Fig. 5.1
Lymphatic vessel basics. Interstitial fluid and immune cells pass through discontinuous adherens junctions on lymphatic capillaries in a unidirectional manner. One of the main markers for lymphatic capillaries is LYVE-1. Precollecting vessels share features of both lymphatic capillaries (LYVE-1 expression) and collecting vessels (lymphatic valves). Lymphatic collecting vessels have valves that ensure unidirectional flow of lymph, continuous basement membrane and contractile smooth muscle cells
The diversity of lymphatic vessel types found in adults is a product of a complex interplay between lymphatic endothelial identity factors, growth factor-mediated signaling to lymphatic-specific receptors, and interaction with external factors, e.g. interstitial fluid pressure, and flow and shear stress. We summarize in this chapter our current understanding of the molecular mechanisms that produce the lymphatic vasculature.
5.3 Developmental Lymphangiogenesis
5.3.1 Lymphatic Network Formation
Florence Sabin first proposed the venous origin of lymphatic vessels [7], and recent studies, using lineage tracing and high-resolution imaging in thick sections and whole embryos, have confirmed this hypothesis [8–10]. Lymphatic endothelial cell (LEC) progenitors emerge from the cardinal veins and intersomitic vessels through a process of budding without disturbing the integrity of the venous wall. The streams of LECs, connected by continuous adherens junctions, migrate from the veins and form an initial lymphatic plexus. LECs then coalesce and form large lumenized lymphatic vessels, commonly called ‘lymph sacs’, one of which will become the thoracic duct [8, 10]. This process of lymphatic plexus formation, followed by lumen formation, continues in a caudal–dorsal manner to form an almost complete lymphatic network before birth (Fig. 5.2a, b, c, d).

Fig. 5.2
Molecular mechanisms controlling lymphatic cell identity, sprouting, and segregation from blood vessels. (a) ERK signaling activation delineates a subset of venous cells as progenitor lymphatic endothelial cells (LECs), which, in turn, express SOX18, COUP-TFII, and PROX1. (b) LECs migrate from the vein in response to vascular endothelial growth factor (VEGF)-C/VEGF receptor (VEGFR)-3 signaling. The VEGFR-3 co-receptor neuropilin-2 (NRP2) also promotes VEGF-C/VEGFR-3 signaling. CCBE-1 is also necessary for LEC migration from the cardinal vein. LEC mechanical stretching contributes to activation of VEGFR-3 signaling and LEC proliferation. (c) LECs migrate in streams away from the cardinal vein to form the lymphatic primary plexus and primordial thoracic duct. (d) Normal platelet aggregation is important for keeping lymphatic and blood vasculatures segregated. Platelets may act either by modulating formation or function of lymphovenous valves via unknown mechanisms or by preventing ectopic fusion of lymphatic and blood vessels
Although many questions remain regarding the process of lymphangiogenesis during embryonic and postnatal growth, the molecular mechanisms controlling many steps of LEC identity, differentiation, migration, proliferation, and vessel formation have been identified.
5.3.2 Establishment of Lymphatic Endothelial Cell Identity
The first gene described as a regulator of LEC identity was PROX1 [11], a homeobox transcription factor that is first expressed in a subset of cells in the cardinal vein around E9.5 (Fig. 5.2a). Nascent LECs, which bud off into the mesenchyme, also express lymphatic endothelial-specific markers, including VEGFR-3, podoplanin, CCL21, neuropilin-2 (NRP2), and LYVE-1, as well as a specific integrin repertoire, such as ITGα6β1 (Fig. 5.2b) [8, 12]. PROX1 is essential for lymphatic vascular development as Prox1-null mice fail to develop a lymphatic vascular network because of the failure of LECs to migrate from veins [10, 11]. Furthermore, PROX1 is necessary for lymphatic identity maintenance after the formation of the lymphatic network, as shown by both in vitro and in vivo experiments [13, 14].
