9 Sarah N. Ali1 and Anne Dornhorst2 1 Department of Diabetes and Endocrinology/General Internal Medicine, Royal Free London NHS Foundation Trust, London, UK 2 Division of Diabetes, Endocrinology and Metabolism, Hammersmith Hospital, London, UK Diabetes in pregnancy poses major health risks to both the mother and the growing fetus, and influences the future health of the child [1–4]. The risk of diabetes to pregnancy outcome increases with increasing maternal hyperglycaemia [5,6], and thus the risk from pre‐gestational diabetes, whether type 1 diabetes (type 1 DM) or type 2 diabetes (type 2 DM), is greater than gestational diabetes mellitus (GDM) which develops during the pregnancy [7]. In 1989 the St Vincent Declaration pledged to improve pregnancy outcomes for women with diabetes to those of non‐diabetic women [8]. Despite major advances in obstetric, neonatal and diabetic care, this has not happened. While some improvement for women with type 1 DM have been reported, in general congenital abnormalities, stillbirths and perinatal deaths remain twofold to fourfold higher among women with pre‐gestational diabetes [9]. Recent years has seen a rapid rise in type 2 DM among women of childbearing age who have additional risk factors that adversely affect pregnancy, including high maternal age, weight, parity, levels of social deprivation and belonging to non‐white ethnic minority groups [6,10,11] (Fig. 9.1). These risk factors are similar to those associated with GDM. Because of changing demographics in European urban cities, women with pre‐gestational type 2 DM now outnumber those with type 1 DM while those with GDM account for the majority of women attending an obstetric diabetes antenatal clinic [7,12]. Fig. 9.1 Venn diagram illustrating the relationship between the demographics of the antenatal population and the risk factors for diabetes and those for adverse pregnancy outcomes. The consequences of a diabetic pregnancy in women with type 1 DM, type 2 DM or GDM extend beyond the pregnancy. Approximately 40% of all births to women with pre‐gestational diabetes have evidence of growth acceleration, with approximately half above the 90th percentile for gestational age. High birthweight and infant adiposity is associated not only with shoulder dystocia and caesarean section rates above 60% but also with early‐onset obesity, metabolic dysfunction and diabetes in the child in later life []. Optimal glycaemic control prior to conception and throughout pregnancy improves pregnancy outcome [6,16,17]. All professionals caring for women of childbearing age with either known diabetes or at risk of GDM need to emphasize the importance of pregnancy planning and good glycaemic control prior to pregnancy and the need for GDM screening for those at risk. This chapter covers the clinical management of pregnancies complicated by pre‐gestational diabetes, as well as the screening and management of GDM. Finally, the long‐term health consequences for the child of a diabetic pregnancy are discussed. The diabetic and obstetric management is influenced by the type of diabetes. The main types of diabetes seen in obstetric practice are shown in Table 9.1. Table 9.1 The types of diabetes encountered in obstetric practice. Diabetes in pregnancy can be defined as pre‐gestational (i.e. pre‐existing) diabetes or GDM. Most women with pre‐gestational diabetes have type 1 or type 2 DM. However, a small percentage have monogenetic or mitochondrial forms of diabetes that require genetic screening for correct diagnosis. The importance of recognizing this cohort of women is that their pregnancy outcomes are determined as much by their genetic mutation as by their diabetic control [18–20]. GDM is defined as diabetes or glucose intolerance occurring or first recognized in pregnancy, a definition that includes those with previously unrecognized diabetes [21]. The numbers of women with pre‐gestational DM and GDM is increasing, with the largest proportional rise in those with pre‐gestational type 2 DM and GDM, driven predominantly by the rise in obesity [22]. There is also a slower rise in the prevalence of type 1 DM, whose incidence is estimated at 3–4% per annum in Europe [23]. The prevalence of GDM across Europe in 2012 varied between 2 and 6% [24], influenced by the demographics of the antenatal population and the