, Desa Lilic2, Joachim Roesler3, Tim Niehues4, Jean-Laurent Casanova5, 6 and Capucine Picard7
(1)
Division of Allergy and Clinical Immunology, Department of Pediatrics, Children’s Medical Center, Tehran University of Medical Sciences, Tehran, Iran
(2)
Primary Immunodeficiency Group, Institute of Cellular Medicine, The Faculty of Medical Sciences, Newcastle University, Newcastle upon Tyne, UK
(3)
Department of Pediatrics, University Clinic Carl Gustav Carus, Dresden, Germany
(4)
HELIOS Medical Center Krefeld, Academic Hospital of RWTH University Aachen, Immunodeficiency and Pediatric Rheumatology Division, Krefeld, NRW, Germany
(5)
St. Giles Laboratory of Human Genetics of Infectious Diseases, The Rockefeller University Hospital, New York, NY, USA
(6)
Necker Hospital and School of Medicine, University Paris Descartes, Paris, France
(7)
Study Center of Primary Immunodeficiencies and Pediatric Hematology-Immunology Unit, Necker Hospital, Paris Descartes University, Paris, France
Keywords
Innate immunityPattern recognition receptorsMendelian susceptibility to mycobacterial disease (MSMD)Herpes simplex encephalitis (HSE)Chronic mucocutaneous candidiasis (CMC)6.1 Introduction
Immune responses comprise innate and, where needed, adaptive steps. The responses of the innate immune system are mostly phylogenetically old, fast (e.g., phagocytosis of a bacterium) and encoded within the germ-line DNA, while responses of the adaptive immune system are first described in jawed vertebrates, and are slow, long-lived and antigen-specific as a result of somatic DNA recombination (e.g., T cell cytotoxicity to a virus infected cell).
Examples of the innate immune components are epithelial barriers, antimicrobial peptides, soluble factors (e.g., complement, chemokines) and cellular elements (e.g., neutrophils, monocytes and natural killer cells). Humoral and cellular components of the innate immune system are diverse, and their responses are often initiated by pattern recognition receptors (PRR) such as Toll-like receptors (TLRs) and NOD-like receptors (NLR; NOD, nucleotide-binding and oligomerization domain) that recognize pathogen-associated molecular patterns (PAMPs).
The important role of innate immunity in host defense is verified by potentially serious infections that result from naturally occurring defects of the innate immune system.
This chapter reviews PIDs that have been defined as defects of the innate immune system. The defects of neutrophil phagocytes and the complement system are covered in Chaps. 4 and 8, respectively. The development of human genetic theory of infectious diseases boosted up the field in the past two decades [47]. The study of otherwise healthy children, who develop a severe infectious disease, resulted in identification of monogenic inborn errors of immunity, mainly the innate system [47, 48]. The field is rapidly growing and description of more PIDs in this group can be expected in the near future. (See Table 1.5 and Fig. 1.12 for updated classification of defects in intrinsic and innate immunity: receptors and signaling components)
6.2 Anhidrotic Ectodermal Dysplasia with Immunodeficiency
(NEMO deficiency, IKBA gain-of-function mutations)
6.2.1 Definition
As NF-kB is a central transcription factor through which classical TLR activation triggers inflammatory responses, inadequate NF-kB activation will uniformly result in impaired TLR function. NF-kB is composed of homo- or heterodimers of five proteins belonging to the Rel family (p50, p52, RelA, c-rel and RelB) [118]. In resting cells, NF-kB is kept inactive in the cytoplasm through interaction with the inhibitors of NF-kB (IkBα, IkBβ and IkBε). In response to cell stimulation, IkBs are phosphorylated by the IkB kinase (IKK) complex, leading to subsequent degradation by the proteosome apparatus (Fig. 6.1) [170]. IKK consists of two catalytic subunits, IKKα and IKKβ, and a regulatory subunit, IKKγ, also known as the NF-kB essential modulator (NEMO) [155]. The release of NF-kB from IkB allows it to translocate to the nucleus and activate transcription of various genes involved in immunity [170]. Transcriptionally active NF-kB dimers are induced upon stimulation of a wide range of receptors of the immune system (TLRs, TNF receptor superfamilies, IL1 receptor family, and T and B cell antigen receptors and of receptors of ectodermal and bone cells). Impaired NF-kB activation by ectodysplasin in skin and altered receptor activator of NF-kB (RANK)-Ligand signaling in bone cells have been observed in ectodermal dysplasia (EDA) [88]. This explains the broad phenotype of patients with defects in NEMO-regulated NF-kB activation involving the immune system, ectoderm and bones. EDA is a unique feature of defects of NF-kB activation due to altered NEMO that is not observed in other disorders of TLR signaling.


Fig. 6.1
Toll–IL-1R domain (TIR) and NF-kB signaling pathways. Six proteins responsible for PIDs are shown in black
6.2.2 Etiology
The NEMO (IKBKG, OMIM*300248) gene is located on the X-chromosome, consists of ten exons and encodes a protein of 419 amino acids. This protein is an essential part of the IKK complex and consists of two N-terminal coiled coil domains followed by a leucine zipper and C-terminal zinc finger, all separated by α-helical regions [272]. X-linked anhidrotic ectodermal dysplasia with immunodeficiency (EDA-ID, OMIM*300291, 300584, 300301, 300636 and 300640) is a rare inherited disease caused by hypomorphic mutations in IKBKG, the gene encoding NEMO [12, 46, 52, 57, 72, 80, 88, 95, 100, 111, 114, 136, 138, 150, 159, 187, 188, 196, 207, 210, 222, 231, 240, 242–244, 251, 260, 277, 285, 298, 352]. More than 100 male patients with hemizygous hypomorphic mutations of NEMO have been reported to date and about 50 different mutations have been identified [12, 46, 52, 57, 72, 80, 88, 95, 100, 111, 114, 136, 138, 150, 159, 187, 188, 196, 207, 210, 222, 231, 240–244, 251, 260, 277, 285, 298, 352]. The disease is normally confined to males, although it has rarely been reported in females with skewed X-inactivation [182, 210]. More recently, mutations in the leucine zipper domain of the NEMO gene have been diagnosed as the X-linked form of Mendelian Susceptibility to Mycobacterial Diseases (XL-MSMD) [111, 150]. This finding illustrates the importance of NEMO for the IL12/IFNγ pathway. In contrast to hypomorphic mutations, amorphic mutations of NEMO cause incontinentia pigmenti which is a genodermatosis seen in females, as affected males die in utero [298].
Furthermore, an autosomal dominant form of EDA-ID (AD-EDA-ID, OMIN#612132) was identified in seven patients from six kindreds [66, 161, 201, 215, 237, 292]. In these patients, a heterozygous missense mutation has been identified in IKBA (NFKBIA, OMIM*164008) gene, which encodes an inhibitor of NF-kB, IkBα, have been identified [66, 161, 201, 215, 237, 292]. One of these patients displays complex partial mosaicism [161]. AD mutation in IKBA leads to a severe impairment of TCR signaling [66, 161, 201, 215, 237, 292]. The same heterozygous 94G>T mutation was identified in two unrelated kindreds. This mutation is responsible for the replacement of a serine residue important for the phosphorylation of IkBα with an isoleucine residue (S32I). The S32I, Q9X, Q14X and M37K mutations are gain-of-function mutations, as they increase the inhibitory capacity of IkBα by preventing its phosphorylation and degradation, resulting in the impairment of NF-kB activation [66, 161, 201, 237, 292]. The W11X nonsense mutation is responsible for haplo-insufficiency and less severe clinical, immunological and functional phenotypes in the patient bearing this mutation [215]. IkB molecules are involved in several pathways, including those triggered by the many members of the TNF-R, IL1R, TCR, BCR and TLR families. The T cell phenotype seen in AD-EDA-ID may reflect NEMO-independent NF-kB signaling in response to TCR/CD3-ligation [136, 260, 320].