The transcription factor SOX18 acts upstream of PROX1, and its expression in a subset of dorsolateral cardinal vein endothelial cells is necessary for the induction of PROX1 expression [15]. Mutations in SOX18 were described in patients with hypotrichosis-lymphedema-telangiectasia [16], which underscores the role of this transcription factor in lymphatic vascular development. Recent work suggests that localized activation of the MEK/ERK signaling cascade regulates polarized SOX18 expression and subsequent development of lymphatic vasculature [17]. Endothelial-specific activation of the MEK/ERK pathway, through an activating mutation in Raf1, caused increased commitment of venous endothelial cells to the lymphatic fate, which led to expansion of lymphatic vessels and decreased size of veins. Strikingly, activated ERK was able to induce PROX1 expression in arterial endothelial cells, suggesting a fundamental role of this signaling cascade in the establishment of the LEC identity program [17].
Another factor necessary for lymphatic cell identity maintenance is COUP-TFII. In blood vessels, COUP-TFII maintains venous identity by suppressing Notch signaling [18]. COUP-TFII is critical for the early stages of lymphatic identity establishment and maintenance in LEC progenitors during migration away from the vein by promoting expression of PROX1, VEGFR-3, NRP2 and LYVE-1 [9, 19, 20]. However, COUP-TFII is not necessary to maintain PROX1 after initial LEC identity establishment as PROX1 expression is unchanged after inducible deletion of COUP-TFII at E13.5 [19]. The importance of COUP-TFII seems to be limited to growing lymphatics as COUP-TFII deletion in adults resulted in no detectable phenotype [19]. Nevertheless, COUP-TFII deletion suppressed tumor lymphangiogenesis and in vitro LEC sprouting, and these effects seem to be mediated by a direct effect of COUP-TFII on NRP2 expression, a modulator of VEGFR-3 receptor tyrosine kinase (RTK) activity [19, 21].
Notch signaling plays a major role in arteriovenous differentiation (reviewed by Swift and Weinstein [22]), and was also recently shown to play a role in lymphatic endothelial differentiation [23]. Lymphatic-specific Notch1 deletion from E9.75 to E13.5 resulted in an increase of PROX1-positive cells, enlarged lymph sacs and dermal lymphatics, and LEC migration into veins [23]. Consistent with Notch as a negative regulator of LEC identity, LEC-specific Notch activation, by inducible expression of the Notch1 intracellular domain, during the same timeframe, caused an opposite phenotype: decreased expression of PROX1, podoplanin, VEGFR-2, VEGFR-3, LYVE-1, and hypoplastic, abnormal, blood-filled lymphatics near the cardinal vein [23]. Furthermore, LEC-specific Notch1 activation also decreased COUP-TFII expression, suggesting that Notch repression is necessary for COUP-TFII-mediated PROX1 activation. However, the effect of Notch signaling may not be so simple as endothelial cell-specific deletion of RBPJ, the main mediator of Notch target gene expression, could not rescue PROX1 expression in embryos with ablated COUP-TFII [20]. Whatever the exact mechanism, Notch plays an important role in constraining lymphatic cell differentiation during embryogenesis.
