9. Diagnostic Imaging in the Neonate*
John D. Strain and Juley C. Jenkins
Imaging has become an important part of the diagnosis and workup of medical problems of newborns. The ability to use a noninvasive means to diagnose disease, screen for potential pathologic conditions, monitor the effects of therapy, and assist in defining prognosis for counseling has made imaging an essential part of health care. With refinements in diagnostic equipment and capabilities, the role of imaging has expanded significantly in recent years. There are many ways to assess any problem, and the vast potential of the new imaging modalities makes appropriate imaging a constant challenge (Table 9-1). New modalities have been introduced, and advancement in computer technology has added sophistication to established modalities. Nearly 60% of diagnostic imaging involves modalities that were not even available 20 years previously.
Ionizing Radiation | Spatial Resolution | Contrast Resolution | Cost | Sedation | Miscellaneous | |
---|---|---|---|---|---|---|
X-ray | Very low | Excellent | Fair | Low | Never | Very fast acquisition eliminates motion |
Fluoroscopy | Low | Excellent | Fair | Moderate | Never | Evaluates motion real-time |
Ultrasonography | None | Good | Fair | Moderate | Never | Portable; evaluates motion real-time |
Computed tomography | Low | Good | Good | Moderate to high | Sometimes | Cross-sectional imaging |
Magnetic resonance imaging | None | Good | Excellent | High | Frequent | Multiplanar (i.e., in multiple planes) imaging, flowing blood without contrast |
Nuclear medicine | Very low | Poor | Excellent | Moderate to high | Sometimes | Physiologic imaging |
There are many excellent reference books and textbooks on neonatal imaging, and specific questions can be addressed most adequately through these resources. This chapter reviews the various imaging modalities available for diagnosis and intervention. A short summary of each imaging modality includes background information, a discussion of image acquisition, and the risks and benefits of each. We have provided a thumbnail description of each modality; however, for clarity, we have taken significant liberty and license in discussing the physics of image acquisition. Each section addresses the most common usage of the modality in neonates, followed by a focused discussion of one or two aspects of image interpretation.
Because there may be more than one appropriate way to evaluate any given problem, it is essential to understand the inherent advantages and limitations of each modality to decide which might be most effective. We have pointed out some of the challenges associated with diagnostic imaging. A focused problem-solving approach with appropriate collaboration and consultation can yield positive results.
RADIOGRAPHY
Background
The 1896 introduction of the roentgenogram was met with great enthusiasm, and x-ray examination quickly became an indispensable diagnostic tool in clinical settings throughout the world. Until 25 years ago, the field of radiology was based almost exclusively on use of the x-ray.
A beam of ionizing radiation from a source (x-ray tube) passes through the patient, and various structures within the body interact to attenuate the x-ray before it is received on the other side. The x-rays pass through the patient and then expose a film, just as light exposes a negative in black-and-white film photography. The film is developed, and the resultant image (radiograph) is a map that corresponds to the transmitted x-ray (that portion of the x-ray not attenuated by absorption or scattered as it passes through the patient). Somewhat analogous to the shadows that result from objects in the sun, the images from x-ray are a shadow of the object being radiographed. (Hence the slang term “shadow doctor” came into use in reference to early radiologists.) Bone attenuates a greater amount of the x-ray (or allows the penetration of fewer x-rays) than lung tissue does; this results in a film on which the rib is white and the lung black. In some ways, this can be compared with the different shadows cast by the trunk of a tree and by its leaves. With radiography, the spatial resolution is exquisite although the contrast resolution is lacking. One can capture 10 to 20 line pairs per millimeter with film radiography, although only five different densities can be distinguished routinely: air, fat, water (which includes all solid viscera—liver, spleen, kidney, pancreas, and heart), bone, and metal.
More recent developments in x-ray technology include computed radiography (CR) and digital radiography (DR). Although the physics of x-ray generation is essentially the same, the receiver has changed. With CR, a phosphorescent plate replaces film and the latent image can be either exposed to film or captured digitally. With DR, the image is directly captured in a digital mode. The introduction of these products was driven by the desire to capture, archive, distribute, and display digital images. Almost all medical imaging is now digital, and a picture archiving and communication system (PACS) has become an essential component of any imaging department.
