Patient age
Total body watera
Extracellular fluida
Intracellular fluida
<3-Month fetus
92
65
25
Term gestation
75
35–44
33
4–6 months
60
23
37
12 months
26–30
Puberty
~60
20
40
Adult
50–60
20
40
Understanding the body distribution characteristics for a drug is important when prescribing a drug and calculating the dose to be administered. For example, if target drug receptors are located within the central nervous system (CNS) the drug must distribute into the CNS for therapeutic effect. Although the absolute value of a drug’s V d does not correlate with any real physiologic volume (i.e., hence the formal name for V d is apparent volume of distribution), knowledge of this PK parameter provides insight into the total amount of drug present in the body relative to its concentration in blood and, thus, tissue distribution. Clinically it can be speculated with a moderate degree of certainty that a drug with a very large V d (e.g., 10 L/kg) can be assumed to distribute widely throughout the body (possibly even into the CNS, e.g., anesthetics), whereas a drug with a very low V d value (e.g., 0.1 L/kg) might be expected to have limited body distribution. However, regardless of a drug’s V d numeric value, what really matters is the drug effect. Independent of a drug’s V d, what matters clinically is that a sufficient amount of drug distributes to and binds to the necessary receptor site to stimulate the desired pharmacologic effect(s).
Identification of the age-appropriate V d for a drug can be obtained from most computer information/pediatric drug dosing references. The reported V d value is unique to each individual drug and will change relative to age and possibly even by disease, particularly for those diseases that result in large volume shifts. A comparison of V d values between neonates and adults for a few select drugs is shown in Table 2.2.
Table 2.2
Comparison of volume of distribution and elimination half-life for selected drugs in neonates compared to adults
Example drug (brand name) | Neonate | Adult | ||
---|---|---|---|---|
V d | t1/2 | V d | t1/2 | |
Amikacin (Amikin®) | 0.6 | 8.4 | 0.3 | 2.3 |
Amoxicillin (Amoxil®) | 0.7 | 3 | 0.2 | 1.7 |
Bumetanide (Bumex®) | 0.22 | 6.5 | 0.13 | 1.5 |
Caffeine citrate (Cafcit®) | 0.85 | 84 | 0.6 | 5 |
Caspofungin (Cancidas®) | 0.43 | 8.3 | 0.25 | 13 |
Cefepime (Maxipime®) | 0.43 | 5.0 | 0.26 | 2.1 |
Gentamicin (Garamycin®) | 0.7 | 7.2 | 0.31 | 2.5 |
Levetiracetan (Keppra®) | 0.89 | 9 | 0.6 | 6 |
Morphine | 2.3 | 7.0 | 3.0 | 2 |
Pantoprazole (Protonix®) | – | 3.1 | – | 1–1.5 |
Phenobarbital | 0.71 | 108 | 0.54 | 60–80 |
Tobramycin (Nebcin®) | 0.7 | 8.3 | 0.33 | 2.2 |
Vancomycin (Vancocin®) | 0.57 | 6–10 | 0.39 | 6 |
Drug Distribution: Protein Binding
In addition to body fluid compartments, the amount (%) of drug binding to protein will influence the amount of drug distributed within the body. Drug bound to proteins (or other fractions) is not pharmacologically active or available for metabolism or excretion—it is only the free (unbound) fraction of the absorbed drug that is pharmacologically (also toxicologically) active, capable of diffusing outside the circulatory compartment/across cell membranes distributing to the site(s) of action, and available for body elimination, e.g., liver metabolism and/or renal excretion. Important differences exist between the degree of drug–protein binding in premature and newborn infants compared to adults and examples are outlined in Table 2.3. The concentration of plasma proteins is reduced in the immediate post-delivery period as well as there are select, endogenous circulating compounds found in a neonates circulation that may/will compete for plasma/albumin binding. The most important of these compounds is bilirubin for which controversy persists surrounding possible displacement of bilirubin from its albumin binding sites by drugs and possibly precipitating kernicterus. Although such an albumin–drug–bilirubin displacement interaction is possible, the magnitude of such an interaction depends on a number of variables but most notably, the absolute concentration of drug(s) and bilirubin relative to the circulating albumin concentration, number of available albumin binding sites, and the presence of other albumin binding compounds that will compete for the same albumin binding sites. Fortunately, this combination of required events rarely occurs in clinical medicine. In fact more recent animal data suggests that the immaturity of the efflux pump, P-glycoprotein, in the blood–brain barrier may be the most important determinant of bilirubin-brain concentrations rather than a simple drug–protein displacement interaction [8]. Further complicating this controversy is the fact that our knowledge of the extent to which a drug is usually bound to albumin, e.g., 50 and 90 %, provides little to no dependable clinically relevant information as to the extent a drug might displace bilirubin from its albumin binding sites. For example, two drugs frequently used in the care of premature and newborn infants furosemide (Lasix®) and midazolam (Versed®) are both highly bound to plasma albumin [14] and unassociated with any drug–bilirubin–albumin displacement reaction.