5.3.3 Lymphangiogenic Sprouting and Migration
Once LECs have differentiated in cardinal veins they migrate and proliferate to form the lymphatic vascular network (Fig. 5.2c). Vascular endothelial growth factors (VEGFs) are the primary stimuli driving developmental angiogenesis and lymphangiogenesis by binding VEGF receptors, which in turn activate intracellular signaling cascades promoting migration, proliferation, and survival (reviewed by Jeltsch et al. [24]). VEGF-A and VEGF-C are the main angiogenic and lymphangiogenic growth factors, respectively, and both are ligands for VEGFR-2 and VEGFR-3 (reviewed by Adams and Alitalo [25]). LECs express high levels of VEGFR-3 [26]. In addition, sprouting blood vessels, as well as some tumor vessels and fenestrated endothelial cells, also express low levels of VEGFR-3 [27–29]. VEGF-C expression is first detected at E12.5 near the cardinal vein where the first LECs are differentiating, corresponding with high levels of LEC VEGFR-3 expression [30]. VEGF-C-null embryos, which die before birth, have severe edema due to the lack of lymphatic vessels. Indeed, in the absence of VEGF-C, PROX1 is expressed in LEC progenitor cells; however, these cells do not migrate from veins to form ‘lymphatic sacs’, suggesting VEGF-C is one of the main stimuli promoting LEC migration [8, 31]. The importance of VEGF-C is highlighted by the fact that even VEGF-C +/− mice exhibit chylous ascites and defects in postnatal lymphangiogenesis with hypoplastic dermal lymphatics [31]. Furthermore, VEGF-C overexpression in skin results in hyperplastic and proliferating lymphatic vessels [32]. Mutations in VEGFR-3 cause congenital lymphedema, and similar mutations in mice lead to severe lymphatic vascular hypoplasia [33]. In addition, expression of soluble VEGFR-3, which prevents VEGF-C-mediated activation of VEGFR-3, in the skin, caused complete regression of the dermal lymphatic network in mouse pups without affecting blood vessels [34]. Thus, the VEGF-C/VEGFR-3 signaling axis is likely the most important mechanism regulating lymphatic endothelial migration and survival.
5.3.4 Extracellular Signaling Pathways in Lymphangiogenesis
One accessory protein that promotes VEGFR-3 signaling in LECs is NRP2. NRP1 and 2 were first described as axon guidance factors belonging to the class-3 semaphorin subfamily, but were later shown to play an important role in the vasculature. NRP1 is a co-receptor of VEGFR-2 and is essential for embryonic angiogenesis and vascular maturation (reviewed by Koch and Claesson-Welsh [35]). NRP2 binds VEGF-C and VEGFR-3 and, after ligand binding, both NRP2 and VEGFR-3 are internalized in LECs [36]. NRP2 is highly expressed in actively growing lymphatic vessels, where it controls VEGF-C/VEGFR-3-dependent lymphatic vessel sprouting [21, 37, 38].
Another factor associated with VEGF receptors is claudin-like protein 24 (CLP24), a member of the claudin protein family of intercellular junction proteins [39]. Frogs or fish with CLP24 ablation failed to develop a lymphatic network, whereas CLP24-deficient mice display a milder phenotype with dilated lymphatic capillaries and ectopic smooth muscle cell coverage [40]. CLP24 associates with VEGFR-2 and VEGFR-3 and restricts activation of Ca2+/cAMP/CREB, while other downstream signaling pathways, such as p38 mitogen-activated protein kinase (MAPK), are not affected [40].
Collagen- and calcium-binding EGF domains 1 (CCBE1) protein is an extracellular matrix (ECM) component critical for lymphangiogenesis in zebrafish, mice, and humans [41–44]. Hennekam syndrome is characterized by general lymphatic dysplasia with patients having limb lymphedema and lymphangiectasia of the lung, intestine, pericardium, thyroid, and kidney [41]. Some Hennekam syndrome patients have inherited point mutations in the calcium-binding EGF domain of CCBE1 [41]. CCBE1-deficient mouse embryos have severe edema and die before birth because of a complete lack of lymphatic vessels [42]. Similar to the phenotypes of VEGF-C– and PROX1-deficient mice, the PROX1-positive LEC precursors cells are unable to bud from the cardinal vein, indicating that LEC migration cues are disturbed in these mice [42].
An elegant study recently shed light on how mechanosensory input influences developmental lymphangiogenesis [45]. LEC stretching was found to increase VEGF-C- and integrin-β1-dependent VEGFR-3 phosphorylation and LEC proliferation [45]. Therefore, interstitial fluid pressure, which increases at the onset of lymphatic vasculature expansion at E10.5–E12.5 and causes stretching of LECs, is one of the important mechanical factors that contributes and cooperates with tissue gradients of VEGF-C to induce growth of lymphatic vessels [45].