diagnostic criteria used [25]. This prevalence is anticipated to increase as more universal screening and testing for GDM is introduced. Annual audit data from England and Wales in 2015 estimated that approximately 35 000 pregnant women had either pre‐gestational DM or GDM, the latter making up approximately 80% of cases [26]. While GDM typically arises in the late second trimester due to physiological changes in maternal carbohydrate metabolism [27], the number of women with undiagnosed type 2 DM becoming pregnant will increase as the age of onset of type 2 DM falls [28]. It is important to recognize this high‐risk group as the clinical management needs to be similar to women with pre‐existing type 2 DM. While the definition of GDM is not controversial, how and who to screen for GDM remains contentious [29]. Outside pregnancy the diagnosis of diabetes is made by [18]: The distinction between type 1 and type 2 DM can usually be made clinically based on history and recognized risk factors; when there is uncertainty, testing for the specific pancreatic islet autoantibodies GAD, IA‐2 and ZnT8 can help confirm the diagnosis of type 1 DM in approximately 80% of cases. It is increasingly recognized that new‐onset type 1 DM after the age of 30 years may account for up to 50% of all type 1 DM in the white population. Two large randomized controlled trials, one Australian and the other Canadian, have provided evidence that diagnosing and treating GDM improves pregnancy outcomes. These two randomized trials used different diagnostic criteria for GDM and randomized women between 24 and 34 weeks’ gestation to active management with diet, glucose monitoring and insulin if required or to routine antenatal care [16,17]. The Australian trial of 1000 women diagnosed by World Health Organization (WHO) criteria for impaired glucose tolerance showed that serious perinatal complications were lower among the 490 pregnancies in the intervention group than among the infants of the 510 women assigned to routine care (1% vs. 4%; relative risk adjusted for maternal age, race or ethnic group, and parity). The intervention group had a 10% higher rate of induction of labour with a similar caesarean section rate compared with women receiving routine care [16]. The Canadian study of 958 women identified as having mild gestational diabetes showed that active treatment reduced mean birthweight (3302 vs. 3408 g), neonatal fat mass (427 vs. 464 g), frequency of large‐for‐gestational‐age infants (7.1% vs. 14.5%), birthweight greater than 4000 g (5.9% vs. 14.3%), shoulder dystocia (1.5% vs. 4.0%), and caesarean delivery (26.9% vs. 33.8%). Active treatment was also associated with reduced rates of pre‐eclampsia and gestational hypertension [17]. The Hyperglycemia and Adverse Pregnancy Outcome (HAPO) study was designed to establish internationally agreed diagnostic criteria for GDM [5]. This observational study analysed over 23 000 non‐diabetic pregnant women between 2000 and 2006 from nine countries. All women underwent a 2‐hour 75‐g OGTT between 24 and 32 weeks’ gestation. The results were analysed to clarify associations between maternal glucose values (below those diagnostic of diabetes) and four primary perinatal outcomes: macrosomia (corrected birthweight >90th centile), primary caesarean delivery, clinical neonatal hypoglycaemia and hyperinsulinaemia (assessed by cord serum C‐peptide >90th centile for the whole study group). Analysis showed a continuous gradient between maternal glucose levels (at fasting, 1 hour and 2 hours post 75‐g glucose load) and all four primary outcomes. Using these results new diagnostic criteria for GDM were drawn up by the International Association of Diabetes and Pregnancy Study Groups (IADPSG) that have been subsequently endorsed by the American Diabetes Association (ADA) and WHO [30]. However, the IADPSG criteria for GDM differ from those recommended in 2015 by the National Institute of Health and Care Excellence (NICE) in England that proposed a higher fasting value, a lower 2‐hour value, and no 1‐hour value. The NICE criteria were based on published intervention studies and cost‐effective analyses. The IADPSG and NICE criteria for GDM diagnosis are summarised in Table 9.2. Table 9.2 The IADPSG and NICE criteria for GDM diagnosis. The decision to screen universally for GDM or only