FIGURE 13.1 Arteriovenous malformation—axial unenhanced computed tomography (CT) (A), axial MIP CT angiography (CTA) (B), axial T2-weighted magnetic resonance imaging (MRI) (C), axial fluid-attenuated inversion recovery (FLAIR) MRI (D). Frontal oblique (E) and oblique lateral (F) angiograms (different patient). (A) Hyperdense area in the right occipital lobe is present (white arrows). (B) CTA demonstrating serpentine enhancing vessels supplying a large nidus. (C) Dilated feeding arteries and nidus appear as flow voids on the T2-weighted image. (D) Areas of increased signal within the nidus on FLAIR imaging, likely representing injury or gliosis (black arrow). (E) and (F) Enlarged right posterior cerebral (white arrow) and pericallosal (black arrow) arteries supplying a right medial temporoparietal nidus. There is rapid shunting into the straight (STS) and transverse sinus (TS) and superficially draining cortical vein (CV).
CT angiography (CTA) often provides valuable information regarding intracranial AVMs and exceeds CT in its ability to diagnose small AVMs (27–28). Feeding arteries, particularly if large, can be identified with considerable precision. In addition, the nidus, draining veins, and surrounding structures are well seen (29). Also, associated lesions including aneurysms are often well-characterized on CTA. The use of 4-dimensional CTA may become an important adjuvant for diagnosis of AVM (30). CTA is however limited in its ability to identify or characterize small aneurysms (less than 5 mm) or intranidal aneurysms (31).
Magnetic resonance imaging (MRI) and angiography (MRA) are most often the best screening techniques (32). Both are excellent for evaluating high flow structures including the feeding arteries, nidus, and draining veins of the AVMs (33). Evidence of arteriovenous shunting is always present in AVMs and may not be optimally evaluated on static imaging modalities such as CT and MRI, but is easily identified with digital subtraction angiography (DSA). The characteristic MRI appearance of AVMs consists of groups of signal voids representing the high flow components of the lesion. Hemorrhage, with the exception of acute subarachnoid hemorrhage, is usually well evaluated on MRI. MRI also provides evaluation of the brain parenchyma for assessment of perilesional edema, gliosis, or infarction which may be associated with the AVM (34). Lastly, hydrocephalus is well evaluated on MRI.
Advanced MRI techniques including functional MRI and diffusion tract imaging have seen an increasing role in pretreatment evaluation of AVMs to determine the tracts (35–37). In addition, the use of arterial spin labeling has in some cases been able to demonstrate the temporal component of arteriovenous shunting necessary to confirm the diagnosis of AVM (38).
DSA remains necessary for complete evaluation of all AVMs. DSA gives the best depiction of arteriovenous shunting through the lesion, thereby confirming the diagnosis of AVM and permitting differentiation from other types of cerebrovascular malformations. In addition, DSA provides most accurate delineation of arterial supply, morphology of the nidus, and evaluation of the venous drainage. DSA is also excellent for identifying aneurysms associated with AVMs (39).
Evaluation of all arterial feeders to the lesion is necessary for complete characterization. This includes injecting all arterial circulations potentially providing contribution to the AVM and may include external carotid as well as internal carotid arteries and the vertebrobasilar system. Arteriovenous shunting with excessively rapid delivery of contrast into the venous system is essential to make a diagnosis of AVM. Consequently, immediate filling of the nidus and venous drainage is characteristic. Appropriate oblique views are necessary to determine the morphology of the nidus and its relationship to intracranial structures. An evaluation of drainage into the superficial and/or deep venous system is also necessary to fully characterize the lesion (40).
Management
Management options for AVMs include observation, surgical resection, radiosurgery, embolization, and combinations of the three latter techniques (41–42). The poorly characterized natural history of AVMs often makes management decisions difficult. At present, evidence from randomized trials comparing different treatments for brain AVMs which might guide the interventional treatment of brain AVMs is lacking (43). Currently an ongoing randomized controlled trial, A Randomized trial of Unruptured Brain Arteriovenous malformations (ARUBA), is comparing interventional treatment versus medical management for brain AVMs that have never bled (44).