The angiopoietins (Ang1 and Ang2) are ligands for Tie receptors (Tie1 and Tie2), another class of RTKs. Ang1 and Ang2 are important in blood vessel maturation and sprouting, respectively (for review, see Augustin et al. [46]). Ang2 −/− mice have chylous ascites, hypoplastic lymphatic capillaries, and ectopic smooth muscle cell coverage of lymphatic capillaries, suggesting that Ang2 plays an important role during developmental angiogenesis [47]. However, the lymphatic phenotype observed in Ang2 −/− mice could be rescued by inserting Ang1 into the Ang2 locus, suggesting that the differential roles of Ang1 and Ang2 in lymphangiogenesis need to be resolved [46]. Tie1 is necessary for functional lymphatic vessels. Hypomorphic mutation or induced deletion of Tie1 results in embryos with edema. This edema is likely caused by a loss of lymphatic vessel caliber regulation, suggesting a role for Tie1 in modulating pro-lymphangiogenic signaling [48, 49].
The Eph receptors and their ligands, ephrins, are a class of RTK. Ephrin ligands bind and stimulate phosphorylation of the cytoplasmic end of Eph receptors in ‘forward’ signaling. Eph/ephrin interactions can also result in ‘reverse’ signaling whereby the Eph receptors act as ligands and stimulate cytoplasmic phosphorylation of ephrin ligands (reviewed by Kullander and Klein [50]). EphB4 and ephrinB2 are expressed in lymphatic vessels [51]. The cytoplasmic domain of ephrinB2 is important for lymphangiogenic sprouting as sprouting defects were observed in the postnatal dermis in mutant animals [51]. The mechanism of reduced sprouting in eprhinB2 mutants may be through inhibition of VEGFR-3 internalization after VEGF-C binding [52]. Therefore, EphB4/ephrinB2 signaling plays an important part in mediating the effects of the VEGF-C/VEGFR-3 signaling axis.
Notch signaling plays a fundamental role in the regulation of sprouting angiogenesis. The current view is that angiogenic tip cells induce canonical Notch signaling in stalk cells, which represses VEGF receptor signaling and thereby reduces sprouting potential [53, 54]. Such maintenance of differential sprouting potential is essential for efficient angiogenesis [55]. The role of Notch signaling in lymphatic vascular sprouting and remodeling has recently been investigated. Systematic analysis of Notch signaling components in zebrafish revealed that Dll4 and Notch1b are necessary for thoracic duct formation and LEC sprouting and migration [56]. In mice, the role of Notch signaling in sprouting and migration is less well-defined. A study by Zheng et al., using Dll4-Fc, showed that Notch blockade increased LEC spheroid sprouting in vitro, especially in response to VEGF-A [57]. Co-injection of Dll4-Fc and VEGF-A-expressing adenovirus caused an increase in dermal lymphatic vessels in adult mice compared with VEGF-A alone, suggesting that Notch signaling in lymphatic vessels restricts VEGFR-2-dependent signaling in LECs [57]. In contrast, a study by Niessen et al. found that postnatal Notch inhibition, using Notch1 and Dll4 blocking antibodies, decreased lymphatic vessel density and VEGFR-3 surface staining in dermal lymphatic vessels [58]. Therefore, the role of Notch signaling in lymphatic sprouting, and possible differences between adult and postnatal lymphangiogenesis, remains an open question.