perform the test in women with recognized risk factors remains unresolved. The NICE criteria advocate using universal screening based on risk factors identified at the initial antenatal visit, which includes body mass index (BMI) above 30 kg/m2, previous macrosomic baby weighing 4.5 kg or above, previous GDM, family history of diabetes (first‐degree relative with diabetes) and a minority ethnic family origin with a high prevalence of diabetes. Only women with one or more of these factors are advised to have a 75‐g OGTT between 24 and 28 weeks [26]. An OGTT at 16 weeks is also recommended for women with a history of GDM, which should be repeated at 24–28 weeks if the initial test is normal. The list of risk factors recommended by NICE is not exhaustive as they omit maternal age [31,32] and polycystic ovary syndrome [33]. This perhaps explains why using NICE risk factor screening alone can miss up to 25% of women identified with GDM on an OGTT [29,34]. The frequency of GDM among the original HAPO cohort using the IADPSG criteria was 17.8% (9.3–25.5%) depending on the country of origin [25]. The IADPSG criteria diagnose women with a fasting glucose between 5.1 and 5.6 mmol/L and these women are missed using the NICE criteria; 38% of these pregnancies result in a large‐for‐gestational‐age infant despite the 2‐hour value being below 7.8 mmol/L, this group of women tending to be obese [35]. The debate on the benefits of treating women with lesser degrees of glucose intolerance than identified by the NICE criteria continues [36]. The 2002 CEMACH audit of 3800 pregnancies in England, Wales and Northern Ireland showed similar fetal complications (perinatal mortality, stillbirth and neonatal mortality) among women with type 1 and type 2 DM, emphasizing that the major determinant of fetal complications is maternal hyperglycaemia rather than type of diabetes [6] (Fig. 9.2). Maternal and social risk factors that can impact on pregnancy do nonetheless differ between women with type 1 DM and those with type 2 DM, and include being more obese, socially deprived and less prepared for pregnancy. This is especially true among adolescents and young adults with type 2 DM, a particularly vulnerable group whose pregnancy outcomes are extremely poor, with fetal malformation rates in excess of 20% [37]. Women with type 1 DM are at greater risk of recurrent and severe hypoglycaemia and retinopathy. Fig. 9.2 Data from the Confidential Enquiry into Maternal and Child Health (CEMACH) Diabetes Audit. PNMR, perinatal mortality rates, stillbirth rates and neonatal death rates for mothers with type 1 and type 2 DM (delivered or booked between 1 March 2002 and 28 February 2003 in England, Wales and Northern Ireland). Maternal age adjusted: *, per 1000 total births; **, per 1000 live births [6]. Maternal hyperglycaemia affects all aspects of pregnancy, from fertilization and implantation to birth (Fig. 9.3). There is growing evidence that maternal hyperglycaemia, via fetal programming, can cause changes in gene expression that increase the fetus’s future susceptibility to obesity, diabetes and other health problems [3,38]. Hence a principal tenet for management of diabetes in pregnancy is to strive for euglycaemia throughout the whole of pregnancy. Congenital abnormalities, miscarriage, accelerated fetal growth, late stillbirth, birth trauma and neonatal hypoglycaemia all increase as maternal glycaemia rises (Table 9.3). This risk can be further increased by the presence of obesity [39]. Fig. 9.3 Maternal hyperglycaemia affects all aspects of pregnancy from fertilization and implantation to birth. Table 9.3 The role of maternal hyperglycaemia on maternal and fetal complications. DAG, diacylglycerol; PKC, protein kinase C. In a systemic review involving 1977 diabetic pregnancies from seven USA cohort studies between 1986 and 2006, the mean fetal malformation rate rose from 3% to 10% as peri‐conception HbA1c levels rose from two standard deviations (2SD) to 8SD above the normal 2% range, respectively (Fig. 9.4) [40]. This strong correlation between peri‐conception glycaemic control and congenital anomaly was also seen in England among 1677 births to diabetic women between 1996 and 2008 [41]. High peri‐conception HbA1c values are also a predictor of stillbirth [2,6]. Fig. 9.4 The relationship between congenital