Clinical Utility in the Neonatal Intensive Care Setting
Radiography is the simplest and most reliable way to define tube and line position. Radio-opaque markers are incorporated into most of these devices. From peripherally inserted central catheters (PICC) to endotracheal, thoracostomy, and feeding tubes, a simple radiograph can help eliminate the complications of suboptimal line or tube placement (Table 9-2). Chest radiographs are most commonly used to evaluate the heart and lungs. Abdominal imaging allows one to assess the solid viscera (the liver, spleen, and kidneys), as well as the bowel gas pattern, useful in evaluating a neonate with a feeding intolerance (Figure 9-1). Bones of the trunk and extremities are assessed easily with plain film radiology.
PICC, Peripherally inserted central catheter; T8 and T10, thoracic vertebrae 8 and 10. | |
Line/Tube | Position |
---|---|
Endotracheal tube | 1 cm above the level of the carina |
Umbilical artery catheter | Descending aorta between T8 and T10 |
Umbilical venous catheter | Junction inferior vena cava and right atrium |
Central line | Junction superior vena cava and right atrium |
PICC line | Junction superior vena cava and right atrium |
Nasogastric tube | Antrum of the stomach |
In addition to helping determine a specific diagnosis, imaging is frequently a valuable means for assessing patient response to therapy. For instance, lung compliance and volume, as assessed by x-ray studies, help determine the patient’s response to various ventilator rates and pressures; therefore the x-ray findings can be very useful in the selection of the most appropriate ventilator settings.
Focused Discussion: Chest Radiographs
The most common use of x-ray imaging in the neonatal unit is for evaluation of the chest to help define abnormalities that might contribute to respiratory distress. Respiratory distress in newborns can be divided into three categories: conditions that are managed medically, those that are managed surgically, and iatrogenic respiratory distress.
MEDICALLY MANAGED RESPIRATORY DISTRESS
Table 9-3 summarizes plain film diagnosis of respiratory distress in newborns. Use of this approach takes advantage of the fact that only a limited number of changes can be identified radiographically, and a constellation of findings can define a specific group of etiologic factors. A systematic analysis of these various characteristics helps determine a specific group that has a fairly limited differential diagnosis (Box 9-1).
*Idiopathic respiratory distress syndrome (IRDS). | |||||||
Gestational Age | Heart Size | Lung Volume | Nature of Infiltrate | Progression | Ancillary Findings | ||
---|---|---|---|---|---|---|---|
C | Congenital heart disease | Increased | Normal or increased | Increased pulmonary vascularity or edema | Stable or progressive | Abnormal situs, aortic discordance | |
H | Hyaline membrane disease* | <36 weeks | Decreased | Diffuse granularity with air bronchograms | Progressive over first 24 hours | No pleural effusions or body wall edema | |
I | Immature lung | <26 weeks | Normal | Decreased | Diffuse granularity | Progressive | Absent thymus from stress |
M | Meconium aspiration | ≥39 weeks | Normal | Increased | Streaky and patchy | Stable | Air leak (i.e., pneumothorax) |
P | Neonatal pneumonia | Normal or increased | Either diffuse or focal | Pleural effusions and body wall edema |
BOX 9-1
Congenital heart disease
Transient tachypnea of the newborn (resolves over first 24 hours)
Extracardiac shunts
Immature lung
Represents anectasis rather than atelectasis
Meconium aspiration
Amniotic fluid aspiration
Pneumonia
Diffuse
Birth asphyxia
Focal
Pulmonary hemorrhage
Bronchopulmonary dysplasia represents the chronic lung disease that may result from any of the causes of respiratory distress.
SURGICALLY MANAGED RESPIRATORY DISTRESS
Respiratory conditions that are managed surgically can be subdivided into three groups: (1) those associated with aspiration, such as cleft palate, laryngeal cleft, or tracheoesophageal fistula; (2) those that compromise functional lung volume, including congenital diaphragmatic hernia (CDH), congenital lobar emphysema (Figure 9-2), and congenital cystic adenomatoid malformation (CCAM); and (3) those associated with tracheal or bronchial narrowing, such as a double aortic arch, congenital tracheal stenosis, and bronchogenic cyst (Box 9-2).