ALK1 and ALK5 are endothelial type I receptors of the transforming growth factor (TGF)-β superfamily of ligands [59]. ALK1-Fc treatment in pups induced chylous ascites as well as a massive increase in retinal blood vessel density [60]. Dermal and intestinal lymphatic capillaries also fail to fully develop after ALK1 blocking; however, collecting vessels are unaffected [60]. Interestingly, genetic inactivation of one of the high-affinity ALK1 ligands, BMP9, has no effect on lymphatic capillary sprouting, while the formation of collecting vessels is compromised ([61]; see below). This suggests that other ALK1 ligands or ligand-independent signaling should be considered for explaining the effects of ALK1-Fc. Endothelial- or LEC-specific inactivation of TGFβr1, or its co-receptor TGFβr2, during embryonic development prevents LEC sprouting and leads to enlarged, hyperproliferative dermal lymphatic vessels [62]. Thus, signaling via ALK1, TGFβr1, and TGFβr2 is important to ensure the balance of sprouting versus proliferating LECs during lymphangiogenesis, and is necessary for the correct patterning of the vascular network [60, 62].
5.3.5 Intracellular Signaling Pathways in Lymphangiogenesis
Survival and proliferation of LECs in vitro is mediated by the PI3K/AKT and MEK/ERK signaling pathways [63]. Indirect evidence suggesting a role for the PI3K/AKT pathway was the observation that mice with germline deletions of the regulatory subunits of PI3K develop chylous ascites [64]. Intestinal submucosal and diaphragm lymphatic capillary density was decreased in the mice with germline deletion of PI3K regulatory subunits, suggesting that PI3K plays a role in LEC survival postnatally [65]. Furthermore, PI3K catalytic subunit interaction with Ras is necessary for LEC survival as mice with point mutations disturbing this interaction have chylous ascites and defects in lymphatic vasculature development [66].
AKT-deficient mice provided further evidence for the role of PI3K signaling in lymphangiogenesis. There are three isoforms of AKT (AKT1, 2, and 3), and AKT1 is the predominant form in BECs [67]. Dermal lymphatic capillaries in AKT1, but not AKT2 or AKT3, germline knockout mice were hypoplastic due to a decreased number of LECs [68]. In addition, AKT1 was also important for the formation of precollector valves; however, valves in larger collecting vessels were unaffected. Nevertheless, AKT1 inactivation did not affect VEGF-C-induced LEC sprouting in vivo, suggesting that it may be more important for cell survival [68]. Interestingly, the development of blood vessels is normal in the absence of AKT1 [69], which suggests differential usage of this signaling pathway between blood and lymphatic vessels.
A modulator of the Ras/MEK/ERK pathway, RASA1, was also found to be involved in lymphatic function. RASA1-null mice have numerous blood vessel defects, including decreases in branching and stability [70]. Constitutive or lymphatic-specific deletion of RASA1-induced chylothorax and chylous ascites in both weaning pups and adults, presumably due to loss of VE-cadherin/LYVE1 button-like junctions [71]. Lymphatic-specific deletion of RASA1 also caused increased LEC proliferation, coincident with an increase in Ras/ERK signaling in RASA1-deficient lymphatic vessels in vivo. Aberrant ERK signaling is activated via the VEGF-C/VEGFR-3 pathway, as RASA1-deficient mice treated with VEGFR-3 blocking antibodies did not have a lymphatic phenotype. Therefore, RASA1 provides a link between pro-lymphangiogenic growth factors and mechanisms of VEGFR-3-mediated transduction of these signals through Ras [71].
RAC1, a small GTPase in the Rho family, has been shown to play a role in endothelial cell migration in vitro [72]. Surprisingly, endothelial-specific deletion of RAC1 in vivo is not sufficient to impair sprouting angiogenesis, but is required for the migration of committed LECs from the cardinal vein [73]. RAC1 inactivation reduces LEC migration in response to VEGF-C, which ultimately leads to close association of early lymphatic vessels (‘lymph sacs’) and the cardinal vein. RAC1-deficient embryos also display blood-filled lymphatic vessels [73]; however, complete interpretation of this phenotype needs to be evaluated in the context of the roles of lymphovenous valve status and/or platelet function in lymphatic-venous separation (see below).
