrous sheath in the principal piece. The dense fibres and the fibrous sheath form the cytoskeleton of the flagellum. Through the hydrolysis of adenosine triphosphate, the dynein arms undergo a series of conformational changes resulting in adjacent doublets sliding over one another. Synchronized movement of groups of microtubules propagating waves of bending motions of the tail is the key to the various modes of coordinated sperm motility. Energy for sperm motility is provided by the sheath of mitochondria in the midpiece of the tail through a second messenger system, involving the calcium-mediated calmodulin-dependent conversion of adenosine triphosphate to cAMP and interaction with the adenosine triphosphatase of the dynein.
Sperm transport and maturation
Spermatozoa within the testis and male tract are quiescent and play little active role in their own transport along the tract. Moreover, they are functionally immature and the maturation process continues as they pass through the epididymis. Passage out of the seminiferous tubules and through the main testicular collecting duct, the rete testis, is due to the flow of secretions from Sertoli cells and rete epithelium and to the intrinsic smooth muscle contractions of the tubules. From the rete, the cells pass through the efferent ducts to the epididymis, a 3–4-m-long single coiled tube whose function is under androgen and neural (adrenergic) control. It is typically subdivided into a caput or head region, a middle body or corpus, and a distal tail or cauda region which leads into the proximal vas deferens. The epididymal epithelium actively reabsorbs testicular fluid but also secretes a hyperosmolar fluid rich in glycerophosphorylcholine inositol and carnitine. The specific transport of these compounds across the epithelium creates a favourable fluid environment where progressive motility and fertilizing capacity of the spermatozoa are normally acquired. Some of these maturational changes are probably ‘housekeeping’ functions to ensure that the cell remains viable during its stay in the excurrent ducts, while others are associated with the development of fertilizing ability. In many animal species, spermatozoa retain fertility for several weeks in the cauda epididymis, which acts as a sperm reservoir prior to ejaculation. The human cauda epididymis, in contrast, has a relatively poor storage function, which diminishes further along the vas.
At ejaculation, spermatozoa pass along the vas and are mixed with the secretions of the accessory glands which form over 90% of the volume of the ejaculate. The seminal vesicles contribute the largest volume of alkaline fluid to the ejaculate, and are also the souce of seminal fructose, prostaglandins and coagulating proteins. Prostatic secretions contain proteolytic enzymes (which normally liquefy the coagulated proteins in semen within 20–30 min) and are rich in citric acid and zinc. Seminal plasma provides a support medium for transporting male gametes out of the body and for buffering the acidic pH of the vagina, so that a reservoir of functional sperm can be established after ejaculation. Just as testicular germ cells are subjected to constant attrition, ejaculated spermatozoa have to traverse the cervix, uterus and uterotubal junction before reaching the middle third of the oviduct, the site of fertilization. At each barrier, the sperm population is further reduced so that eventually only 200 or so of the most robust spermatozoa have the opportunity to fertilize the ovum. The number of functionally competent sperm is more important than the total number ejaculated.
The cervical canal is the first selective filtering barrier to meet the ejaculated sperm (Figure 21.5). This barrier is virtually complete except during midcycle, when oestrogenized cervical mucus glycoprotein fibrils form parallel chains called ‘micelles’ which permit spermatozoa with active progressive motility to swim through at a rate of 2–3 mm/min. Spermatozoa probably enter the uterine cavity from the internal os by virtue of their own motility, and appear in the uterine cavity approximately 90 min after insemination. The uterotubal junction is the second of the major physical barriers for spermatozoa. The mechanism for selectivity is not clear, but may depend on factors other than sperm motility since inert particles can pass through. Once the uterotubal junction has been successfully negotiated, a minority of sperm immediately traverse the oviduct to the ampulla; however, the majority congregate in the isthmus until ovulation has occurred. At this time, capacitated sperm showing hyperactivated movements of the tail gradually progress towards the fimbriated end, helped on by the muscular contraction of the oviduct wall and the flow of fluid in the oviduct. A maximum number of spermatozoa is present in the cervix 15–20 min following insemination and remains constant for 24 h, although a rapid decline has commenced by 48 h. Some spermatozoa may remain motile at the site of fertilization for up to 3 days (Mortimer 1995).
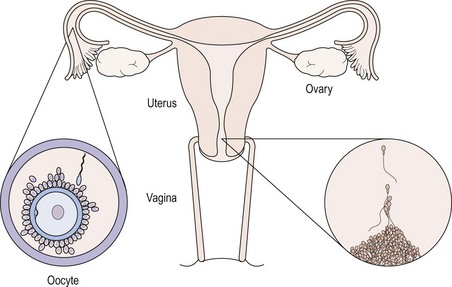
Figure 21.5 Attributes of sperm function associated with fertility in vivo. The spermatozoon must escape from seminal plasma, penetrate and traverse the cervical mucus barrier, reach the site of fertilization, undergo capacitation, bind to and penetrate the zona pellucida, fuse with the plasma membrane of the egg, and undergo nuclear decondensation within the cytoplasm of the oocyte.