BOX 9-2
1. Associated with aspiration
a. Cleft palate
b. Laryngeal cleft
c. Tracheoesophageal fistula
2. Compromised functional lung volume involvement
a. Congenital diaphragmatic hernia
b. Congenital lobar emphysema
c. Congenital cystic adenomatoid malformation
3. Cause tracheal or bronchial narrowing
a. Double aortic arch
b. Tracheal stenosis
c. Bronchogenic cyst
IATROGENIC RESPIRATORY DISTRESS
Most iatrogenic respiratory distress results from either a misplaced catheter or tube or from barotrauma. An endotracheal tube (ETT) can be placed too deep and will preferentially ventilate only a single lung. An ETT may even be inadvertently placed into the esophagus, resulting in inadequate ventilation (Figure 9-3), which is further compromised by distention of the esophagus and small bowel, limiting lung expansion.
Air leaks are considered to be the result of barotrauma (Figure 9-4). Although barotrauma occurs much less frequently since the introduction of exogenous surfactant and high-frequency ventilation, air leaks continue to be a problem that causes significant concern. Appropriate ventilation management requires timely and accurate diagnosis. One goal in review of a chest x-ray film is to define the location of the extrapulmonary gas. Abnormal extrapulmonary gas can include any one or a combination of the following: pulmonary interstitial emphysema, subcutaneous emphysema, pneumomediastinum, pneumothorax, pneumopericardium, pneumocardia, and portal venous gas.
FLUOROSCOPY
Background
In fluoroscopy, an x-ray tube similar to that used for plain film radiography is used. The x-ray is generated in the same manner as in plain radiography, but it is received in most cases by a device that is similar to a TV camera or VCR. Fluoroscopy allows real-time evaluation of a patient and can be performed with or without contrast material. Spatial resolution in fluoroscopy is not as good as that in plain film radiography, but it is still excellent. Contrast resolution is about the same: air, fat, water, bone, and contrast are about the only densities that can be separated. Contrast media can be given orally or per rectum, instilled into the urinary bladder, or given intravenously. The contrast attenuates the radiation beam to a variable extent related to physical properties and thickness of the attenuator. Most contrast agents are compounds that use either inert barium or iodine as the attenuator of the radiation beam. The most important characteristic of fluoroscopic imaging is the ability to evaluate motion in real time. This is essential in the evaluation of swallowing function, gastrointestinal (GI) peristalsis, and diaphragmatic motion.
Clinical Utility in the Neonatal Intensive Care Setting
The most common fluoroscopic examinations requested for neonates include the upper GI (UGI) series, the contrast enema, and voiding cystourethrography. The UGI series is useful in the evaluation of swallowing, aspiration, feeding intolerance, vomiting, and abdominal distention with possible bowel obstruction.
A contrast enema can be diagnostic in Hirschsprung’s disease (Figure 9-5). It can be both diagnostic and therapeutic in meconium plug syndrome and meconium ileus.
A voiding cystourethrogram is used to evaluate the urinary bladder and the urethra and to look for vesicoureteral reflux (Figure 9-6), which is associated with urinary tract infection. Vesicoureteral reflux is a common cause of hydronephrosis, which is now frequently identified during prenatal ultrasonography. Ureteroceles, periureteral diverticula, and posterior urethral valves all can be associated with hydronephrosis in the neonatal period and demonstrated with cystourethrography.
![]() |
FIGURE 9-6 Frontal view from a voiding cystourethrogram demonstrates grade II vesicoureter on the left (arrow). |
Air works as a fine contrast agent, and the nasal and oral airway, as well as the trachea and proximal bronchus, can be easily evaluated fluoroscopically. Because the diaphragm is immediately adjacent to aerated lung, diaphragmatic motion and its relationship to inspiratory effort help in the evaluation of phrenic nerve injury and diaphragmatic paralysis. Eventration of the diaphragm also can be evaluated fluoroscopically but, at times, can be indistinguishable from diaphragmatic hernia.