Surgical Resection
For many years, surgical treatment was the only effective intervention for AVMs and remains the mainstay of treatment in many cases. The goal of surgical resection is the complete removal of the AVM, which probably reduces the risk of death, hemorrhage, and epilepsy (43). Surgical decision making is aided by various grading systems, the most widely utilized being the Spetzler-Martin grading system.
The Spetzler-Martin grading system is designed to determine the risk of surgery for AVM resection. The system evaluates three features of the AVM and assigns a score to each feature. The numerical value of each feature is added together to determine the surgical grade of the AVM. Features evaluated to grade an AVM using this system include the size of the nidus, whether or not there is drainage into the deep venous system, and whether or not eloquent brain is involved by the AVM. Eloquent brain is defined as primary sensorimotor, language, visual cortex, hypothalamus and thalamus, internal capsule, brainstem, cerebellar peduncles, and the deep cerebellar nuclei (17).
A number of studies have been published relating the estimated mortality and morbidity associated with surgical resection to the grade of the AVM (45–46). However, significant surgical issues remain unaddressed by grading systems. These include the timing of surgery and the combination of surgery with other AVM intervention techniques including radiosurgery and embolization (47–50). The vast majority of AVM resections are performed on an elective basis. This is almost always the case in nonhemorrhagic presentations. In addition, most authors advocate elective resection of AVMs following hemorrhage, usually weeks after a hemorrhage has occurred. Delayed resection permits liquification of acute clot, with formation of a discrete cavity in the region of prior hemorrhage, often minimizing brain retraction and aiding resection. Nevertheless, early surgery may be required in patients with large hematomas to relieve mass effect and minimize progression of neurologic damage. The goal of early surgery may be complete resection of AVM and hematoma or may be restricted to removal of the acute hematoma.
Radiosurgery
Three radiosurgical techniques are commonly in use for treatment of AVMs. These include the Gamma knife (51), linear accelerator (52), and particle beam therapy (53). All three techniques deliver multiple radiation beams, consisting of gamma rays, high-energy photons, and charged particles respectively. The beams are targeted to converge on an isocenter, which is confined to the nidus of the AVM, thereby delivering a relatively high dose to that region with minimal dose to normal surrounding structures. The high isocenter dose damages the nidus vessels with subsequent smooth muscle proliferation, fibrosis, and obliteration of the nidus (54). Development of these changes requires a 2 to 5 year latent period for full effect. During the latent period, hemorrhage risk persists.
Obliteration rates following radiosurgical treatment are highly dependent on the size of the nidus treated and location (55). Radiosurgery obliterates 65% to 85% of AVMs under 3 centimeters and is best for treating AVMs with small, compact nidi (56). Lower obliteration rates are the rule for lesions exceeding 3 centimeters in diameter, limiting the use of radiosurgery for the treatment of large AVMs (57).
Embolization of AVMs
Embolization of AVMs involves selective endovascular catheterization of arterial feeders to the AVM with injection of embolic material into the nidus. The technique is designed to obstruct or obliterate flow within the vessels comprising the nidus and is generally performed to reduce the size and flow through the nidus prior to surgical resection or radiosurgical therapy, thereby making treatment safer or extending treatment options to lesions otherwise not amenable to therapy. It may also be performed to palliate or reverse progressive symptoms (58) or to selectively obliterate high risk features associated with the AVM (40).
In approximately 5% to 10% of cases, embolization alone may result in complete obliteration of an AVM. The situation usually occurs in a setting of one or two direct feeders to a relatively small AVM (59). However, with the use of newer microcatheters, angiographic obliteration of the nidus after embolization may occur in up to 55% of cases (60).
Embolic materials utilized for AVM embolization include polyvinyl alcohol particles (PVAs) (61) and liquid embolic agents, including n-butyl cyanoacrylate (n-BCA) and Onyx (62). The liquid agents are most commonly used, and have the advantage of more permanent obliteration of the embolized components of the AVM. In addition, one study found a significantly higher rate of postembolization hemorrhage in patients embolized using PVA compared with n-BCA (63).
Complications of neuroendovascular AVM treatment consist primarily of ischemic and hemorrhagic events. Reported complication rates vary considerably, ranging from 4% to 14% for morbidity and 1% to 4% for mortality, likely reflecting differences in patient selection, embolization technique, and operator experience (64–65).