5.3.6 Platelets and Lymphatics: More Complex than Initially Thought
The mature lymphatic vasculature is connected to blood circulation at only few specific regions, such as the junction between the thoracic duct and subclavian vein. The reflux of blood to the thoracic duct is prevented by a lymphovenous valve that forms early during embryonic development [74]. A number of genetic model phenotypes include blood-filled lymphatic vessels, indicative of additional patent connections between the blood and lymphatic vasculatures. Importantly, genes deleted in models that display the blood-filled lymphatic phenotype encode components of signaling pathways regulating platelet development or aggregation, such as MEIS1 [75], podoplanin (or the enzyme important for its post-translational modification) [76, 77], PLCγ2 [78], CLEC-2, SLP76, and Syk [79, 80]. Podoplanin, a cell surface glycoprotein, is highly expressed in LECs. Binding of podoplanin to CLEC-2 on platelets induces activation of the Syk/SLP76/PLCγ2 signaling cascade and platelet aggregation (reviewed by D’Amico and Alitalo [81]). Until recently, it was thought that platelet thrombi, induced by podoplanin on the surface of the nascent lumenized lymphatic vessel, ‘seal off’ new lymphatic vasculature from the cardinal vein [77, 80], in a mechanism somewhat analogous to closure of the ductus arteriosus [82]. However, more recent evidence shows that LECs migrate from veins as nonlumenized streams of cells, without disturbing venous wall integrity [8, 10]. Therefore, the role of platelet aggregation in the formation of abnormal connections between blood and lymphatic vessels is probably more complex, and may, at least in part, include malformations or dysfunction of the lymphovenous valve and cytokine-induced fusion of the lymphatic and blood vasculatures (Fig. 5.2d) [83].
5.4 Lymphatic Vessel Maturation
5.4.1 Maturation of Lymphatic Capillaries
Fully mature lymphatic capillaries contain LECs with discontinuous highly specialized ‘button-like’ junctions, allowing free passage of interstitial components and immune cells into the vessel lumen while maintaining vessel integrity [5]. In contrast, LECs in actively sprouting lymphatic vessels are connected by continuous adherens junctions (Fig. 5.3a). The transition from ‘zipper-like’ to ‘button-like’ junction phenotype begins at E17.5 and is completed by P28 [84]. The mature state of lymphatic intercellular junctions is lost during the lymphangiogenic response in inflammation but can be restored by dexamethasone treatment, which appears to act through the direct regulation of glucocorticoid receptors in LECs [84] (Fig. 5.3a). Lymphatic endothelial-derived sphingosine-1-phosphate is another factor contributing to the maintenance of mature junctions, although this effect may be, in part, indirect due to increased lymphatic endothelial sprouting [85]. In contrast, inhibition of lymphangiogenesis using VEGFR-3-blocking antibody did not increase junction maturation [84], suggesting that VEGF-C/VEGFR-3 signaling and formation of specialized lymphatic junctions are not directly coupled. In summary, intercellular junctions of capillary LECs are highly dynamic structures, which are regulated by a variety of pathological and physiological stimuli; this regulation may have important consequences for lymphatic vessel functions.