Focused Discussion: Upper Gastrointestinal Series
Indications for performing a UGI series include swallowing dysfunction, aspiration, vomiting, choking, and apnea. An appropriately performed UGI series offers a systematic approach to the upper GI tract. Starting with the patient in a left-side-down recumbent position, deglutition is evaluated. Tongue action, transport, nasopharyngeal regurgitation, aspiration, and laryngeal penetration all can be assessed. The right-side-down position better separates the esophagus and the tracheal air column. However, if this position is used initially and the evaluation of the deglutition is prolonged, the stomach may empty, filling the proximal small bowel and obscuring the location of the ligament of Treitz. The left-side-down position allows one to evaluate swallowing without concern that the stomach may empty prematurely. Once deglutition is satisfactorily evaluated, one can concentrate on the esophagus. Vascular rings and slings are evaluated in both the frontal and lateral positions.
Esophageal atresia usually is diagnosed clinically; plain film observation of intraluminal bowel gas defines the most common form, which is associated with a distal tracheoesophageal fistula. The benefit of a proximal pouch study in esophageal atresia is controversial. There is a small incidence of fistula from the proximal pouch to the trachea; this incidence is independent of the presence or absence of a distal fistula. If the surgical approach to esophageal atresia repair includes direct visualization of the proximal pouch, the pouch contrast study is superfluous. If, on the other hand, esophagoscopy is not routinely performed, there is some value in evaluating the proximal pouch before surgery. In the absence of esophageal atresia, the location of the fistula (H type) is at the thoracic inlet. This is higher than the fistula that occurs in the most common form of esophageal atresia, in which the fistula is at the level of the carina.
The caliber of the esophagus is informative, because it is usually dilated in association with significant gastroesophageal reflux. Esophageal contour, mucosal detail, and peristalsis are assessed. The configuration of the gastroesophageal junction can indicate gastroesophageal reflux, and rare hiatal hernias can be diagnosed. Gastric emptying is evaluated and gastric peristalsis examined. Because the rotation and fixation of the bowel have important consequences in the newborn period, this is an important part of a complete examination. The duodenal bulb, C-loop, and ligament of Treitz are defined. The lateral film is essential in localizing the ligament of Treitz. For proximal bowel rotation and fixation to be considered normal, the duodenal-jejunal junction (ligament of Treitz) must be retroperitoneal and therefore posterior, to the left of the spine, and at the level of the retroperitoneal portion of the second portion of the duodenum (just distal to the duodenal bulb).
The rotation of the proximal bowel may be independent of the rotation of the hindgut. Therefore if the clinical question is malrotation and possible volvulus, the UGI series is the examination of choice. The caliber, contour, and fold pattern of the proximal bowel are evaluated and the transit time observed. This simple, systematic, yet comprehensive approach to a UGI series yields a tremendous amount of information.
ULTRASONOGRAPHY
Background
One of the most prominent mass media introductions of ultrasound (US) technology came when Dr. Robert Ballard located the wreckage of the Titanic using US to explore the ocean floor of the North Atlantic. Medical ultrasonography has its roots in sound navigation and ranging (sonar) developed during World War II.
In medical ultrasonography, a transducer (essentially a piezoelectric crystal) converts electrons into mechanical vibration that creates high-frequency sound waves within the body. The same transducer serves as both the transmitter of the sound wave and the receiver of the reflected sound. Within the body, these high-frequency sound waves propagate through the soft tissues until they meet a reflective surface that reflects some of those fluid waves back to the transducer. The percent of the sound beam reflected relates to the difference in the acoustic impedance of the material being evaluated. When the acoustic impedances of materials are similar, as is the case with the abdominal wall musculature (e.g., liver, kidney), most of the sound is transmitted and a small percent reflected at each interface. As the sound wave travels through the abdominal wall to the liver, the abdominal wall–liver interface reflects a portion of the beam and transmits most of the sound through the liver to the liver-kidney interface. The small difference in acoustic impedance between the liver and kidney causes reflection of some of the beam and transmission of most to the posterior abdominal wall. This allows the visualization of multiple interfaces that are deep to the first structure encountered. If the velocity of the sound beam in tissue is known, the distance to the reflective surface can be estimated by measuring the time it takes for the pulse to travel the distance to and from the object imaged.
Most of the tissues in the body have similar acoustic impedances; however, air has extremely low impedance and bone extremely high impedance.