■ CAVERNOUS MALFORMATION
Epidemiology
Cavernous malformations represent from 5% to 15% of cerebrovascular malformations (66–68) with approximately one-fourth of cavernous malformations occurring in the pediatric population (69–70). Associated with an adjacent developmental venous anomaly (DVA) approximately 25% of the time (see below), cavernous malformations affect males and females with equal frequency, and are believed to have a prevalence of 0.02% to 0.9% in the general population (66,71–73). The incidence may be higher, however, as the increasing use of MRI has resulted in increased detection of asymptomatic lesions, with at least 40% detected incidentally in one study (68). Cavernous malformations may be single or multiple with multiplicity seen in up to 25% of sporadic cases. However, multiplicity also suggests a familial trait as 6% to 50% are associated with familial clustering with a higher prevalence noted in Mexican Americans (72,74). For unknown reasons, children with cavernous malformations tend to present in a bimodal pattern, at ages 0 to 2, and 13 to 16 years of age (75).
Morphology
Pathologically, cavernous malformations consist of unencapsulated collections of thin-walled sinusoidal channels separated by a collagenous stroma without mature vascular wall components (72). No normal brain parenchyma is found within cavernous malformations. Exceedingly slow blood flow and various stages of clot formation are found within the channels. Considerable fibrosis and collagen is present within the vessel walls and calcification may be present. Cavernous malformations are characterized by a complete rim of iron storage products surrounding the lesions and exert minimal mass effect in the absence of acute hemorrhage.
Cavernous malformations typically occur in subcortical locations of the cerebral hemispheres. The temporal and frontal lobes are most commonly involved, with approximately 10% of the lesions involving deep central regions of the brain. An infratentorial location is identified in approximately 20% of the lesions, with the majority of these affecting the brain stem, most commonly the pons. The spinal cord may be involved in up to 5% of cases and the lesions rarely may affect the third ventricle or be found extra axially.
Clinical
Cavernous malformations are most commonly asymptomatic, causing symptoms in approximately 15% of patients. When symptoms are present, they depend on the size and location of the lesion (76). Twenty-three percent to 79% of symptomatic supratentorial lesions give rise to seizures (74). Compared to other parenchymal lesions of similar volume and location, cavernous malformations are twice as likely to present with seizures (72). Focal neurologic deficits or gait ataxia are common presenting symptoms in patients with infratentorial lesions (74) and brain stem cavernous malformations may give rise to truncal ataxia, facial nerve paresis, dysphagia, diplopia, arm or leg weakness, numbness, dizziness, nausea, vomiting, or hiccups (77). Approximately half of symptomatic cases present with nonhemorrhagic neurologic deficits, and most patients present with multiple neurologic deficits (78). Hemorrhage is much less common when compared to AVMs, and is the presenting symptom in approximately 20% of cases, although some have reported a higher incidence of hemorrhage in the pediatric population (79–80).
Imaging
Cavernous malformations demonstrate characteristic features on MRI which most often permit confident noninvasive diagnosis (Figure 13.2). T2-weighted and gradient echo images demonstrate a complete rim of hypointense signal secondary to iron storage products (81–82). The central portion of the lesion is characterized by heterogeneous intensity, reflecting various stages of blood products. Minimal mass effect is present and no evidence of edema except in the setting of acute hemorrhage (83). Gradient echo images are most sensitive for detection of cavernous malformations and should be performed in every case as they can often identify lesions not appreciated on other sequences (84). Similar but less conspicuous features are identified on T1-weighted images (83). Minimal or no enhancement is present (85–86).
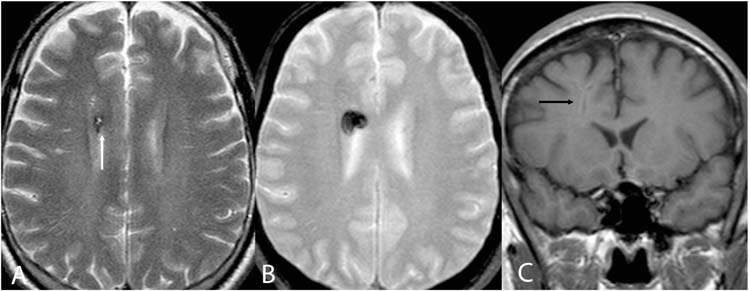
FIGURE 13.2 Cavernoma—axial T2-weighted (A), axial T2* gradient echo (B), and axial T1-weighted (C) images demonstrating a right frontal cavernoma with central heterogeneous signal completely surrounded by a hypointense rim (white arrow). Susceptibility artifact (“blooming”) is present on the T2* gradient echo images. Post contrast coronal T1-weighted images demonstrate no enhancement. Notice the vertically oriented flow void characteristic of an associated developmental venous anomaly.