Male reproductive ageing
Reproductive ageing in the male is not accompanied by such an overt and abrupt fall in gonadal function as occurs in women, but by a more gradual decline. These differences reflect the consequence of a finite pool of gametes compared with one that continually replicates from a stock of stem cells, and the clearer distinction between the endocrine and gametogenic functions of the gonads.
The decline in function of the endocrine output of the hypothalamo–pituitary–testicular axis with age is well established and results in a fall of approximately 50% in plasma testosterone concentrations (Vermeulen 1991). This has both central and peripheral components; thus, there is a relatively small increase in LH. The ‘andropause’ is increasingly the subject of investigation (Wu 2007), with large placebo-controlled studies of the effect of replacement under way. Changes in Sertoli cell function and spermatogenesis with ageing have received more limited investigation, but the data do suggest that there is indeed an age-related decline in function. Spermatogenesis, however, may be well maintained in elderly men.
Male Infertility
Definition and epidemiology
Infertility is commonly defined as the failure of conception after at least 12 months of unprotected intercourse (Rowe 1993), but such a definition serves to obscure the true complexity of the clinical situation. In reality, those couples who fail to achieve a pregnancy within 12–24 months include those who can be considered sterile (i.e. who will never achieve a spontaneous pregnancy) and those who are more properly termed ‘subfertile’ and who have reduced fecundability (probability of achieving a pregnancy within one menstrual cycle) and hence a prolonged time to pregnancy. Accurate assessment of the prevalence of infertility has always been difficult because of the scarcity of large-scale, population-based studies. Estimates suggest that some 14–17% of couples may be affected at some time in their reproductive lives (Hull et al 1985), with European data suggesting that as many as one in four couples who try may experience difficulties in conceiving (Schmidt 2006).
While infertility is relatively common, it is very difficult indeed to establish the relative contribution of the male partner, given the profound difficulties which exist in the accurate diagnosis of male infertility. Most studies which have attempted to evaluate the aetiology of infertility have used the conventional criteria of semen quality, promulgated by the World Health Organization (WHO) (World Health Organization 1999), to define the ‘male factor’. Although of great importance and shortly to be updated, these criteria are of limited diagnostic value, and a significant proportion of men with normal conventional criteria of semen quality will be infertile because of defects in sperm function, while a significant number of men with abnormal semen quality will have normal sperm function. Very few studies on the epidemiology of male infertility have used functional, as opposed to descriptive, diagnostic criteria. Nevertheless, one common theme to emerge is that, using the available diagnostic techniques, male factor infertility is, in many studies, the most common single diagnostic category.
Pathophysiology
In the simplest terms, male infertility is a failure to fertilize the normal ovum arising from a deficiency of functionally competent sperm at the site of fertilization. Since less than 0.1% of ejaculated sperm actually reach the fallopian tube, it is defective sperm function rather than inadequate numbers of sperm ejaculated that constitutes the most important pathophysiological mechanism in male infertility. Specific lesions leading to defective sperm motility or transport and abnormal sperm–egg interaction are probably the key factors responsible for loss of fertilizing capacity in the gametes. In most instances, however, inadequate sperm function is usually but not invariably accompanied by reduced sperm production, suggesting that specific defects in spermatozoa commonly arise from disturbances in regulatory mechanisms which interfere with both germ cell multiplication and maturation in the seminiferous tubules. There is rarely any clinical evidence of systemic endocrine deficiency in men with male infertility. By inference, therefore, disturbances in paracrine regulation within the testis could lead to low sperm output (oligozoospermia), from an increased rate of degeneration in the differentiating spermatogonia at successive mitotic divisions, as well as abnormal spermiogenesis giving rise to spermatozoa with poor motility (asthenozoospermia) and/or abnormal morphology (teratozoospermia). Abnormal epididymal function may lead to defective sperm maturation, impairment of sperm transport or even cell death. Interruption of the transport of normal sperm may be due to mechanical barriers between the epididymis and fallopian tube or abnormal coitus and/or ejaculation.