Table 2.3
Percent (%) protein binding of select representative drugs in newborn infants compared to that in adults
Drug | Percent bound | |
---|---|---|
Newborn | Adult | |
Ampicillin | 10 | 18 |
Diazepam (Valium®) | 84 | 99 |
Digoxin (Lanoxin®) | 20 | 32 |
Ibuprofen (Caldolor®) | 95 | 99 |
Micofungin (Mycamine®) | 96.7 | 99.6 |
Morphine | 35 | 45 |
Nafcillin (Unipen®) | 69 | 89 |
Phenytoin (Dilantin®) | 80 | 90 |
Phenobarbital | 32 | 47 |
Theophylline | 36 | 56 |
Clinical relevance: Knowledge of the age-appropriate V d in liters per kg body weight (V d L/kg) for a given drug allows the clinician a simple yet accurate method to calculate the peak drug concentration achieved with the first dose of drug. The peak concentration obtained after the first dose can be calculated using the following relationship: peak drug concentration = drug dose (μg or mg) divided by drug V d (l/kg) multiplied by the patient’s body weight in kg. For example, if you order a 5 mg dose of a drug to be administered to an 800 g infant and the drugs V d is 0.4 L/kg, the estimated peak concentration right after the full dose is administered would be peak = (5,000 μg dose) divided by (320—infant body weight (0.8 kg) multiplied by the drug V d in milliliters—400 mL); thus it would be ~15.6 μg/mL. Conversely, if you want to determine the dose of a drug to achieve a specific target blood concentration the equation can be rearranged to: dose (μg or mg) = (desired blood level in μg/mL) multiplied by (the drug V d in mL/kg) (patient body weight (kg). The most common errors observed in these simple mathematical calculations are those related to converting units properly, i.e., mg to μg and kg to g. Note: the reason this simple calculation is only valid after the first dose is these equations assume no drug is present in the body at the time of the first dose. After multiple doses the amount of drug must be inserted into the calculation (subtracted from the peak concentration) to accurately determine a drug’s V d.
With respect to drug binding to plasma proteins the extent of binding for a specific drug is most often of limited to no clinical significance. The defined clinically used dosing strategy accounts for the amount of drug bound and the amount of free drug. However for certain drugs, the “target” serum drug concentration differs from that defined for infants as compared to older infants, children, or adults. This important clinical discrepancy is merely due to the reduced drug–albumin binding for various drugs in the neonate vs. older infants, children, and adults (see Table 2.3). The importance of this factor is that defined, target “therapeutic serum/plasma drug concentrations” determined in adults may be totally appropriate for older infants and children but may be different in the neonate underscoring the importance of drug concentration definitions for different post-conceptional ages (PCA). Table 2.3 provides some estimates in the percent of protein binding for select drugs used in the NICU.
Drug Metabolism
Most drugs are not excreted from the body unchanged, but rather undergo biochemical modification usually by specialized enzymatic systems in a process known as xenobiotic metabolism or biotransformation. These enzymes are found in most human tissues (e.g., lung, kidney) with the highest concentrations in the liver and small and large intestines. While the liver is considered the major source of drug metabolizing activity, the enzymes located within the epithelial cells of the small intestine initiate the biotransformation of most orally administered medications. As noted in absorption above, once drugs are intestinally absorbed they enter the portal circulation for the aforementioned “first-pass” through the liver. Any hepatically metabolized drug that “escapes” this initial pass through the liver eventually undergoes sufficient metabolism on subsequent passes through the portal circulation. One can avoid or minimize the impact of intestinal and/or hepatic first-pass drug metabolism by using the sublingual (e.g., nitroglycerine), nasal, and in some cases rectal routes for drug administration. Some drugs are not easily metabolized and, hence, remain in the body for longer periods of time whereas other drugs may not undergo any metabolism and will be eliminated from the body unchanged.