CT is much less sensitive than MRI in the detection of cavernous malformations. CT findings include areas of hyperdensity and occasional calcification (82). Mass effect and edema are most often absent on CT in the absence of hemorrhage (87).
Angiographic evaluation is rarely indicated in the evaluation of cavernous malformation. The study is generally performed to exclude an AVM in the setting of acute hemorrhage. The extremely slow flow through cavernous malformations accounts for them being angiographically occult.
Management
There is no role for endovascular management and cavernous malformations. Treatment, if indicated, is generally surgical resection.
■ DEVELOPMENTAL VENOUS ANOMALIES
Epidemiology
DVAs, also known as venous angiomas, are the most commonly identified incidental cerebrovascular malformation, with a prevalence of approximately 2% (88). They are believed to represent approximately 60% of cerebrovascular malformations detected at autopsy (89).
Morphology
DVAs represent anatomic variants of physiologically normal venous architecture. They consist of multiple dilated thin-walled vessels diffusely distributed in the normal white matter (90) that drain into an enlarged central trunk (91). The central trunk, in turn, drains into either the superficial or less often, into the deep venous system. DVAs occur in the subcortical white matter of the cerebrum, most often the frontal and parietal lobes (91). From 14% to 29% are located in the cerebellum (92). They are less often found in the basal ganglia (93) or brainstem (94). DVAs provide venous drainage to normal brain parenchyma within their distribution.
Imaging
DVAs have a characteristic MRI appearance, best evaluated on gadolinium enhanced T1-weighted images, as the dilated medullary venous system and enlarged central trunk are well seen (95). T2-weighted images may demonstrate small areas of the signal abnormality and signal void within the dilated central trunk secondary to flow related signal loss (96). No mass effect or edema is present (Figure 13.2C).
Angiographic evaluation of DVAs is rarely indicated. When MRI cannot differentiate DVA from AVM, angiography may be used to exclude AVM. Angiography shows filling of the DVA at the time of the normal venous drainage with no evidence of the characteristic arteriovenous shunting that characterizes an AVM (Figure 13.3).
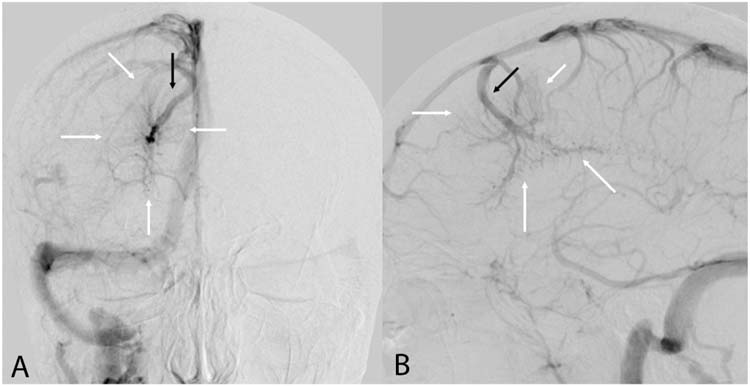
FIGURE 13.3 Developmental venous anomaly—Frontal (A) and lateral (B) angiograms in the venous phase demonstrating dilated medullary veins (white arrows) draining into enlarged central collecting vein (black arrow). The collecting vein then drains into the superior sagittal sinus. The dilated medullary veins appear simultaneously with normal venous structures, characteristic of a developmental venous anomaly.
Treatment
The vast majority of DVAs remain asymptomatic throughout life and require no treatment. Since they function as drainage pathways for normal brain, resection of DVAs may result in significant venous compromise or venous infarction (90).