6.2.3 Clinical Manifestations
The range of clinical manifestations of XL-EDA-ID is broad [136, 260]. About 80 % of the reported NEMO-deficient patients to date have had abnormal development of ectodermal derived structures, which is characterized by hypohidrosis, widely spaced cone- or peg-shaped teeth and hypotrichosis [136, 260]. These features result from defective signaling via the ectodysplasin receptor (EDAR) signaling pathway. A severe form of XL-EDA-ID exhibiting osteopetrosis and lymphedema (XL-OL-EDA-ID) has been reported in few patients carrying mutation in NEMO at the C-terminus of the molecule [95, 207, 277]. In contrast, some NEMO-deficient children had ID without EDA [100, 222, 231, 242]. Poor clinical and biological inflammatory responses during infectious episodes are remarkable in NEMO-deficient patients [260, 272]. The severity of the course of infections is in contrast to the paucity of abnormalities in routine immunological tests.
NEMO-deficient patients are susceptible to severe bacterial infections of the respiratory and gastrointestinal tracts, skin, soft tissues and bones, and suffer from meningitis and septicemia during infancy [136, 260]. The infectious phenotype is characterized mostly by infections due to encapsulated pyogenic bacteria, such as Gram-positive (Streptococcus pneumoniae, Staphylococcus aureus) and Gram-negative (Haemophilus influenza, Pseudomonas aeruginosa) organisms [136, 260]. Infections caused by weakly pathogenic mycobacteria, such as Mycobacterium kansasii, Mycobacterium avium, and Mycobacterium bovis, have also been diagnosed in some NEMO-deficient patients [136, 260]. Viral infections reported include cytomegalovirus, herpes simplex virus, adenovirus, Molluscum contagiosum and human papilloma virus [136, 260]. Pneumocystis jiroveci has also been found as an opportunistic infection [136, 260]. One third of the NEMO-deficient patients died from invasive infections, demonstrating the severity of this disorder [136, 260]. Finally, the phenotype caused by NEMO mutations includes autoimmune phenomena such as hemolytic anemia, arthritis and inflammatory bowel disease-like colitis [136, 231, 244, 260].
Seven patients with AD mutation in IKBA have EDA, failure to thrive, recurrent opportunistic infections and chronic diarrhea early in infancy [66, 161, 201, 215, 237, 292]. One patient, whom displayed complex partial mosaicism, had a milder EDA phenotype [161]. The broad and profound immunological impairments of patients with AD mutation in IKBA are responsible for broad susceptibility to severe pyogenic bacterial infections (meningitis, sepsis, arthritis, enteritis, abscesses and pneumonia), fungi and severe viral infections [66, 161, 201, 215, 237, 292]. All patients have developed recurrent pyogenic bacterial infections, due to β hemolytic type A streptococci, S. aureus, P. aeruginosa, Klebsiella pneumoniae, Serratia marcesens and Salmonella typhimurium [66, 161, 201, 215, 237, 292]. Finally, six of these patients presented recurrent diarrhea and/or colitis [66, 161, 201, 215, 237, 292]. Thus, a diagnosis of gain of function mutation in IKBA should be considered in children with EDA and combined immunodeficiency.
6.2.4 Diagnosis
Severe infections in combination with the symptoms of EDA are indicating clinical signs. Scanty hair, thin skin, defective tooth formation, abnormal nails, and/or heat intolerance can be striking, but are variable, can also be discrete, and are sometimes absent. Poor inflammatory responses during severe infections raise suspicion [136, 260]. In XL-EDA-ID, immunodeficiency results from defective NF-kB activation through Toll-interleukin-1 receptor (TIR), CD40 and TNF signaling pathways [136, 260]. Immunologic evaluation shows an abnormal antibody response to glycans due to the altered TLR signaling [136, 260]. Most patients exhibit hypogammaglobulinemia with low serum IgG levels [136, 260]. A subset of patients may present with hyper-IgM phenotype due to altered CD40 signaling and isotype-class switching [136, 160, 260]. NK cell abnormalities have been demonstrated as well [240]. Most NEMO-deficient patients fail to produce IL10 in response to activation with TNF-α in whole-blood assays [136, 260]. AD-EDA-ID shares many similarities with XL-EDA-ID, but is also associated with severely impaired T cell function. Five of these patients had low proportions of memory CD4+ and CD8+ T cells and no TCR gamma/delta T cells. They displayed severe impairment of T-cell proliferation in response to anti-CD3 [66, 161, 201, 237, 292], had hypogammaglobulinemia and no production of specific antibodies [66, 161, 292]. The child with the W11X mutation presented only a defect of glycan antibody production [215]. To confirm the diagnosis and to differentiate it from specific defects of adaptive immunity NEMO and IKBA genes should be sequenced.
6.2.5 Management
All NEMO-deficient patients should receive prophylactic trimethoprim-sulfamethoxazole and/or penicillin V treatment. IgG substitution should be carried out in patients with NEMO deficiency presenting with a humoral defect. NEMO-deficient patients with functional B-cell defect should receive vaccines against S. pneumonia, H. influenza and N. meningitidis. Vaccination with live BCG is contraindicated for NEMO-deficient patients. The families and physicians of NEMO-deficient patients should note that it is important to initiate empiric parenteral antibiotic treatment against S. pneumoniae, S. aureus, P. aeruginosa and H. influenzae as soon as infection is suspected or the patient develops a moderate fever, without considering inflammatory parameters, as patients may die from rapid invasive bacterial infections despite appropriate prophylaxis. Intensive four-drug regimens for at least 12 months are necessary to treat atypical mycobacteria. Patients with a severe infectious phenotype should be considered for HSCT, but experience is very limited [95, 112, 218, 252, 259, 313]. For patients with AD IKBA mutation, a preventive treatment, including antibiotic prophylaxis with cotrimoxazole and/or penicillin V, should be proposed and IgG replacement are recommended. The recommendations for NEMO-deficient patients with fever should also be applied to IKBA mutated patients. HSCT has been reported in two patients with IKBA mutation having combined immunodeficiency [94, 112]. One of these patients is alive and well, 8 years after haploidentical HSCT, whereas the other patient died of bacterial sepsis during the period of aplasia [94, 112].
6.3 HOIL1 and HOIP Deficiencies
6.3.1 Definition
The linear ubiquitination chain assembly complex (LUBAC) has important functions in immunity and inflammation [32]. LUBAC assembled by a complex containing HOIL1 (also known as RBCK1), SHARPIN, and HOIP (also known as RNF31) were implicated in IL1R and TNFR signaling pathways [117]. This E3 ligase complex, which adds head-to-tail linear polyubiquitin chains to substrate protein, has been implicated in NF-kB signaling. SHARPIN and HOIP protein levels are low in the absence of HOIL1 and their restoration following HOIL1 re-expression suggests that LUBAC is a ternary complex [32]. LUBAC is involved in the NF-kB pathway and conjugates linear polyubiquitin chains onto specific lysine residues of NEMO [312]. It has been suggested that LUBAC facilitates the recruitment of the NEMO-/IKK complex to cytokine receptors, in at least some cell types [312]. The recognition of these linear ubiquitin chains by NEMO itself and, probably, by other components of the NF-kB cascade may then lead to a local accumulation of IKK kinases, favoring their trans-phosphorylation and activation. LUBAC in human is required for optimal responses to other members of the TIR family, such as TLR3, and of the TNF-R family, such as CD40 [32].