abnormalities and antenatal glycosylated haemoglobin (HbA1c). Alongside glycaemic management, screening for and treating specific diabetic complications is also important. The association between pre‐pregnancy levels of glycaemia and congenital malformations begins at the upper level of the normal non‐diabetic range [41]. The teratogenic effect of hyperglycaemia occurs in the first 12 weeks of gestation at the time of blastocyst formation, embryogenesis and organogenesis. In order to significantly limit early fetal loss and congenital abnormalities women need optimal glycaemic control prior to pregnancy. To achieve this, women with pre‐gestational diabetes ideally need to plan their pregnancies, be given pre‐conception counselling and continue contraception until good glycaemic control has been achieved [26]. As half of all pregnancies are unplanned, discussing pregnancy planning should be an integral part of all diabetic reviews for women of childbearing age, whether in primary or secondary care [42]. Congenital malformations and preterm delivery are reduced when women receive pre‐conception counselling [43]. These clinics provide a supportive environment to discuss pregnancy care pathways, emphasize the need and timing for increased fetal and maternal surveillance, and offer reassurance that good glycaemic control can reduce the potential risks of a diabetic pregnancy. Glycaemic control can be intensified, high‐dose folic acid supplements (5 mg) prescribed and potentially teratogenic drugs, such as angiotensin‐converting enzyme (ACE) inhibitors, angiotensin receptor blockers and statins (HMG‐CoA reductase inhibitor), stopped or switched to safer alternatives [44,45] (Table 9.4). Diabetic complications can be assessed as can the need for aspirin or heparin for women at high risk of pre‐eclampsia or thrombophilia or who have significant proteinuria (>4 g per 24 hours) [26]. Unfortunately, women with type 2 DM, those from ethnic minority groups and those with the highest social deprivation scores are less likely to access these services [46]. Table 9.4 Key topics to discuss with all women of childbearing age with diabetes. The level of glycaemic control required from pre‐conception to birth mandates continual blood glucose monitoring and dose adjustment of insulin. Providing education and training to women with pre‐gestational diabetes to enable them to self‐manage and adjust their own insulin to match their carbohydrate intake is key to improving glycaemic control and reducing the risk of hypoglycaemia [47–52]. Based on the association between HbA1c and major fetal anomalies, the 2015 NICE guidance strongly advises women with an HbA1c above 86 mmol/mol (10%) not to become pregnant, while recommending an HbA1c below 48 mmol/mol (6.5%) if achievable without causing problematic hypoglycaemia [6]. For women with type 1 DM, current recommended targets are as follows: fasting plasma glucose of 5–7 mmol/L on waking and a plasma glucose of 4–7 mmol/L before meals at other times. For women with type 1 DM to achieve this level of glycaemic control requires four to five daily insulin injections (multiple‐dose insulin, MDI) or a continuous subcutaneous insulin infusion (CSII) pump. With the MDI regimen, a long‐acting basal insulin analogue is usually given at night or as a split morning and evening dose, with rapid‐acting bolus insulin given with each meal. The recent advances in insulin pump technology, glucose monitoring and bolus insulin calculators that recommend a rapid‐acting insulin dose based on the amount of carbohydrate may all assist women achieve better control. In the future, advances in the technologies of closed‐loop insulin delivery, which combines real‐time continuous glucose monitoring (CGM) with CSII using a computer algorithm, have the potential to normalize glycaemic control while reducing the risk of hypoglycaemia [53–55]. For women with type 2 DM, achieving an HbA1c below 48 mmol/mol (6.5%) prior to pregnancy is easier, as these women are at less risk of hypoglycaemia [56]. While metformin can be continued in pregnancy, women previously on other oral hypoglycaemic agents, such as sulphonylureas, will need to be switched to insulin using MDI regimens. Diabetic complications differ in women with type 1 DM and type 2 DM. Women with type 1 DM are more likely to have had diabetes