■ CAPILLARY TELANGIECTASIA
Epidemiology
Capillary telangiectasias have an estimated prevalence of 0.4% at autopsy (97) and are believed to represent approximately 10% to 20% of cerebrovascular malformations (98–99).
Morphology
Telangiectasias are composed of numerous capillary-like vessels that lack smooth muscle or elastic fibers and are separated by normal parenchyma (99). There is little or no gliosis associated with the lesions. The vast majority occur in the pons, however they have been rarely reported in other locations throughout the brain and spinal cord (100–103).
Imaging
Telangiectasias are usually incidentally seen and are virtually always asymptomatic, almost never requiring treatment (Figure 13.4). They are generally occult on imaging except for MRI. Although variable signal characteristics have been described, capillary telangiectasias are generally isointense to hypointense on T1-weighted images and isointense to hyperintense on T2-weighted images most commonly in the midpons (104). Homogeneous or mildly heterogeneous enhancement is typically present and gradient echo images demonstrate focal hypointensity. Occasionally an enhancing adjacent vessel may be identified (100). There is no associated edema or mass effect. Rarely capillary telangiectasias can be seen as an area of focal enhancement on CT, sometimes with associated calcification (105).
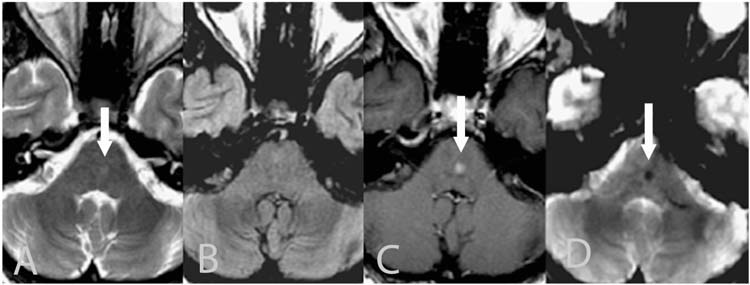
FIGURE 13.4 Capillary telangiectasia—axial T2-weighted (A), axial FLAIR (B), gadolinium enhanced axial T1-weighted (C), and axial T2* gradient echo (D) images demonstrating mild increase signal in the mid pons (white arrow) with no significant signal abnormality on FLAIR images. There is mild homogenous enhancement (white arrow) on the gadolinium enhanced post contrast images. Susceptibility artifact (blooming) is present on the T2* gradient echo images (white arrow).
■ ANEURYSMAL MALFORMATION OF THE VEIN OF GALEN
Epidemiology
Aneurysmal malformations of the vein of Galen consist of direct arteriovenous fistulas between an embryonic choroidal artery and an embryonic venous precursor to the vein of Galen. Aneurysmal malformations of the vein of Galen are rare, constituting less than 1% of all intracranial vascular malformations. They are however of significant importance in very young children, and represent nearly 30% of vascular malformations presenting in the neonatal and infant age groups (106). The lesions are congenital, but no convincing evidence of heritability has been demonstrated.
Anatomy
Aneurysmal malformations of the vein of Galen are located in the subarachnoid space within the cistern of the velum interpositum and quadrigeminal plate cistern (107), thus differentiating them from pial AVMs and fistulas as well as from dural arteriovenous fistulas.
Two anatomic types of AMVG have been described (108–109). The choroidal type consists of numerous arteriovenous fistulas, often with extremely high blood flow through the shunts, which may represent the major portion of cardiac output. Choroidal AMVGs often present in the neonatal period. Mural type AMVGs consist of a few fistulas or even a single fistula, are characterized by lower arteriovenous shunt volumes, and usually present in infancy or even later in life (110).
Clinical
The volume of arteriovenous shunting through the AMVG is believed to be the major contributor to the clinical presentation of AMVG. Three age-specific clinical presentations have been described (111–112). Neonates, usually with choroidal type AMVG and large shunt volumes, experience high myocardial workload, often with decreased myocardial perfusion. Consequently, neonates usually present with high output heart failure. Infants and young children, usually with mural type AMVG, often present with macrocephaly, hydrocephalus, and developmental delay. Uncommonly, older children and even adults may present with headaches or subarachnoid hemorrhage due to AMVG.