6.3.2 Etiology
The HOIL1 (HOIL1/RBCK1, OMIM*610924) gene encodes hemoxidized iron-regulatory protein ubiquitin ligase-1 (HOIL1). HOIL1 deficiency or Polyglucosan Body Myopathy 1 with Immunodeficiency (PGBM1) is an autosomal recessive (AR) immunodeficiency caused by homozygous or compound heterozygous mutation in HOIL1 gene. Two siblings with a compound heterozygous mutations have been reported [32]. They have a large deletion in HOIL1 (genomic rearrangement due to recombination between intron 1 of TRIB3 and intron 4 of HOIL1) which was predicted to result in the deletion of the HOIL1’s ubiquitin-like (Ubl) domain [32]. Their second mutation is a nonsense mutation (p.Q185X) and was predicted to result in premature truncation within the novel zinc finger domain of HOIL1 [32]. A homozygous small nucleotide deletion in the gene was identified in a third patient, which was predicted to result in the deletion (c.121_122delCT) of all functional domains of HOIL1 [32]. The Ubl domain is required for LUBAC formation and linear ubiquitination. Collectively, these genetic data suggest that the three patients have rare deleterious alleles of HOIL1 [32]. More recently, 14 patients from 10 unrelated kindreds with HOIL1 deficiency caused by homozygous or compound heterozygous mutations have been reported [232, 335]. All mutations except one are localized after Ubl domain. Only one missense mutation is localized before Ubl domain [232].
The HOIP (RNF31, OMIM*612487) gene consists of 21 exons and encodes a protein of 1072 amino acids. The encoded protein (HOIL-1-interacting protein, HOIP) contains RING finger motifs, ubiquitin-associated domains and ubiquitin-associated domain and is a component of E3 ubiquitin-protein ligase, LUBAC. Recently, HOIP deficiency is recognized as a hypomorphic autosomal recessive trait in a single patient [31]. Homozygous L72P missense mutation in the Pub domain resulted in diminished mRNA and protein expression. This domain is highly conserved and is important for the interaction of HOIP with OTULIN and CYLD, two deubiquitinases [101, 113, 291]. The L72P mutation destabilizes HOIP and, consequently, SHARPIN and HOIL1 [31].
6.3.3 Clinical Manifestations
The first three patients reported with HOIL1 deficiency were from two unrelated families displaying a paradoxical clinical phenotype combining auto-inflammatory syndrome and pyogenic bacterial diseases [32]. All three patients had recurrent systemic inflammatory symptoms from the first months of life [32]. These episodes generally occurred after simple viral infections of the upper respiratory tract and associated with cervical lymphadenitis and hepatosplenomegaly (HSMG). These episodes are recurrent and no infectious agent could be identified. Some of these episodes are associated with inflammatory bowel disease with abdominal pain, blood and mucus in the stools [32]. Gastrointestinal endoscopy revealed minimal colonic inflammatory lesions with moderate eosinophilic infiltration in the gut epithelium in two patients [32]. One patient also developed diffuse desquamative dermatitis and cheilitis. All three patients have developed recurrent pyogenic bacterial infections, due to S. pneumoniae, H. influenzae, Escherichia coli, Staphylococcus spp and Enterococci. Two of them died from invasive pyogenic bacterial infection in childhood [32]. The third patient had also chronic cytomegalovirus infection. Giardia intestinalis caused a gut infection in one other patient [32]. Amyotrophia, muscle weakness and failure to thrive have been observed in the three patients since infancy [32]. These patients developed muscular amylopectinosis, consisting of intracellular glycogen inclusions, complicated by myopathy and cardiomyopathy [32]. More recently, 14 patients from 10 unrelated kindreds with HOIL1 deficiency presented after neuromuscular and cardiac involvement secondary to amylopectinosis. Their clinical descriptions are succinct, but one of these patients, who had compound heterozygous mutations, including the same large deletion of HOIL1 of the first kindred reported and frameshift mutation, displayed a similar inflammatory clinical phenotype as the three patients firstly identified [232].
The phenotype of reported HOIP-deficient patient is reminiscent of HOIL1 deficiency with some distinctions [31]. The patient was susceptible to encapsulated bacteria since early infancy. She displayed auto-inflammation, like the first described HOIL1-deficienct patient series, but amylopectinosis was mild and limited to the skeletal muscles, sparing the cardiac muscle. A distinctive feature of the disease was development of lymphangiectasia during the second decade of life.
6.3.4 Diagnosis
Chronic clinical and biological (high rate of CRP and leukocyte count) inflammation with acute episodes of fever with cervical lymphadenitis and HSMG, diarrhea, are remarkable [32]. Between these episodes, biological indicators of inflammation can decrease, but never reach normal levels. Immunologic evaluation shows a B-cell deficiency and a partial defect in antibody production against H. influenza type b and pneumococcus in two patients [32]. All patients with HOIL1 deficiency developed accumulation of polyglucosan (amylopectinosis), consisting of intracellular glycogen inclusions, that is associated with muscle weakness and cardiomyopathy [32, 232, 335].
Despite clinical similarity, mild amylopectinosis of skeletal muscles and presence of lymphangiectasia are specific features of HOIP deficiency that have not been observed in HOIL1 deficiency [31].
Characteristic immunological features of HOIP deficiency include, broad impairment of antibody production, defects of CD40-ligand induced B cell activation and plasmablast differentiation. In contrast to HOIL1 deficiency, the HOIP-deficient patient had severe naïve T lymphocytopenia and increased effector memory CD4+ and TEMRA CD8+ T cells [31].
6.3.5 Management
HOIL1-/HOIP-deficient patients should be immunized with S. pneumoniae conjugated and nonconjugated vaccines, H. influenzae conjugated vaccine and N. meningitidis conjugated and nonconjugated vaccines. They should receive prophylactic penicillin V treatment. IgG substitution should be considered in patients presenting with an impairment of B-cell immunity [31, 32]. Pyogenic bacterial infections should be treated aggressively. Patients with heart failure should be considered for heart transplantation, but experience is limited [232, 335]. Only one HOIL1-deficient patient with a severe infectious phenotype received HSCT [32]. After HSCT, the biological indicators of inflammation reached normal levels. The patient presents no further invasive bacterial infections after HSCT [32]. Due to underlying T cell deficiency, HOIP-deficient patients should be closely monitored for development of pertinent infections.
6.4 IRAK4 and MyD88 Deficiencies
6.4.1 Definition
TLRs play a crucial role in the evolutionary conserved innate immunity. TLRs are type I transmembrane proteins that function as homodimers or heterodimers. They are activated upon binding to bacterial, viral and fungal components [172]. To date, ten human TLRs have been identified [172]. It has been shown that TLRs are activated by specific agonist. For example, lipopolysaccharide (LPS) of Gram-negative bacteria is recognized by TLR4 in connection with LPS-binding protein and CD14 [223]. TLR2, in concert with TLR1 or TLR6, recognizes various bacterial components, including peptidoglycan, lipopeptide and lipoprotein of Gram-positive bacteria [223]. TLR3 recognizes double-stranded RNA (dsRNA) that is produced from many viruses during replication [223]. Agonists for other TLRs include flagellin for TLR5, synthetic imidazoquinoline-like molecules and single-stranded RNA (ssRNA) for TLR7 and TLR8, and bacterial and viral CpG DNA motifs for TLR9 [223]. After recognition of microbial pathogens, TLRs trigger intracellular signaling pathways that result in the induction of inflammatory cytokines and type I interferons (IFN) (Fig. 6.1). TLRs share with members of the IL1 receptor family an intracellular domain called the Toll-interleukin-1 receptor (TIR) domain [86]. The classical TLR-mediated intracellular signaling involves TIR-containing cytosolic adaptor molecules myeloid differentiation factor-88 (MyD88) and Toll-interleukin-1 receptor domain containing adaptor protein (TIRAP). Activation through this pathway results in the activation of NF-kB and mitogen-activated protein kinases (MAPKs), via the interleukin-1 receptor-associated kinase (IRAK) complex. The classical pathway culminates in the induction of inflammatory cytokines such as TNFα, IL6, IL1β and IL12. IRAK4 and MyD88 are molecules acting downstream from all TLRs except TLR3.