Fig. 5.3
Lymphatic vessel maturation. (a) Lymphatic endothelial cells in growing capillaries are connected by continuous adherens junctions, which are transformed into discontinuous ‘button-like’ junctions in mature lymphatic capillaries [84]. ‘Button-like’ junctions can revert to the ‘zipper-like’ state during inflammation. Dexamethasone promotes maturation of lymphatic endothelial cell (LEC) junctions. (b) Collecting lymphatic vessels develop from the capillary-like primary lymphatic plexus through the acquisition of lymphatic valves, deposition of basement membrane, and recruitment of smooth muscle cells. Lymphatic valve development is controlled by FOXC2/NFATC1/shear stress/connexin37 signaling, planar cell polarity proteins CELSR1 and VANGL2 regulate rotation and collective migration of lymphatic-valve-forming cells, and integrin-α9/fibronectin EIIIA promote extracellular matrix (ECM) fibril assembly and leaflet elongation. Ang2 and BMP9 are two growth factors important for formation of lymphatic valves. Recruitment of SMCs to collecting vessels is regulated by reelin and Ang2, whereas SEMA3A/NRP1/Plexin A1 signaling is important for keeping the valve area free of smooth muscle cells
5.4.2 Formation of Collecting Lymphatic Vessels
Lymphatic collecting vessel formation involves development of lymphatic valves, increased deposition of basement membrane components, and recruitment of smooth muscle cells. In the mouse embryo, mesenteric collecting lymphatic vessels begin to form around E15.5, and this process is accompanied by the downregulation of lymphatic capillary markers LYVE-1, VEGFR-3, and CCL21 [86]. Sites of forming lymphatic valves are marked by increased expression of PROX1 and the forkhead transcription factor FOXC2. Once specified, lymphatic valve-forming cells rotate and invaginate into the vessel lumen and further elongate to form bicuspid leaflets containing specialized ECM, covered on each side by endothelial cells [86, 87]. Lymphatic valve endothelial cells express high levels of transcription factors PROX1, FOXC2, and GATA2, adhesion receptor integrin-α9, the glycocalyx component podocalyxin, and ECM components laminin-α5 and fibronectin EIIIA [86–89].
Formation of lymphatic valves is a complex morphogenetic process that requires cooperation of multiple signaling pathways (Fig. 5.3b). Inactivation of FOXC2 completely prevents formation of lymphatic valves and further maturation of vessels [86, 90]. Heterozygous loss-of-function mutations of FOXC2 are found in a human hereditary disease, lymphedema-distichiasis, characterized by hyperplastic initial lymphatic vessels and abnormal lymphatic drainage, likely due to absent or dysfunctional lymphatic valves [91–93]. Genome-wide analysis of FOXC2 DNA-binding sequences in LECs suggested cooperation between the FOXC2 and calcineurin/nuclear factor of activated T cells (NFAT) signaling pathways [86]. Indeed, activated nuclear NFATc1 is present in lymphatic valve-forming cells, and lymphatic endothelial-specific loss of the Ser/Thr phosphatase calcineurin, which controls NFATc1 activation, arrests the development of lymphatic valves [86, 89]. A number of gap-junction proteins are also implicated in the maturation of lymphatic vessels. Lymphatic valve development defects are documented in Cx37– and Cx43-deficient mice, as well as in mice double heterozygous for Cx43 and Cx37 [89, 94]. Mechanistically, loss of Cx37 does not affect lymphatic valve cell identity establishment but prevents formation of a defined lymphatic valve territory, and the number of valves is severely reduced in Cx37 −/− animals [89, 94]. In humans, mutations in GJC2 (connexin47) and GJA1 (connexin43) are associated with lymphedema [95–97], further highlighting the important role of connexins in lymphatic vascular biology.