for longer and since diabetic complications increase over time diabetic microvascular disease is more common in women with type 1 DM than those with type 2 DM. This may change as the onset of type 2 DM continues to fall. Pre‐pregnancy counselling reduces the progression of both diabetic retinopathy and nephropathy in pregnancy. Women with long‐standing type 1 DM and older obese women with type 2 DM and previous GDM are potentially at increased risk of diabetic macrovascular disease, including coronary heart disease. A dilated retinal examination should be performed prior to pregnancy, in early pregnancy and again at 28 weeks’ gestation if no retinopathy was detected initially. However, in the presence of retinopathy a check is required at 16 weeks in addition to the one at 28 weeks’ gestation. The risk factors for the development or worsening of retinopathy are pre‐existing hypertension, duration of diabetes and a rapid fall in HbA1c between the first and third trimester. However, diabetic retinopathy should not be considered a contraindication for rapid optimization of glycaemic control in pregnancy or a contraindication to pregnancy or a vaginal birth. As duration of type 1 DM increases, background and proliferative diabetic retinopathy increases. Women with type 2 DM are more prone to diabetic maculopathy, which is less duration dependent. Rapidly improving glycaemic control during the pre‐conception period and in pregnancy can worsen retinopathy, although long‐term detrimental effects are rare post partum. Laser treatment before pregnancy protects against retinopathy progression in pregnancy and if needed should be performed before conception. When diabetic retinopathy develops de novo in pregnancy, it is usually not severe and laser treatment is safe if required [57–59]. An assessment of renal function, comprising urinary protein/creatinine ratio and estimated glomerular filtration rate (eGFR), should be performed prior to pregnancy and in early pregnancy. The presence of proteinuria and/or reduced eGFR is a significant risk factor for nephrotic syndrome, hypertension, pre‐eclampsia, placental insufficiency, preterm delivery and neonatal morbidity and mortality [60]. Intensive glycaemic control before and throughout pregnancy, low‐dose aspirin from 12 gestational weeks, and intensive antihypertensive treatment can reduce these risks [61]. During a healthy non‐diabetic pregnancy, structural, functional and hemodynamic changes to renal physiology occur; by term, eGFR is 40% higher, with an increase seen from 12 weeks alongside an associated rise in creatinine clearance and protein excretion rate [62]. Women with normal or mild renal impairment before pregnancy usually preserve their renal function post partum and can anticipate a successful pregnancy outcome. When microalbuminuria is present that is not attributable to a urinary tract infection, serial urinary protein/creatinine ratios should be performed throughout the remaining pregnancy to quantify the degree of proteinuria. Those with moderate to severe diabetic nephropathy [serum creatinine >124 µmol/L (1.5 mg/dL) or proteinuria >3 g per 24 hours] are most at risk of perinatal death and irreversible deterioration in renal function after pregnancy (especially with serum creatinine >176 µmol/L). If accelerated progression of nephropathy occurs in pregnancy, renal intervention with dialysis may be required [61,63]. A pre‐conception microalbumin measurement is also helpful as it assists with the diagnoses of pre‐eclampsia, which can be difficult to distinguish from diabetic nephropathy in later pregnancy. Diabetic neuropathy can manifest as either a peripheral sensory neuropathy or an autonomic neuropathy and both increase with duration of diabetes [64]. Diabetic autonomic neuropathy can affect the cardiovascular, gastrointestinal and urogenital systems and sudomotor function. Cardiac involvement is associated with overall mortality while gastrointestinal involvement is a recognized cause of gastroparesis [64]. Historically, autonomic gastroparesis has been associated with poor pregnancy outcomes [65]. Gastroparesis causes erratic absorption of meals, resulting in episodes of hyperglycaemia and hypoglycaemia due to a mismatch between food intake and the short‐acting pre‐meal insulin. Although the suggestion that autonomic