6.4.2 Etiology
Two Mendelian PIDs associated with impaired signaling of the TIR canonical pathway have been reported; mutations in IRAK4 (OMIM*607676) and MYD88 (OMIM*612260) [262, 333]. IRAK4 is a protein kinase that plays an essential role in TLR signaling of all known TLRs (except for TLR3) (Fig. 6.1) [2]. It interacts with MyD88 and activates IRAK1. Once hyperphosphorylated, IRAK1 associates with TNF receptor-associated factor 6 (TRAF6), triggering activation of NF-kB and MAPKs pathways [236]. IRAK4 deficiency is an autosomal recessive immunodeficiency caused by homozygous or compound heterozygous IRAK4 gene mutations [11, 36, 46, 54, 64, 74, 75, 137, 142, 184, 189, 216, 260, 262, 265, 282, 306, 307, 322, 344, 345]. All the reported mutations truncated the kinase domain. The Q293X mutation is seen in half of identified patients, reflecting a mutational hot spot [265]. MyD88 deficiency is an autosomal recessive immunodeficiency caused by homozygous or compound heterozygous MYD88 gene mutations [65, 260, 265, 333].
6.4.3 Clinical Manifestations
Patients with IRAK4 and MyD88 deficiencies present narrow susceptibility to invasive pyogenic bacterial infections and have normal resistance to common fungi, parasites, viruses, and many other bacteria. In both IRAK4 and MyD88 deficiencies, most of the invasive bacterial infections observed are caused by S. pneumoniae, S. aureus and P. aeruginosa, in particular [260, 265]. These infections can be recurrent. Gram-negative bacteria have been found in two such patients (Neisseria meningitides, Shigella sonnei and Salmonella spp) [54, 216, 265]. Infections mostly strike in infancy and early childhood, frequently presenting as meningitis, arthritis, and/or septicemia. Fever and other systemic inflammatory responses are low or absent [265]. About 40 % of IRAK4- and MyD88-deficient patients died of invasive bacterial infections (mostly of invasive pneumococcal disease), all before the age of 8 years, and most before the age of 2 years [265]. However, both PIDs improved with age, and patients with IRAK4 and MyD88 deficiencies present no further invasive bacterial infections after their teens. Patients with IRAK4 and MyD88 deficiencies also develop non-invasive pyogenic bacterial infections, mostly affecting the skin and upper respiratory tract sites, at which necrotizing infections are particularly common. All these patients are continuing to suffer from skin infections, sinusitis or pneumonia, including those that have reached adulthood [265]. Transient neutropenia can be associated with the infections. Delayed separation of the umbilical cord may also occur in IRAK4 deficiency [307].
6.4.4 Diagnosis
In whole-blood assays, leukocytes from IRAK4- and MyD88-deficient patients do not produce IL6 and no CD62L shedding from granulocytes is observed in response to activation with most of the TLR and IL1R agonists tested [265, 332]. The defects observed abolished all TLR responses (with the exception of those to TLR3 and a few TLR4 responses), and all IL1R responses (at least IL-1β, and IL18) tested, in all hematopoietic and non-hematopoietic cells from all patients tested. However, there seems to be no overt defect of leukocyte development in IRAK4- and MyD88-deficient patients and these patients have normal antigen-specific T- and B-cell responses, as shown by normal findings for immunological analyses, with three notable exceptions [265]. First, impairment of neutrophil migration [36]. Second, marginal zone B (IgM+IgD+CD27+) cells are markedly reduced in IRAK4- and MyD88-deficient patients [338]. Third, specific IgG and IgM antibody responses to pneumococcal and isohemagglutinuns have been shown to be impaired in up to 50 % of the patients explored [265]. MyD88 has recently been shown to control signaling downstream from TACI [154]. Some of the modest, subclinical abnormalities of B-cell responses, such as the production of low levels of antibodies against carbohydrates in some patients, may thus reflect impaired TACI responses, rather than impaired TLR and IL1R responses [265]. Low fever and low systemic inflammatory parameters in a patient’s serum such as CRP that contrast with a severe clinical course of infectious diseases should alert the physician to consider IRAK4 or MyD88 deficiency or other defects in TLR signaling. However, pus formation has been observed at various sites of infection.
6.4.5 Management
MyD88 and IRAK4 deficiencies are phenocopies in terms of their immunological and clinical phenotypes [333]. IRAK-4 and MyD88 deficiencies are life-threatening, resulting in the deaths of half of identified patients [117]. Patients with IRAK4 and MyD88 deficiencies should be immunized with conjugated and non conjugated S. pneumoniae vaccines, conjugated H. influenzae vaccine and conjugated and non conjugated N. meningitidis vaccines. A preventive regimen, including antibiotic prophylaxis with cotrimoxazol plus penicillin V (or equivalent), is required throughout the patient’s life, with empirical intravenous or subcutaneous IgG injections until at least the age of 10 years [87]. This prophylaxis seems to have an impact on the incidence of invasive bacterial infections [265]. Both clinical status and outcome improve with age and prophylactic treatment appears to be beneficial in these patients. This dramatic improvement with age may be related to the development of adaptive antigen-specific T- and B-lymphocyte responses. The caregivers must be aware that the clinical and laboratory signs of infection are subtle. Early detection of pyogenic infections and rapid institution of appropriate antibiotics are lifesaving.
6.5 Herpes Simplex Encephalitis
(TLR3 , UNC93B , TRAF3 , TRIF , TBK1 , IRF3 deficiencies)
6.5.1 Definition
This group of inherited disorders leads to impaired TLR3 signaling and susceptibility to Herpes simplex encephalitis (HSE) in childhood. The affected patients bear mutations in TLR3, UNC93B1, TRAF3, TRIF, TBK1 or IRF3 genes. The signaling pathway controlled by TRIF, which is mediated by TLR3 and TLR4, leads to activation of the transcription factors IRF3 and NF-kB (Fig. 6.2) [172]. TRIF recruits TRAF6 and activates TAK1 for NF-kB activation. TRIF also recruits a signaling complex involving TBK1 and IKKε via TRAF3 for IRF3 activation [172]. This signaling pathway induces the production of type I and type III IFNs and inflammatory cytokines, and is important in anti-viral immunity [177]. UNC93B is a 12-transmembrane domain protein present in the ER and delivers the nucleotide-sensing receptors TLR3, 7, 8 and 9 from the ER to endolysosomes [177]. TRAF3 has functions downstream from multiple TNF receptors and the receptors inducing IFNα, β, and λ production, including TLR3. UNC93B and TRAF3 defects also impair the TLR7-9 pathway, but with no known clinical consequences.