Aetiology
Notwithstanding the difficulties in diagnosis outlined above, WHO has proposed a scheme for the diagnostic classification of the male partner of the infertile couple (Rowe 1993) (Box 21.1). This approach is of enormous value as a basis for standardization and for comparative multicentre studies. However, many of the male diagnostic categories are of a descriptive nature (e.g. idiopathic oligozoospermia) or of controversial clinical relevance (e.g. male accessory gland infection). Moreover, recent advances in our understanding of the causes of male infertility, particularly in the area of genetic problems (Hargreave 2000), mean that this classification is in need of review. The relative frequency of the major diagnostic categories is shown in Figure 21.6, using data taken from a WHO study of over 8500 couples from 33 centres in 25 countries (Comhaire et al 1987). It can be seen that the largest single male ‘diagnostic’ category was men with seminal abnormalities of unknown cause. Beyond this, varicocoele was a relatively common pathology, as was male accessory gland infection; however, systemic, iatrogenic and endocrine causes were very infrequent.
Box 21.1
Diagnostic categories for the male partner of an infertile couple according to WHO
Source: Rowe PG 1993 WHO Manual for the Standardized Investigation and Diagnosis of the Infertile Couple. Cambridge University Press, Cambridge, UK.
Genetic causes
Perhaps the most striking advances in our understanding of the aetiology of male infertility in the past decade have been in the area of genetics. Many of the ‘systemic’ disorders commonly associated with male infertility (see below) are now understood to have a genetic basis, and as our knowledge of the aetiology of disease expands, this will be increasingly the case. Traditionally, genetic causes of male infertility have been sought at the level of chromosomal abnormalities, with chromosomal abnormalities being detected in between 2.1% and 8.9% of men attending infertility clinics (Chandley 1994). The frequency of chromosomal abnormalities increased as sperm concentration declined, with abnormal karyotypes being found in 15% of azoospermic patients, 90% of whom had Klinefelter’s syndrome (47XXY), which accounted for half of the entire chromosomally abnormal group. In oligozoospermic patients, the incidence of chromosome abnormalities was 4%. However, it has been recognized for some time that structural anomalies of the Y chromosome, resulting in deletion of the distal fluorescent heterochromatin in the long arm, are associated with severe abnormalities of spermatogenesis. More recent studies have defined a family of genes on the Y chromosome involved in spermatogenesis, and it has become clear that a little over 10% of cases of non-obstructive azoospermia may have deletions affecting these genes. A proportion of cases of very severe oligozoospermia may have a similar aetiology. Microdeletions have been found in three non-overlapping regions of the Y chromosome, AZF a-b-c. Several genes have been described and these include RBM, DAZ, DFFRY, DBY and CDY. The abnormality most commonly reported in the literature is a microdeletion in the AZFc region and encompassing the DAZ gene. However, there is no exact correlation between DAZ deletion and the presence or absence of spermatogenesis, but this may be because of an autosomal copy of the DAZ gene (Hargreave 2000). DNA fragmentation has also been identified as the cause of defective sperm function (Tarozzi et al 2007). The ability of microassisted fertilization to overcome severe deficits in spermatogenesis has reinforced the importance of understanding and investigating genetic causes of male subfertility, as these will now be transmitted to the next generation of males.
Cryptorchidism
Undescended testis is a good example of a condition present at birth, and presumed to have its origins in intrauterine life, which is significantly associated with an increased risk of impaired spermatogenesis in later life and with an increased risk of testicular cancer (Irvine 1997). The testis which is not in a low scrotal position by the age of 2 years is histologically abnormal; spontaneous descent rarely occurs after 1 year and there is little evidence that surgical orchidopexy for an undescended testis after 2 years of age improves fertility. For these reasons, treatment should ideally be undertaken between 1 and 2 years of age. Evidence suggests that fertility may also be impaired in boys with retractile testis who experience spontaneous descent during puberty. Apart from the association with infertility, cryptorchidism is a well-established risk factor for testicular cancer, the risk of which in a patient with a history of undescended testis, whether successfully treated or not, is four to 10 times higher than in the general population.
Orchitis
Symptomatic orchitis occurs as a complication in 27–30% of males over the age of 10–11 years who suffer from mumps. In 17% of cases, orchitis is bilateral and seminiferous tubular atrophy is a common sequela of mumps orchitis, although recovery of spermatogenesis even after persistent azoospermia for 1 year has been reported (Sandler 1954). The prevalence of infertility after mumps orchitis is unknown, but fertility should only be impaired significantly if the orchitis is bilateral and occurs after puberty.