neuropathy causes hypoglycaemia unawareness has recently been challenged [66], the intensity of autonomic symptoms in response to hypoglycaemia decreases with duration of diabetes and this reduces the threshold at which hypoglycaemic symptoms occur [67]. Achieving good glycaemic control in early pregnancy, at a time when nausea and vomiting is common in women with autonomic gastroparesis, is challenging and hypoglycaemia is frequent. Diabetes is associated with premature cardiovascular disease. Women with over 20 years’ duration of type 1 DM aged over 40 years are likely to have a degree of coronary artery calcification and coronary artery disease (CAD), independently of other risk factors. Older women with type 2 DM and GDM are also at increased risk of CAD. A previous history of CAD or its diagnosis in pregnancy is associated with significant maternal morbidity [68–70]. Hypoglycaemia affects up to 70% of pregnant women with pre‐existing diabetes and is associated with excess maternal mortality. Hypoglycaemic symptoms change in pregnancy, especially if autonomic neuropathy is present. The risk of hypoglycaemia is greatest in the first 20 weeks of pregnancy and immediately post partum. Women with type 1 DM may benefit from an insulin pump and or continuous glucose sensors. Diabetic ketoacidosis (DKA) is a rare but a serious complication in pregnancy, with an associated fetal mortality in excess of 10% [71]. It usually occurs in women with type 1 DM, and can be the first presentation of type 1 DM; rarely it can occur in women with type 2 DM and even GDM. In pregnancy, DKA can occur at normal blood glucose levels (euglycaemic DKA), and thus it should be excluded in any pregnant woman with pre‐gestational diabetes and persistent nausea and vomiting and considered in all cases of unexplained maternal acidosis. The risk of DKA is increased during pregnancy because of increased insulin demands and enhanced lipolysis. Other risk factors include infection, vomiting, use of beta‐mimetic agents and insulin pump failures. The treatment of DKA is the same as for non‐pregnant patients, with insulin administration, fluid replacement, correction of electrolyte imbalance and treatment of the underlying cause. Fetal monitoring and assessment should continue alongside maternal treatment. Women with type 1 DM are at increased risk of autoimmune diseases. All women should be screened prior to pregnancy for thyroid disease; if they are already being treated for thyroid disease, this should be repeated in early pregnancy. There should be a high level of suspicion to screen for other autoimmune diseases if clinically indicated. Women with type 2 DM are likely to have other metabolic risk factors, including obesity, hypertension, dyslipidaemia and insulin resistance as are those with a previous history of GDM. Obesity, independently of diabetes, is a risk factor for hypertension, late stillbirth, induction of labour, caesarean section, birth trauma and maternal complications post partum [72,73]. Advising obese and overweight women on weight management prior to pregnancy is an important part of pre‐conception counselling. The hypertriglyceridaemia associated with obesity is a source of glycerol and fatty acids, both fetal metabolic substrates that in excess can contribute to accelerated fetal growth, and therefore maternal weight management prior to pregnancy will help. Maternal glucose crosses the placental barrier but insulin does not, so increases in maternal glucose stimulate fetal insulin production and hyperplasia of insulin‐sensitive tissues [74,75]. Maternal hyperglycaemia also enhances production of human placental growth hormone, fetal insulin‐like growth factor and tumour necrosis factor. As with insulin, these hormones act as fetal growth factors, resulting in accelerated fetal growth, macrosomia and organomegaly. The commonest malformations secondary to maternal hyperglycaemia are cardiac and those involving the central nervous system [1]. Cardiac malformations include transposition of the great vessels, ventricular septal defect and dextrocardia, while the central nervous system anomalies include anencephaly, spina bifida, hydrocephaly and holoprosencephaly; malformations of the genitourinary system and the skeleton also occur [76]. Caudal regression, a rare malformation, has the strongest