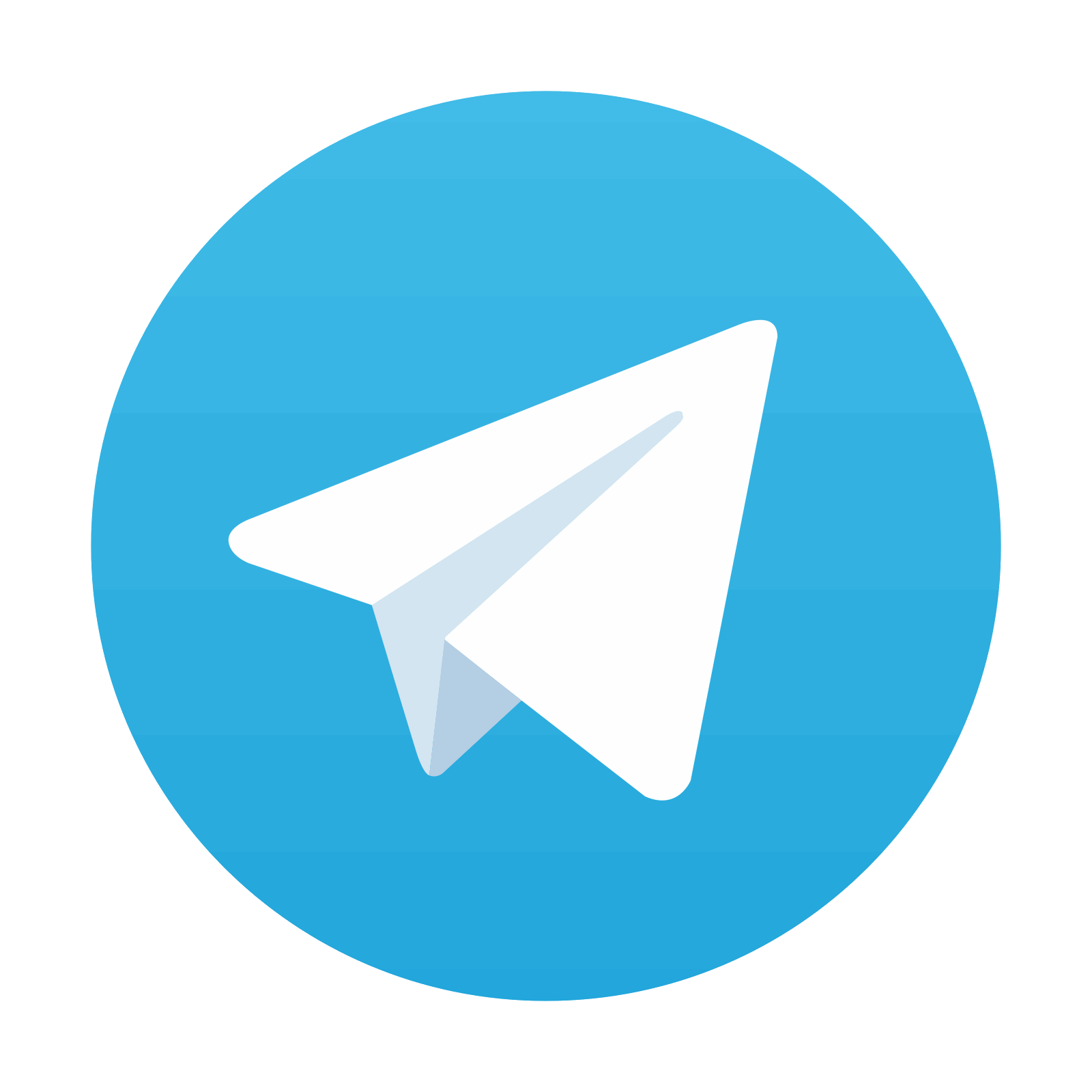
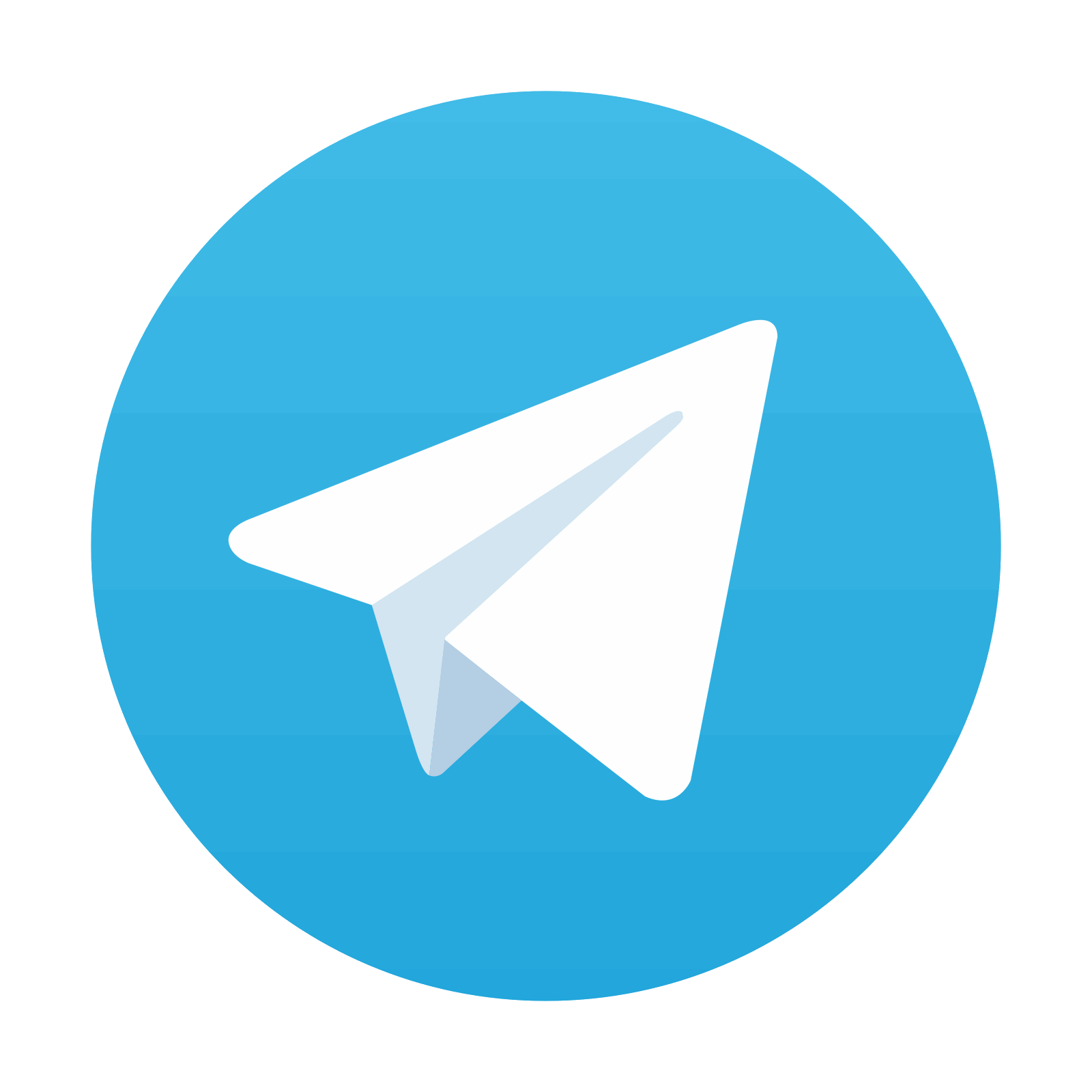
Stay updated, free articles. Join our Telegram channel

Full access? Get Clinical Tree
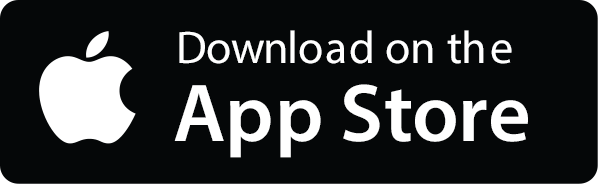
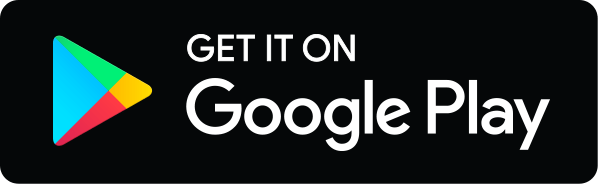