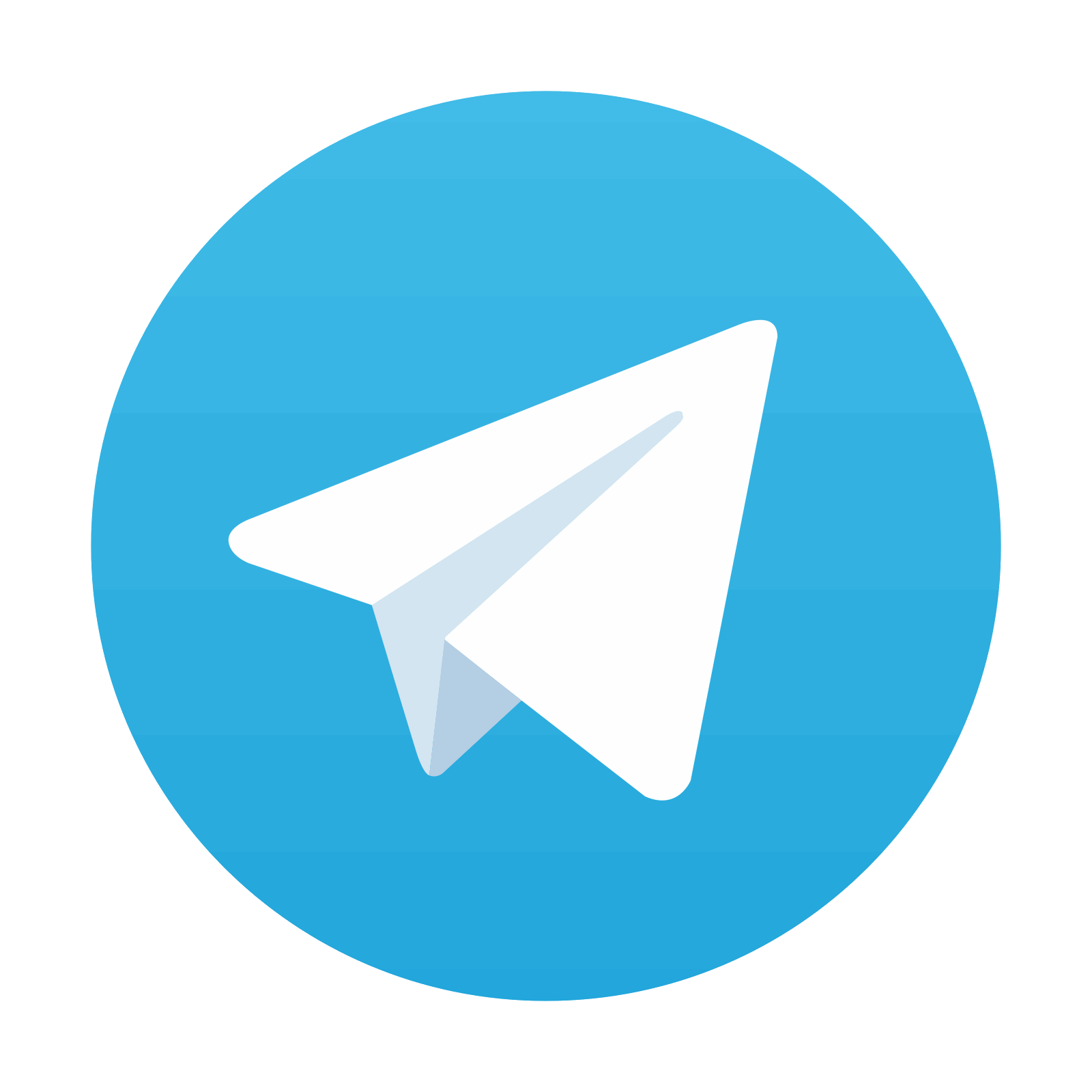
Stay updated, free articles. Join our Telegram channel

Full access? Get Clinical Tree
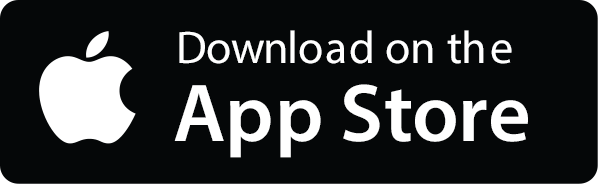
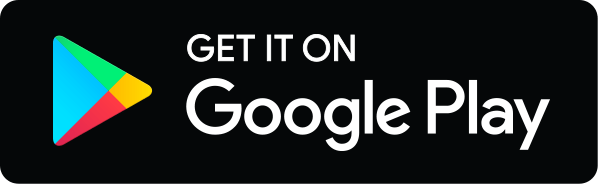