Fig. 6.2
TLR3 signaling pathway. TLR3 signaling is initiated by the recognition of dsRNA, inducing activation of the IRF3 and NF-kB pathways via TRIF, leading to the production of IFNα/β and/or IFN-λ. Deficiencies of the components of this pathway lead to herpes simplex encephalitis (HSE)
6.5.2 Etiology
Heterozygous mutations of TLR3 have been documented as cause of HSE in otherwise healthy children [350]. Autosomal Dominant (AD) form of TLR3 deficiency due to a heterozygous mutation of TLR3 with dominant-negative effect was identified in 2007 [350]. The recent report of an AR form of complete TLR3 deficiency (OMIM*613002) in a young adult who had developed HSE during childhood further illustrates the crucial importance of TLR3 signaling in fibroblasts for protective immunity to HSV1 in the Central Nervous System (CNS) [129]. AR UNC93B deficiency was identified in 2006 as the first genetic etiology of isolated HSE (OMIM*610551) [50]. Three individuals from two consanguineous kindreds were found to carry homozygous mutations in UNC93B1. As UNC93B is implicated in TLR3 signaling, it seems that impaired TLR3-dependent induction of IFNα/β and γ is involved in HSE [349]. A French patient with AD TRAF3 deficiency (OMIM*614849) and HSE has been identified in 2010 [257]. The de novo germline TRAF3 heterozygous missense mutation in this patient is loss-of-expression, loss-of-function and dominant-negative. Two kindreds with childhood HSE associated with TRIF deficiency (OMIM*614850) were recently identified in 2011 [289]. The first patient, from consanguineous kindred, was found to have a homozygous nonsense mutation (R141X) in the N-terminal region of the TRIF gene resulting in complete absence of protein [289]. The second patient, from a non-consanguineous kindred, has a heterozygous missense mutation (S186L) in the N-terminal part of the TRIF gene, suggesting that this hypomorphic S186L allele of TRIF is dominant negative and responsible for autosomal dominant AD TRIF deficiency [289]. Two kindreds with childhood HSE associated with TBK1 deficiency were recently identified carrying different heterozygous mutations (D50A and G159A) in TBK1, the gene encoding TANK-binding kinase 1, a kinase at the crossroads of multiple IFN-inducing signaling pathways [140]. Both mutations are associated with an AD trait but by different mechanisms, haplotype insufficiency (D50A) or negative dominance (G159A) [140]. Finally, heterozygous mutation of IRF3 has been documented as cause of HSE (OMIM*616532) in 2015 [9]. AD R285Q mutation impaired signaling trough TLR3-TRIF pathway.
6.5.3 Clinical Manifestations
Two of seven patients with AD TLR3-deficiency had developed HSE during childhood [350]. Five individuals, who carry the same heterozygous mutation in TLR3, are now adults and have not developed HSE, despite serologically documented HSV1 infection. The patient with AR complete TLR3 deficiency developed one HSE episode during childhood [129]. Two of the three UNC93B-deficient individuals developed HSE [50]. The first patient had recurrent episodes of HSE at the age of 11 months, 14 months and 3 years and half. The second UNC93B-deficient patient presented recurrent episodes of HSE at the ages of 5 and 17 years. Both UNC93B-deficient patients are now adults and have experienced no subsequent acute events [50]. One sibling of the second patient, who carries the same homozygous mutation in UNC93B1, is now adult and has not developed HSE, despite serologically documented HSV1 infection [50]. The first clinical signs of HSE in the TRAF3-deficient patient appeared at the age of 4 years [257]. The patient with TRAF3 deficiency and HSE described here is now adult and has otherwise remained healthy with no prophylaxis. She shows normal resistance to other infectious diseases, including viral diseases in particular. The patient with the AR TRIF deficiency had no infectious problems until age 2, when he developed HSE complicated by seizures, EEG abnormalities, atrophy of the left temporal lobe, and delayed speech [289]. Patient with AD TRIF deficiency has HSE, although with incomplete penetrance as only one of the three S186L TRIF heterozygote individuals developed HSE following HSV1 infection at age 21 months [289]. She responded to treatment with acyclovir and never relapsed, but has neurologic sequelae, consisting of blindness and epilepsy. Two of three patients with TBK1 deficiency suffered from HSE at the ages of 11 months and 7 years [140]. They are now 17 and 26 years old, respectively, and have suffered no other unusual infectious diseases, viral in particular, in the absence of prophylaxis. One adult individual who carry the same heterozygous mutation in TBK1, has not developed HSE [140].
A Danish girl was found to carry homozygous mutations (R285Q) in IRF3 [9]. She received acyclovir as inpatient treatment; however, the neurologic deficits persisted after discharge. The mutation was also present in the healthy father, consistent with incomplete clinical penetrance.
6.5.4 Diagnosis
HSE certainly raises suspicion of TLR3, UNC93B, TRAF3, TRIF, TBK1 or IRF3 deficiency. However, it is reasonable to presume that any severe HSE infection, even a single one, is due to an immunodeficiency of a known or unknown type. All standard immunological parameters, such as the numbers of blood phagocytes, B and T lymphocytes, lymphocyte subset distribution, NK cells, antibody production, and T-cell proliferation in response to the mitogens and antigens tested, were all normal in all patients tested. Blood leukocytes (monocyte-derived dendritic, NK, and CD8 T cells) from TLR3-deficient patients have an impaired response to stimulation with TLR3 agonist (polyinosinic-polycytidylic acid (polyI/C)). The fibroblast cells of the TLR3-deficient patients produce low levels of the antiviral molecules IFNβ and IFNλ in response to TLR3 agonist, HSV1, and vesicular stomatitis virus (VSV), leading to higher levels of viral replication and virus-induced cell death than for healthy control cells [350]. These observations suggest that the TLR3-dependent generation of IFNα, IFNβ, and IFNλ is critical for primary immunity to HSV1 in the CNS but redundant for immunity to most other viral infections [350]. UNC93B deficient fibroblasts and leukocytes did not respond to TLR3, or to TLR7-9 agonists respectively, in terms of IFNα, β and λ production [50]. The fibroblasts from UNC93B-deficient patients displayed abnormally high levels of viral replication and cell death after infection with HSV1. Fibroblasts from the patients displayed impaired responses to TLR3 agonist stimulation, in terms of IFNβ and λ production. Various TRAF3-dependent pathways were impaired in the patient’s cells, including the IFNα/β-, and λ-inducing and TNFR-responsive pathways [257]. However, there was sufficient residual TRAF3-dependent signaling for most defects to remain clinically silent. By contrast, the impaired TLR3 response was symptomatic and caused HSE, implying that the TLR3 pathway is critically dependent on TRAF3 and essential for immunity to HSV1 in the CNS [257]. The loss- of -expression/function of TRIF resulted in the abolition of TLR3-mediated signaling and TRIF-dependent TLR4 responses as measured by IFNβ and IFNλ production [289]. A defect in polyI/C–induced TLR3 responses can be detected in fibroblasts heterozygous for G159A but not for D50A TBK1 variant [140]. Nevertheless, viral replication and cell death rates caused by two TLR3-dependent viruses (HSV1 and VSV) were high in fibroblasts from both patients, and particularly so in G159A TBK1 fibroblasts. The IFN responses to the TLR3-independent agonists and viruses tested were maintained in both patients’ peripheral blood mononuclear cells and fibroblasts [140].
The R285Q IRF3 variant cannot undergo serine phosphorylation or dimerization and thus fails to activate transcription [9, 348]. This IRF3 mutation impairs the induction of antiviral IFNα/β in response to various stimuli. Moreover, IFNβ induction in heterozygous fibroblasts stimulated by poly(I:C), and HSV1 is also impaired.
6.5.5 Management
A secondary preventive treatment regimen with acyclovir should be proposed in patients presenting an impairment of TLR3 signaling pathway. Treatment with recombinant IFNα, in parallel with acyclovir, may be considered in patients with TLR3, UNC93B, TRAF3, TRIF, TBK1 and IRF3 deficiencies during acute HSE episode. The management of these patients is not still well established and will require supplementary clinical studies, notably regarding the duration of the preventive treatment.
6.6 Mendelian Susceptibility to Mycobacterial Diseases
(IFNγ receptor 1/2 deficiencies, IL12/23 receptor β1 chain deficiency, IL12p40 deficiency, DP- STAT1 deficiency, LZ-NEMO deficiency, Macrophage-specific CYBB deficiency, AD-IRF8 deficiency, ISG15 deficiency)
6.6.1 Definition
The IL12/Interferon-γ dependent signaling pathway is central to controlling mycobacterial infections (Fig. 6.3). Upon phagocytosis of such bacteria, macrophages secrete IL12p70, a heterodimer of IL12p40 and IL12p35 that stimulates Th1 T cells and NK cells through activation of IL12R. This receptor is composed of two chains, IL12Rβ1 and IL12Rβ2 [110] that associate with TYK2 and JAK2 kinases. Activation of this complex by IL12 ligand promotes signal transducer and activator of transcription-4 (STAT4) phosphorylation and nuclear translocation, and thereby induces IFNγ production and secretion [337]. IFNγ acts through its receptor, IFNγR, a heterodimer of IFNγR1 and IFNγR2, on macrophages and other cells.