association with diabetes, occurring roughly 200 times more frequently in infants of diabetic mothers than in other infants [76]. Both liquor and fetal cord blood insulin or C‐peptide at birth correlate with the risk of neonatal hypoglycaemia, respiratory distress, polycythaemia, hypocalcaemia and hyperbilirubinaemia, emphasizing the impact of maternal hyperglycaemia and secondary fetal hyperinsulinaemia on neonatal well‐being. The long‐term effects of a diabetic pregnancy on the child are only now being evaluated. Pregnancy outcomes are improved when women with diabetes attend a hospital‐based multidisciplinary consultant‐led diabetic obstetric antenatal clinic working to locally agreed guidelines based on national and international guidelines (Table 9.5). The team comprises an obstetrician, diabetologist, specialist midwife, diabetes specialist nurse and dietitian and all are jointly involved in the care. Rapid access to this clinic is critical to ensure optimization of glycaemic control during the period of fetal organogenesis. Women need to be seen or phoned by the diabetes specialist nurse or midwife on a weekly basis throughout the first trimester, with access to the other members of the team as required. Table 9.5 A structured approach to the management of diabetic pregnancies. Ideally, a dating scan should be performed within the first 10 weeks of gestation to confirm viability of the pregnancy and to accurately assess gestational age. Relying on ultrasound scanning later in a diabetic pregnancy is less accurate because fetal growth restriction or growth promotion may be present. Chromosomal abnormalities are not increased in women with diabetes. Routine antenatal blood tests plus nuchal translucency ultrasound scan for Down’s syndrome between 11 and 14 weeks of pregnancy should be offered as part of routine antenatal care. Calculating the risk of Down’s syndrome based on the standard formula of gestational age, mother’s age and levels of unconjugated estriol, α‐fetoprotein (AFP) and human chorionic gonadotrophin (hCG) is less accurate, as plasma levels of both AFP and unconjugated estriol are lower in diabetic pregnancies.
Diabetes in Pregnancy
Classification of diabetes in pregnancy
Type 1 diabetes
Absolute insulin deficiency due to autoimmune destruction of the pancreatic β‐cell. Presents typically under the age of 20 years old, and only 10% have a first‐degree relative affected. Not associated with obesity. Accounts for approximately 5% of all diabetes outside pregnancy
Type 2 diabetes
Relative insulin deficiency and decreased insulin sensitivity. Presents typically over the age of 20 years, and >50% have a first‐degree relative affected. Strongly associated with obesity. Accounts for approximately 90% of all diabetes outside pregnancy
Monogenetic diabetes
Maturity‐onset diabetes of the young (MODY). Results from a single gene mutation causing defects in pancreatic β‐cell insulin secretion. Present from birth and typically diagnosed within the second and third decade. Autosomal dominant with approximately 95% having a first‐degree relative affected. Not associated with obesity. Accounts for 1–2% of all diabetes outside pregnancy
Mitochondrial diabetes
Arises from a mutation in mitochondrial DNA leading to a defect in insulin secretion. Associated with a number of other medical problems including neural sensory deafness, a tendency for stroke and lactic acidosis. Diabetes develops at approximately 35 years old, and is maternally inherited. Not associated with obesity. Accounts for less than 1% of all diabetes outside pregnancy
Secondary diabetes
Diabetes due to other medical conditions, i.e. pancreatitis, cystic fibrosis, glucocorticoids and other drugs. Accounts for approximately 2% of all diabetes outside pregnancy
Diagnosing diabetes and gestational diabetes
IADPSG criteria
NICE 2015 criteria
Screening
None
Risk factors at first antenatal clinic
BMI >30 kg/m2
GDM in a previous pregnancy
Previous macrosomic baby ≥4.5 kg
Family history (first‐degree relative with diabetes)
Ethnicity: family origin with a high prevalence of diabetes
75‐g OGTT
Universal at 24–28 weeks
Selective testing 26–28 weeks
Based on the presence of risk factors
Plasma glucose
IADPSG criteria: one or more of the following thresholds be met or exceeded:
NICE 2015 criteria: one or more of the following thresholds be met or exceeded:
0 min
5.1 mmol/L (92 mg/dL)
5.6 mmol/L (100 mg/dL)
60 min
10 mmol/L (180 mg/dL)
120 min
8.5 mmol/L (153 mg/dL)
7.8 mmol/L (140 mg/dL)
The significance of maternal type of diabetes
The principles of maternal glycaemic control
First trimester
Implantation
Inhibits trophectoderm differentiation
Embryogenesis
Increases oxidative stress, affecting expression of critical genes essential for embryogenesis
Organogenesis
Activates the DAG–PKC cascade, increasing congenital defects
Miscarriage
Increases premature programmed cell death of key progenitor cells of the blastocyst
Second trimester
Endocrine pancreas
Stimulates fetal β‐cells
Fetal growth
Stimulates fetal hyperinsulinaemia, which results in growth acceleration seen on ultrasound by 26 weeks
Third trimester
Fetal growth
Major fetal substrate and determinant of accelerated fetal growth
Adipose disposition
Stimulates hyperinsulinaemia, which promotes fat deposition including intra‐abdominal fat
Lung maturation
Stimulates hyperinsulinaemia, which delays lung maturation by inhibiting surfactant proteins
Stillbirth
Is associated with defects in placental maturation that increase the risk of fetal hypoxia
Delivery
Birth trauma
By causing accelerated fetal growth, there is an increased risk of shoulder dystocia, predisposing to birth trauma and asphyxia
Neonate
Hypoglycaemia
Stimulates fetal hyperinsulinaemia, which predisposes to neonatal hypoglycaemia
Hypocalcaemia
Alters the placental expression of calbindin mRNA, which affects calcium status at birth
Polycythaemia
Stimulates fetal hyperinsulinaemia, which enhances antepartum haemopoiesis as does fetal hypoxia
Cardiomyopathy
Stimulates fetal hyperinsulinaemia, which predisposes to hypertrophic cardiomyopathy
Adolescence/adulthood
Obesity
Intrauterine exposure predisposes to the metabolic syndrome, independent of any genetic susceptibility
Type 2 diabetes
Intrauterine exposure predisposes to type 2 diabetes, independent of any genetic susceptibility
Pre‐conception counselling
Optimizing glycaemia control
Reviewing all medications
Screening and management of diabetic complications
Information on optimal weight and weight gain
Information on pregnancy risk to the mother
Information on pregnancy risk to the baby
Achieving optimal glycaemic control
Screening for diabetic complications
Diabetic retinopathy
Diabetic nephropathy
Diabetic neuropathy
Diabetic macrovascular disease
Hypoglycaemia
Diabetic ketoacidosis
Screening for non‐diabetic comorbidities
Risks to the fetus of a diabetic pregnancy
Managing diabetes in pregnancy
First trimester
Referral to a combined multidisciplinary diabetic obstetric antenatal clinic
Prior to pregnancy
Pre‐conception counselling
Review of all medications
Folic acid 5 mg once daily
Screening for diabetic complications, including treatment of diabetic retinopathy
First trimester
Referral to a combined multidisciplinary diabetic obstetric antenatal clinic
Dating scan at 6–8 weeks, confirming viability and gestational age
Screening for diabetic complications
Screening for non‐diabetic comorbidities
Assessment and optimization of glycaemia
Advice on hypoglycaemia prevention
Second trimester
Optimization of glycaemic control
Screening for diabetic complications
Repeat digital retinal assessment at 16 weeks if initial retinal screen was abnormal
Surveillance for medical obstetric complications, including hypertension
Screening for congenital abnormalities, including ultrasonographic examination of the fetal heart at 18–22 weeks
Assessment of fetal growth
Third trimester
Optimization of glycaemic control
Screening for diabetic complications, with repeat digital retinal screening
Assessment of fetal growth and amniotic fluid volume at 28, 32 and 36 weeks’ gestation
Timing and mode of delivery to be discussed at 36 weeks’ gestation
Document any changes to hypoglycaemic therapy during delivery and post partum, including breastfeeding
Delivery
Protocols for insulin during labour and delivery
Post partum
Adjustment of insulin dosage
Breastfeeding
Discuss contraception
Dating ultrasound scan
Screening for chromosomal anomalies
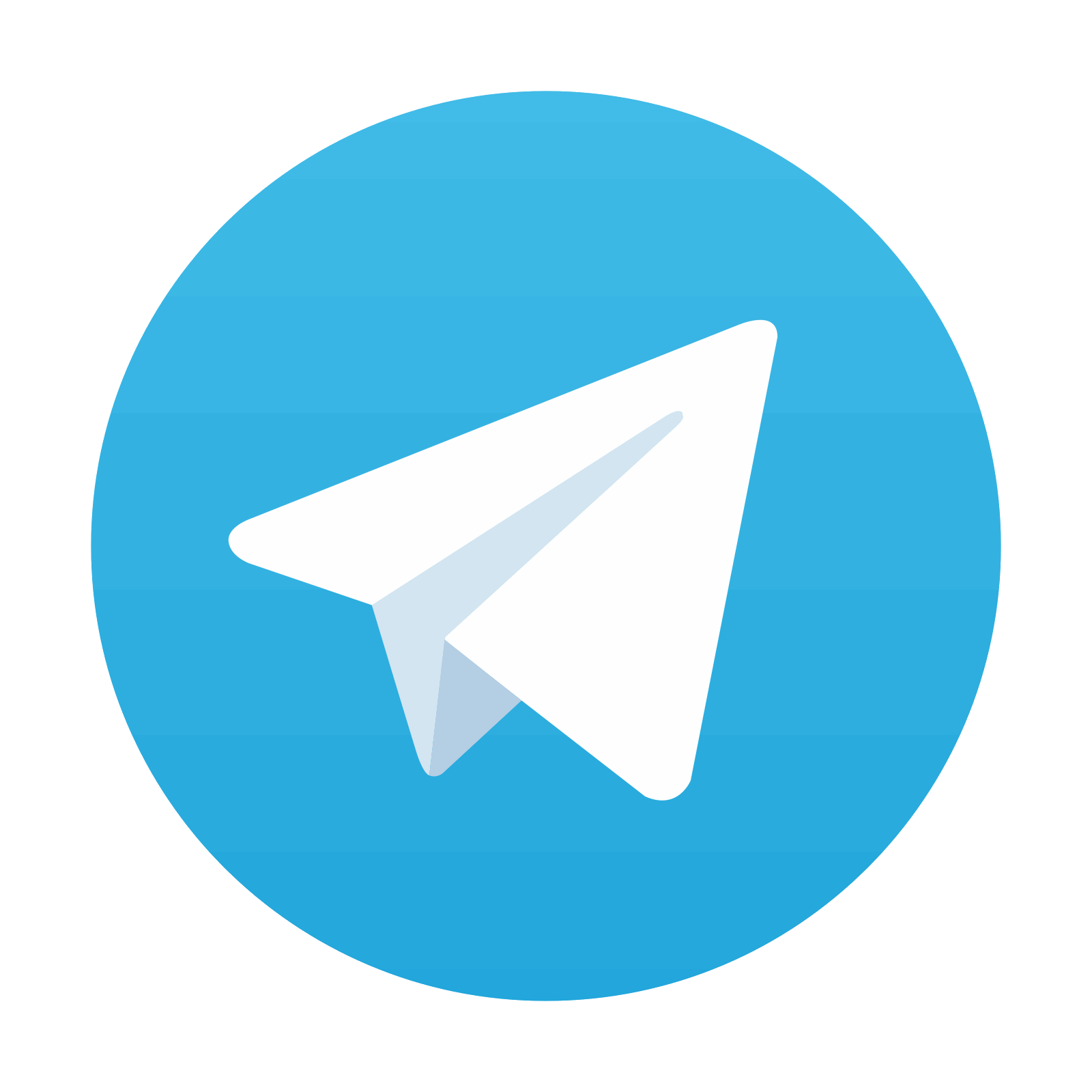
Stay updated, free articles. Join our Telegram channel

Full access? Get Clinical Tree
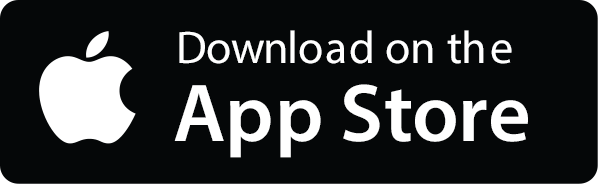
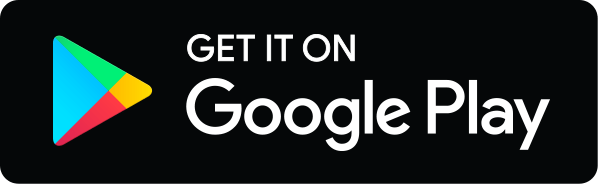