A cranial bruit may frequently be auscultated, particularly in neonates with an open fontanelle. Intracranial hemorrhage associated with AMVG is uncommon in neonates and young infants. Intracranial hemorrhage in this age group may be due to an alternate diagnosis, including a pial AVM draining into the deep venous system (113–114).
Imaging
Unenhanced CT scans usually demonstrate the dilated venous drainage pathway as an isodense or hyperdense structure posterior to the third ventricle (115). Careful examination of the brain parenchyma is necessary to identify areas of gliosis or encephalomalacia which may contraindicate treatment. Evaluation for hydrocephalus, the most common associated imaging finding (116), should also be performed.
MRI combined with MRA sequences are usually the most important preangiographic evaluation in children with AMVG (115). Areas of signal void depict both the enlarged feeding arteries and dilated venous drainage that comprises the lesion (Figure 13.5A, 13.5B and 13.5C). As with CT, careful examination of the brain parenchyma on MRI is necessary. MRA sequences, particularly with 3-dimensional reconstruction, often provide the best imaging to outline the anatomy of the lesion (Figure 13.5D). This information is most often useful to plan angiographic evaluation at the time of endovascular treatment.
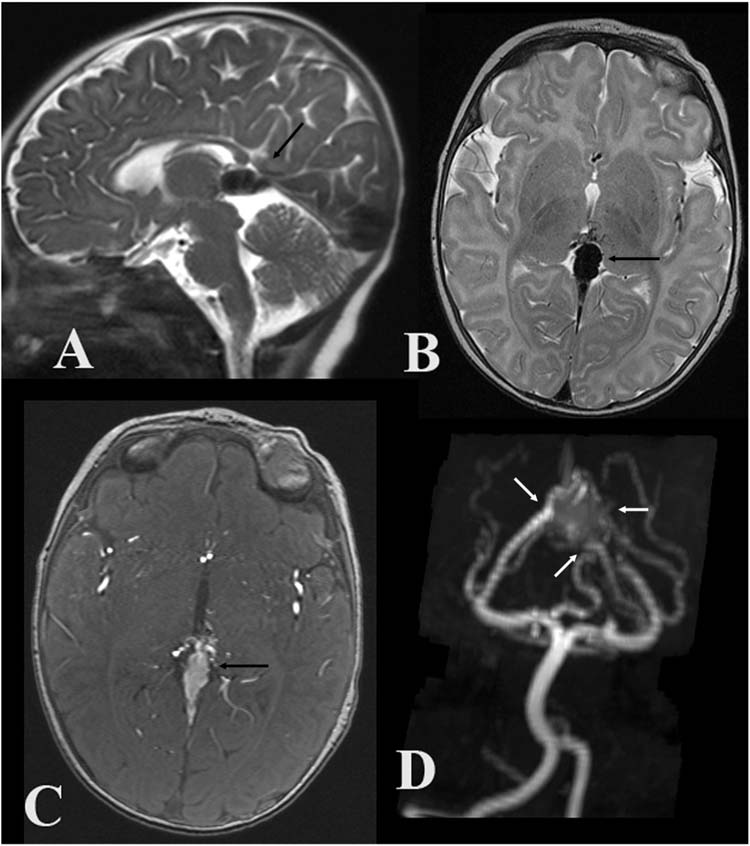
FIGURE 13.5 Aneurysmal malformation of the vein of Galen—midline sagittal T2-weighted (A), axial T2-weighted (B), axial magnetic resonance angiographic (MRA) source images (C), and coronal oblique MRA MIP images (D) demonstrate a large flow void in the cistern of the velum interpositum and quadrigeminal plate cistern (black arrows) fed by multiple branches of the posterior cerebral arteries (white arrows).