Fig. 6.3
IFNγ and IL12 signaling pathways in immunity against mycobacteria and some other intracellular organisms. Structures affected in MSMD are marked in blue. MB mycobacteria
Analogous to IL12 signaling, IFNγR associates with JAK1 and JAK2. A series of phosphorylation steps leads to homodimerization of signal transducer and activator of transcription-1 (STAT1). This phosphorylated STAT1 translocates to the nucleus to initiate the transcription of IFNγ-inducible genes [16]. The respective gene products endow the macrophages with tools to confine (e.g., via granuloma formation) and finally kill mycobacteria.
Molecular defects in IL12/IFNγ dependent signaling cause rare genetic disorders. Theses defects belonging to the group of Mendelian susceptibility to mycobacterial diseases (MSMD) are characterized by disseminated or localized infections caused by either environmental mycobacteria (EM), BCG vaccine strains, or even Mycobacterium tuberculosis in otherwise healthy individuals [3, 49, 110]. Severe disease caused by non-typhoidal and typhoidal Salmonella serotypes is also common. Defects in IFNGR1 (OMIM*107470), IFNGR2 (OMIM*147569), IL12RB1 (OMIM*601604), IL12B (OMIM*161561), STAT1 (OMIM*600555), IRF8 (OMIM*601565), ISG15 (OMIM*147571), CYBB (OMIM*300481) and NEMO (OMIM*300248) have been identified in MSMD patients (Table 6.1) [3, 28, 42, 133].
Table 6.1
Genetic defects that cause Mendelian susceptibility to mycobacterial disease
Gene | Inheritance | Infections | |||
---|---|---|---|---|---|
Mycobacteria | Salmonella | Viruses | Other | ||
IFNGR1 | AR | ++ | + | +, HHV8 | Listeria monocytogenes, Shigella sonei, Haemophilus influenza, Leginella spp., Mycoplasma pneumonia, Klebsiela spp. |
AD | ++ | + | − | Histoplasma capsulatum, coccidioidomycosis | |
IFNGR2 | AR | ++ | − | +, CMV | − |
AD | ++ | − | − | − | |
IL12B | AR | ++ | ++ | − | Nocardia astroides |
IL12RB1 | AR | ++ | ++ | − | Paracoccidoides brasiliensis, chronic mucocutaneous candidiasis, leishmaniasis |
STAT1 | AD | ++ | − | − | − |
ISG15 | AR | ++ | − | − | − |
IRF8 | AD | ++ | − | − | − |
NEMO | XR | ++ | + | + | + |
CYBB | XR | ++ | − | − | − |
6.6.2 Etiology
IFNγR1 deficiency was the first identified genetic disorder recognized as MSMD [163, 229]. Such mutations can be recessive or dominant and are reported in about 39 % of patients with MSMD [3, 110]. In recessive complete (RC) forms, mutations involve the extracellular domain of IFNγR1 and most of them result in complete lack of cell surface receptor (nonsense mutations, frameshift) [8, 91, 144, 163], whereas some allow for receptor expression, but lead to impaired IFNγ binding (in-frame deletions) [164].
A recessive partial (RP) IFNγR1 deficiency with reduced, but not absent, IFNγR1 responsiveness caused by an I87T mutation has also been reported [166, 274].
Dominant partial (DP) IFNγR1 deficiency is due to heterozygous truncations of the cytoplasmic domain of IFNγR1 resulting in accumulation of non-functional receptor molecules at the cell surface [14, 167, 327, 334]. 818del4 is a hotspot for DP-IFNγR1 deficiency. Mutations in IFNGR2 have been identified less often than in IFNGR1.
Recessive complete forms (RC) of IFNγR2 deficiency result mostly in an entire loss of receptor. Sometimes the expression of non-functional receptor molecules has been documented [90, 281, 331]. Several of IFNGR2 mutations cause abnormal N-glycosylation and misfolding of the IFNγR2 protein [221, 331]. The additional polysaccharide is responsible for defective IFNγ signaling. Recessive partial (RP) IFNγR2 deficiency has been reported in a patient with the missense mutation located in the extracellular domain [87]. Furthermore, a 791delG mutation that causes complete IFNγR2 deficiency in the homozygous cells exerts a dominant-negative effect in heterozygous state [281]. Recently the heterozygous loss-of-function 186delC mutation in IFNGR2 is reported as the underlying defect of MSMD in a single patient [181].
IL12RB1 is the most frequent known genetic cause of MSMD seen in about 40 % of cases [3, 110]. The mutations are diverse and all cause recessive complete (RC) IL12 receptor β1 chain deficiency [6, 107, 108, 325]. IL12 comprises two subunits, p35 and p40, encoded by the IL12A and IL12B genes, respectively [317]. P40 subunit is also included in the structure of IL23.
STAT1 is required for cellular responses to both type I (IFNα/β) and type II (IFNγ) interferons. Activation through IFNα/β results in the formation of the transcription factor IFN-stimulated-γ-factor-3 (ISGF3) in addition to the transcription factor gamma activating factor (GAF). The latter is also the main transcription factor mediating cellular activation by IFNγ. Heterozygous missense mutations in STAT1 cause dominant partial (DP) STAT1 deficiency [55, 96]. In these mutations, the formation of sufficient ISGF3 is still possible and, consequently, type I interferon-mediated viral defense is not or only marginally impaired.
Mutations in the leucine zipper (LZ) domain of the NEMO have been discovered as the X-linked form of MSMD [111]. Impaired production of IL12 and IFNγ in response to CD40L largely accounts for the pathogenic effect of LZ-NEMO mutations in these patients [3, 111].
Another X-linked form of MSMD is caused by defect in CYBB-dependent NADPH oxidase assembly and respiratory burst in macrophages [42, 43]. Interestingly, the granulocytic respiratory burst is intact. The connection of the CYBB mutation with the IL12–IFNγ circuit is elusive.
Mutations in interferon regulatory factor 8 (IRF8), inducible by IFNγ, impair IL12 secretion by monocytes and dendritic cells [133]. Heterozygous IRF8 mutations underlie the partial IRF8 deficiency in a dominant-negative manner. Moreover, the circulating dendritic cells that produce large amounts of IL12 are lacking. Complete recessive IRF8 deficiency has a broader immunologic and clinical phenotype and discussed in Sect. 6.14.
Interferon-stimulated gene 15 (ISG15) is an intracellular ubiquitin-like molecule involved in ISGylation. However, it can be secreted from different leukocytes and induces the production of IFNγ from T cells and NK cells [27]. This function complements the role of the IL12– IFNγ circuit in the control of mycobacterial infection. Homozygous ISG15 mutations are reported in patients with MSMD only phenotype [28].
6.6.3 Clinical Manifestations
Susceptibility to weakly virulent mycobacteria, such as BCG vaccines is the rule. The patients are also vulnerable to more virulent species such as M. tuberculosis [3, 110]. Besides the exceptional diseases caused by some intracellular organisms, MSMD patients are resistant to most other microbial infections.