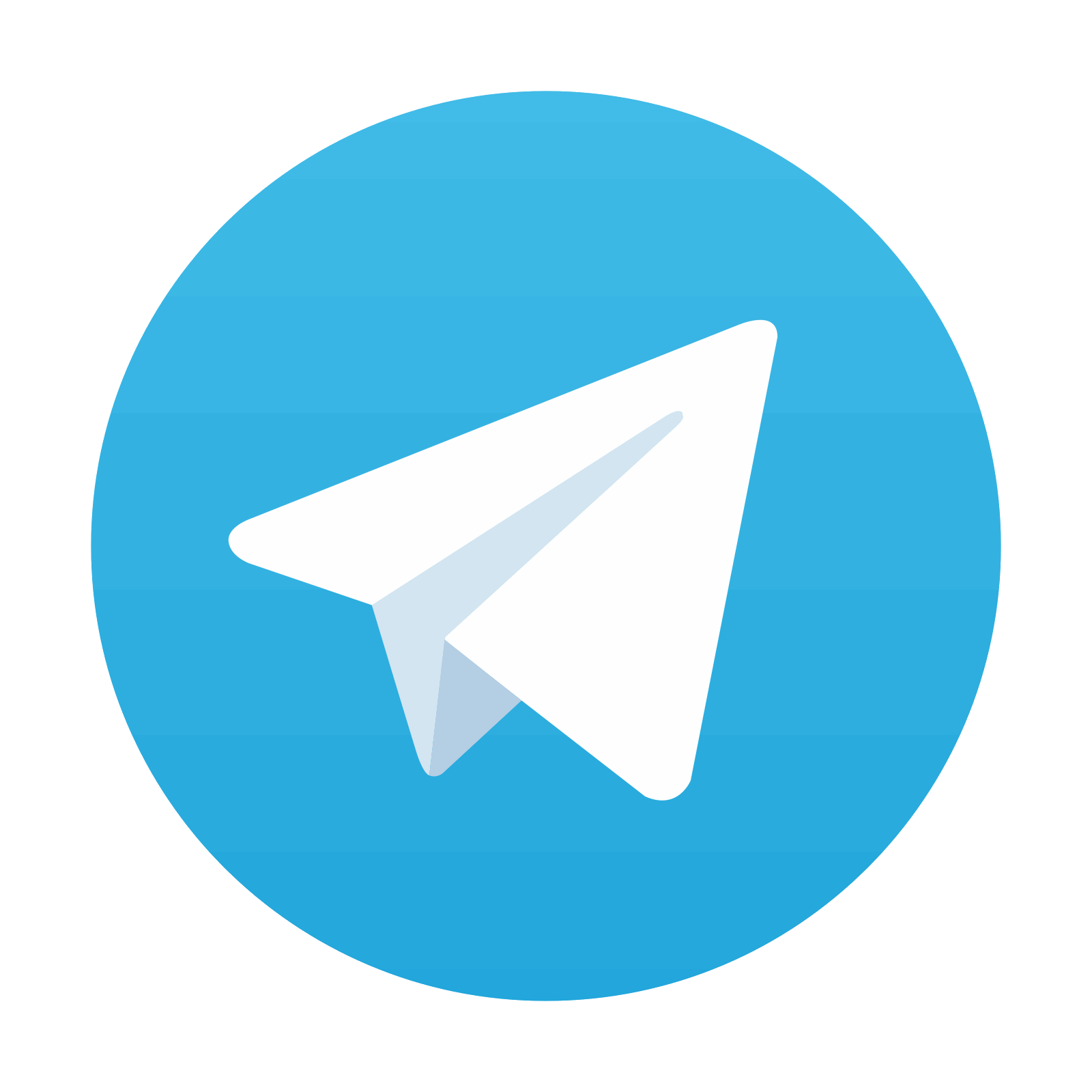
Stay updated, free articles. Join our Telegram channel

Full access? Get Clinical Tree
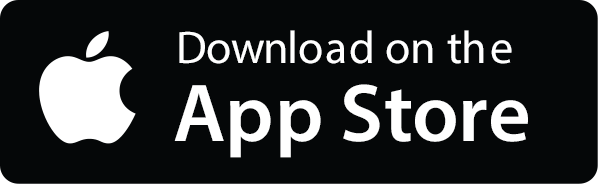
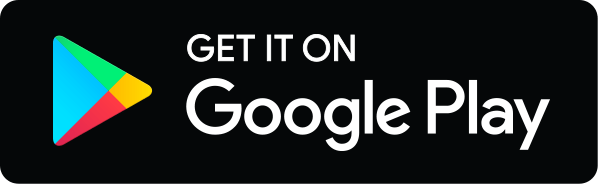