Varicocoele
The subject of varicocoele has generated controversy amongst the andrological community since the Edinburgh urologist Selby Tulloch first reported the apparently beneficial effects of treatment (Tulloch 1952). The available evidence certainly suggests that varicocoele is a common pathology and that it is more common in men with lower sperm counts. The diagnosis of visible (grade 3) and palpable (grade 2) varicocoeles is not difficult when the patient is examined in a standing position. The detection of subclinical (grade 1) varicocoeles, where spermatic vein reflux can only be detected during the Valsalva manoeuvre, requires more experience and has been aided by the use of Doppler or scrotal thermography. Prevalence figures from 5% to 25% have been reported in surveys of apparently healthy men (Hargreave 1994
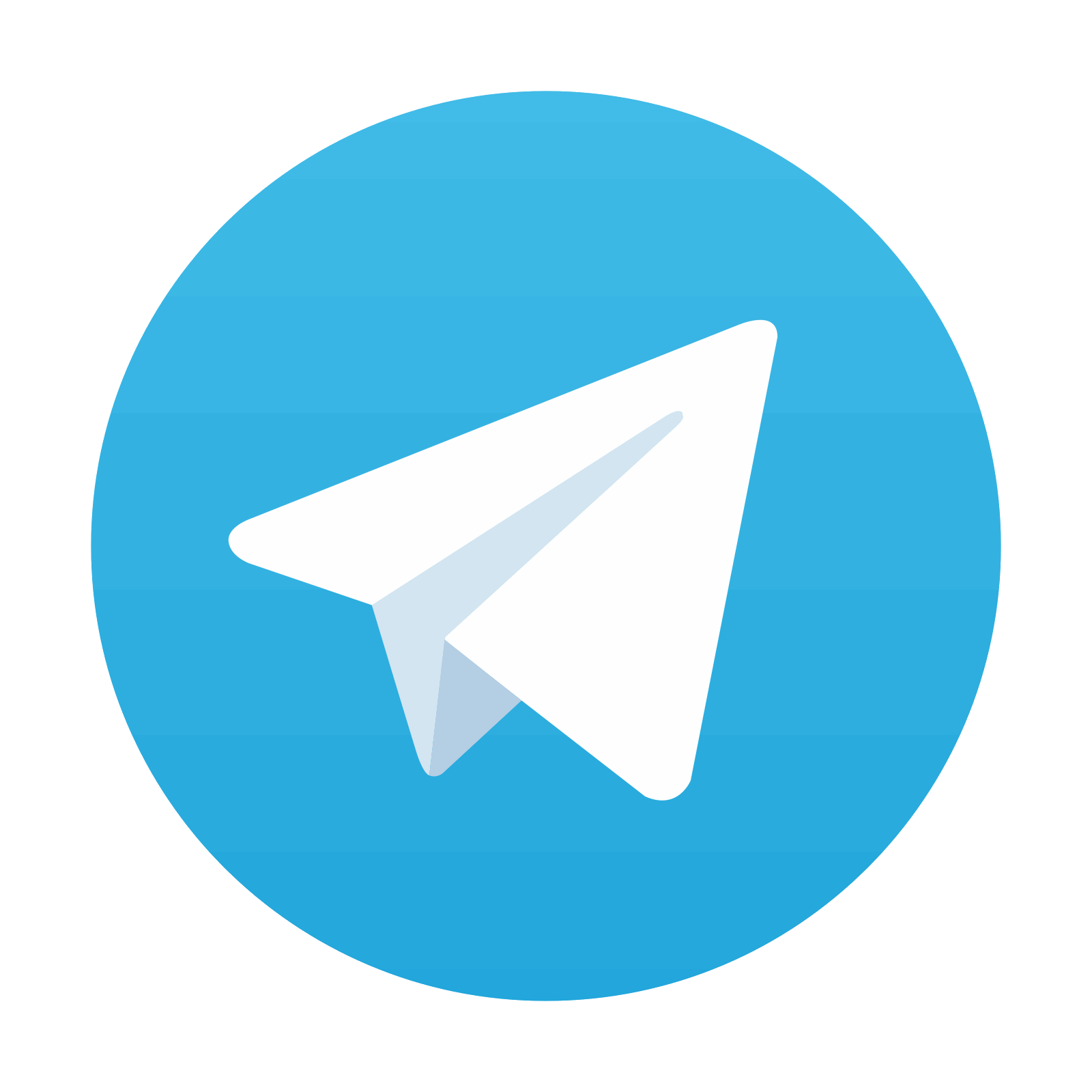
Stay updated, free articles. Join our Telegram channel

Full access? Get Clinical Tree
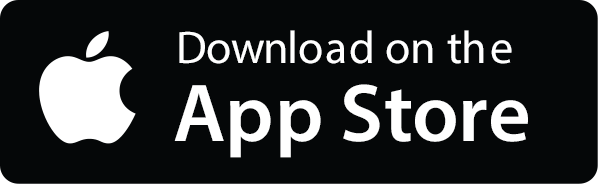
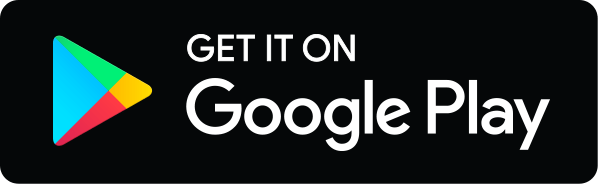