RC-IFNγR-deficient patients are most susceptible to severe, early onset mycobacterial infections with profoundly impaired granuloma formation [102]. In contrast, patients with partial IFNγR deficiencies acquire mycobacterial infections later in life, still sprout granulomas, and are likely to respond well to antimycobacterial antibiotics [44, 334]. A few of these patients present with nontyphoidal salmonellosis not restricted to the gut, histoplasmosis, or listeriosis [91, 279]. Viral infections with cytomegalovirus (CMV) and human herpes virus 8 (HHV-8) are reported in a few patients with RC-IFNγR1 deficiency [44, 92]. Interestingly, in DP-IFNγR1 deficiency osteomyelitis caused by environmental mycobacteria or BCG is common and more frequently observed than in RC-IFNγR deficiency (Fig. 6.4) [91, 279]. A clinical disease similar to Langerhans’ cell histiocytosis is reported in two patients with DP-IFNγR1 deficiency [98].


Fig. 6.4
Osteomyelitis in a patient with dominant partial IFNγR1 deficiency. Radiograph of the left shin showing osteolytic lesion in the left tibia (white arrow). Skeletal scintigram, showing an area of increased uptake of technetium in the distal end of the left tibia (black arrow)
The clinical phenotype of IL-12Rβ1 deficiency resembles that of DP-IFNγR deficiency, but nontyphoidal salmonellosis is more frequent as half the patients acquire this infection [76, 204]. Interestingly, these patients are also susceptible to clinical diseases caused by M. tuberculosis [7, 29, 45, 250]. Granulomas can still be formed but are often multibacillary [261].
A substantial portion of IL12Rβ1 deficient patients develop mucocutaneous candidiasis that is recurrent or persistent [76, 249]. Recurrent leishmaniasis has been reported in a patient with IL12Rβ1 deficiency [287]. The picture of IL12p40 deficiency is similar to IL12Rβ1 deficiency, although the clinical course is mostly more severe [266].
DP-STAT1 deficiency is associated with a relatively mild clinical course, resembling that of partial IFNγR deficiencies [55, 96]. Reported CYBB-MSMD and AD-IRF8 deficient patients were presented solely with mycobacterial diseases [229, 317].
Reported patients with LZ-NEMO mutations were vulnerable to mycobacteria primarily, or in association with other bacteria (e.g. H. influenza) [111].
The clinical and immunologic phenotypes of ISG15 deficiency resembled those of IL12Rβ1 deficiency, with impaired IFNγ immunity and relatively mild MSMD [28].
6.6.4 Diagnosis
In regions of the world that BCG vaccination is routine, clinical BCG disease could be the first and only presenting feature. Infections with environmental mycobacteria that clinically differ from the common and frequent infections of cervical lymph nodes in any respect such as unusual spreading, unusual types of mycobacteria, affection of internal organs and especially of bones are indicating clinical signs. It is important to note, however, that contaminations of patients’ samples with M. avium are common. These bacteria can also sometimes be found at sites of infections without doing much harm, e.g., at teeth. A careful histopathological and microbiological evaluation is therefore necessary.
It is revealing to look at the diagnoses that had been considered over long time periods by physicians before the right diagnosis of an MSMD was made. Environmental mycobacterial osteomyelitis was confused with eosinophilic granuloma, with chronic recurrent multifocal osteomyelitis or with tuberculosis several times, and infection of the gut with Crohn’s disease. Persistent pneumonia and hepatitis can also be due to mycobacteria in MSDM.
Diagnostic tests require in depth experience with cellular immunology laboratory testing and are only available in specialized laboratories.
IFNγR and STAT1 deficiencies are diagnosed by impaired cellular responses to IFNγ. In the PBMC (peripheral blood mononuclear cell) cultures, expression of MHC and TNFα production are impaired in response to LPS and IFNγ [90, 164]. In a whole blood assay, production of IL12 is impaired in response to BCG and IFNγ [105]. In the RC-IFNγR deficiency, high circulating levels of IFNγ are seen, presumably due to impaired receptor-mediated clearance [109]. Flow cytometric evaluation of IFNγR1 shows over accumulation of receptor subunit in patients with AD-IFNγR1 deficiency but the IFNγR2 expression is diminished in the case of AD-IFNγR2 deficiency [167, 181].
In most cases of IL12Rβ1 deficiency, no IL12Rβ1 is expressed on the cell surface and no response is seen to in vitro IL12 or IL23 [76, 108]. All known mutations of IL12B are recessive complete (RC) with a lack of detectable IL12p40 secretion by patients’ blood cells [261, 266].
Unlike the cells of CGD patients, neutrophils and monocytes from macrophage-specific CYBB deficiency display a functional respiratory burst and kill Staphylococcus aureus efficiently. However, monocyte-derived macrophages and EBV-transformed B cells show completely abolished respiratory burst function [42]. The IL12/IFNγ whole blood assay is normal in AD-IRF8 deficiency. However, the patients lacked CD1c+ CD11c+ circulating dendritic cells [133]. The immunological phenotype of ISG15 deficiency resembles those of patients with IL12p40 or IL12Rβ1 deficiency, with impaired IFNγ production in the whole blood assay [28]. Determination of ISG15 secretion from leukocytes could be used as screening. Finally, the diagnosis can be confirmed by sequencing the affected genes.
6.6.5 Management
In general, mycobacteria should be typified and infections treated according to growth pattern (slow or fast) and sensitivity. RC-IFNγR deficiency is a very severe disease with a poor prognosis. Most of the patients die during early infancy or childhood. The mycobacterial infections hardly respond completely to available therapy and subcutaneous application of pharmacological doses of IFNγ is useless [91]. Instead, IFNα could perhaps be helpful [336]. HSCT has been performed in some patients with variable results. A high rate of graft rejection was observed, at least when reduced conditioning was applied [53, 110, 147, 278].
Such infections in partial IFNγR deficiencies mostly respond favorably to appropriate antibiotics or even resolve spontaneously. Subcutaneous application of pharmacological doses of IFNγ in addition to antibiotics can be very helpful. Antimycobacterial treatment can eventually be stopped, but not within the first year after control of the infection is achieved [264]. However, a lifelong antimycobacterial prophylaxis may be required in some patients [139].
It is important to note that bone lesions caused by environmental mycobacteria are not always painful and bone stability may not always be secured. DP-STAT1 deficiency has a clinical course similar to those of patients with partial IFNγR deficiency and should be treated similarly.
Patients with IL12Rβ1/IL12p40 deficiency respond well to antibiotics and IFNγ; the overall prognosis is quite good [76, 266]. Salmonellosis is recurrent and may become resistant to eradication. Recurrent candidiasis should be treated with azole regimens, secondary prophylaxis is recommended for severe cases [249]. Mycobacterial infections in IRF8, ISG15 and macrophage-specific CYBB deficiencies are amenable to antibiotic combinations and possibly recombinant IFNγ.
6.7 Genetic Defects of Interferon Type I and III Responses Other than TLR3 Pathway
(AR STAT1 and STAT2 deficiency, TYK2 deficiency, IRF7 deficiency)
6.7.1 Definition
The significance of STAT1 has already been pointed out in Sect. 6.6. The different compositions of the transcription factors GAF (STAT1/STAT1) in the IFNγ pathway and ISGF (STAT1/STAT2/p48) in the IFN-α/β pathway provide an explanation for the different effects of variant forms of STAT1 and STAT2 deficiencies. In contrast to the dominant partial form (DP STAT1 deficiency), autosomal recessive STAT1 deficiency (AR STAT1 deficiency) does not only affect cellular responses to IFN-γ, but also those to IFN-α/β [97]. DP STAT1 deficiency is only dominant in the IFN-γ pathway, but recessive in the IFN-α/β pathway. However, if a mutated STAT1 allele has no dominant negative effect, it is recessive in both pathways because haploinsufficiency could never be found [30].
AR STAT1 and STAT2 deficiencies can cause diminished defenses against viral infections due to a lack of ISGF [30, 132]. The resulting disorders can be life threatening or moderate or even lack complete heritable penetrance. Furthermore, the STAT1 and two deficiencies reveal redundant defenses against many viruses [57, 132].