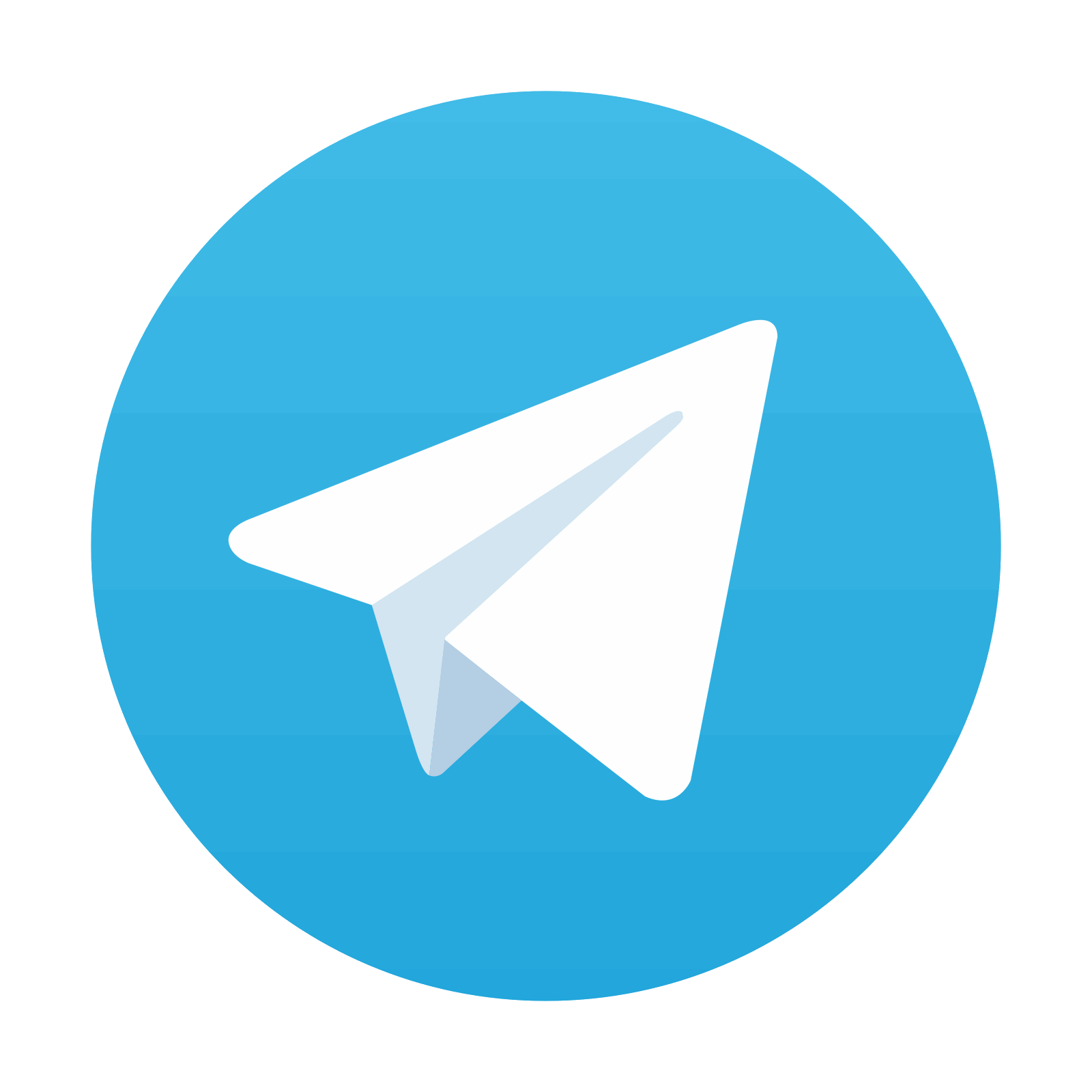
Stay updated, free articles. Join our Telegram channel

Full access? Get Clinical Tree
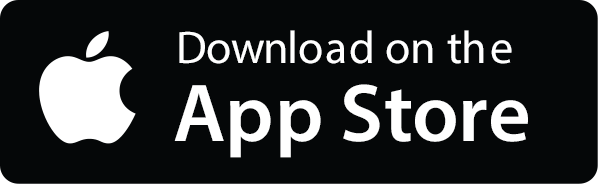
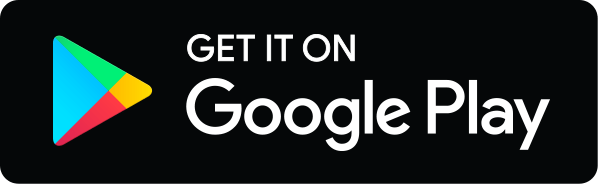