TYK2 (OMIM*176941) is a member of the JAK kinase family, with unclear role in different signaling pathways in the mouse model [302, 326]. However, it seems to be non-redundant in mice for cellular responses to IL12 and IFNα/β [169, 297]. It was originally described as essential for type 1 IFN signaling in a human fibroblast cell line [326]. The study of TYK2 deficient patients highlights its role in different cytokine receptor expression and signaling [176, 185, 219]. IRF7 is a transcription factor that amplifies type I and III interferon genes upon viral infections [145, 208]. Forward genetic studies identify IRF7 as an essential factor in anti-influenza immunity [60].
6.7.2 Etiology
Homozygous or compound heterozygous null mutations in STAT1 lead to complete unresponsiveness to IFNγ, IFNα/β, IFNλ, and IL27 because neither GAF nor ISGF can be formed [30]. Therefore, genes regulated by these transcription factors such as inducible NO synthetase and Mx proteins are not expressed upon stimulation with the respective interferons leaving patients susceptible to life threatening infections with mycobacteria and viruses (mainly herpes viruses). The clinical phenotype is milder if instead of null alleles, homozygous or compound heterozygous hypomorphic STAT1 mutations are present [56]. According to the compositions of GAF and ISGF, mutations in STAT2 that prevent measurable STAT2 protein expression leave responsiveness to IFNγ (and IL27) unaffected, but abolish response to IFNα/β (and IFNλ) [132]. Moreover, STAT2 deficiency is recently revealed to cause abnormal mitochondrial fission, linking the innate immunity to mitochondrial function [295].
All together, the following functionally aberrant STAT1 mutation types have been found and can lead to very different clinical pictures: Dominant negative (Sect. 6.6), and null alleles (Sect. 6.7), hypomorphic (Sect. 6.7), and hypermorphic alleles (Sect. 6.10) (Table 6.2) [200], and alleles that to date do not fit into a clear functional and clinical picture [296]. These heterozygous STAT1 mutations arise de novo and are associated with decreased STAT1 expression, sometimes chronic mucocutaneous candidiasis, autoimmunity, and severe infections. A progressive loss of lymphocytes and lymphocyte function is a feature very different from the other forms. Even though the latter mutations are not recessive they are mentioned here because they can also facilitate viral infections that can be fatal. Furthermore, there is some limited, but remarkable correlation of the different clinical pictures with the localization of mutations within different STAT1 domains [30].
Table 6.2
Genetic defects that cause impaired interferon type I and III responses
Genetic defect | Inheritance | Immunological phenotype | Clinical phenotype |
---|---|---|---|
Partial/complete STAT1 deficiency | AR | Impaired/abolished IFNα/β,γ,λ, IL27 responses | Intracellular bacterial (mycobacteria, salmonella) and viral diseases |
Partial STAT1 deficiency | AD | Selectively impaired IFNγ responses | Selective intracellular bacterial diseases (MSMD) |
Gain of STAT1 activity | AD | Enhanced IFNα/β,γ,λ, IL27 responses | CMC, other infections, autoimmunity, aneurysms, carcinomas |
Complete STAT2 deficiency | AR | Impaired IFNα/β responses | Severe viral infections (disseminated vaccine-strain measles), mitochondrial defect |
Complete TYK2 deficiency | AR | Impaired IFNα/β, IL12, IL23, IFNλ, IL10 responses | Intracellular bacterial (mycobacteria, salmonella) and viral diseases |
Complete IRF7 deficiency | AR | Impaired type I and type III IFN responses | Severe influenza infection |
TYK2 deficiency (OMIM*611521) was first considered to be an AR genetic etiology of HIES based on the observation of a single patient [219]. The impaired IL12 and IFNα/β signaling in this patient accounted for his susceptibility to intracellular organisms and viruses. HIES could be attributed to impaired IL6 cellular responses. The identification and immunological investigation of additional patients changed our perception of TYK2 deficiency as a unique clinical entity that does not match with HIES [176, 185]. Recently, compound heterozygous mutation of IRF7 (OMIM*616345) has been documented as cause of life-threatening influenza infection [61].
6.7.3 Clinical Manifestations
To date, only few patients with AR STAT1 and STAT2 deficiencies have been characterized. Therefore, it is unknown if these disorders have distinctive clinical features that are not shared by other immunodeficiencies. Patients with two null or hypomorphic STAT1 alleles are susceptible to mycobacteria including those that are non- or weakly virulent in healthy individuals such as Mycobacterium kansasii or BCG and also to viral infections [30].
If the disorder is caused by STAT1 null alleles the mycobacterial infections clinically resemble those found in complete IFNγ deficiency (Sect. 6.6). Multiple organs can be affected and blood cultures can be positive for mycobacteria. Such mycobacterial and viral infections are often life threatening early in life. HSV1 may cause lethal encephalitis and other viruses of the herpes group such as CMV may lead to severe infection. Remarkably, patients can fight other viral infections such as rhinoviruses, parainfluenza and live polio-vaccine indicating redundancy of anti-viral host defenses. In the case of hypomorphic STAT1 alleles that allow for some STAT1 signaling the viral and mycobacterial infections tend to occur later in life and the clinical course is milder.
Complete STAT2 deficiency has been described first in a patient as the reason for severe disseminated vaccine strain measles complicated by hepatitis and severe pneumonitis [132]. In the brother of this patient, this deficiency was the likely reason for a fatal short illness due to a suspected viral infection. In relatives with the same homozygous deficiency, viral infections tended to be milder or were even unremarkable and only detectable by serology. These findings support again the notion of redundancy in anti-viral defenses. Recently, three STAT2-deficient patients (including the first reported patient) were evaluated in detail [295]. Two Albanian siblings presented with neurological problems after MMR vaccination. These patients also had underlying mitochondrial problems.
The first TYK2 deficient patient displayed the characteristics of HIES, i.e. high circulating IgE levels, atopy and predisposition to cutaneous staphylococcal infections [219]. Moreover, he was susceptible to clinical disease caused by BCG and nontyphoidal Salmonella, and recurrent viral infections.
The second TYK2 deficient patient did not display HIES features, however like the first one, he suffered from intracellular infections (BCG and Brucella) and recurrent viral infections [176].
A recent report, described in detail the clinical and immunological characteristics of all eight identified TYK2 deficient patients including the first two ones [185].
Altogether, TYK2 deficient patients demonstrate a phenotype similar to partial AR STAT1 deficiency with susceptibility to diseases caused by intracellular bacterial and mild viral infections. TYK2 deficiency also should be considered in cases of severe tuberculosis in children. It is now clear that HIES is not a characteristic feature of TYK2 deficiency.
Finally, AR IRF7 (F410V, Q421X compound heterozygous) deficiency is discovered as the underlying defect in a girl who developed severe primary influenza infection during pandemic H1N1 2009 influenza A virus [61]. The patient did not experience severe infections caused by other viruses.
6.7.4 Diagnosis
Clinical clues to differentiate mycobacterial disease in MSMD from such infections in otherwise healthy individuals and important differential diagnoses have already been described in Sect. 6.6. Severe viral and especially herpes group infections do also occur in T cell deficiencies and in disorders of TLR3 and its signaling pathway. Further genetic defects of anti-viral defenses will most probably be discovered in the near future. If both, viral and mycobacterial infections occur within one family and no T cell deficiency (including HIV) is present then AR STAT1 deficiency is a likely diagnosis [30]. STAT2 deficiency is even rarer than STAT1 deficiency [132]. It should be considered as a differential diagnosis if no other clue for an exclusive susceptibility to viral infections is detected.
In AR STAT1 deficiency, high serum levels of IFNγ can be found. In whole blood assays, monocytes do not respond to BCG and IFNγ by producing normal amounts of IL12. Furthermore, cells from patients with AR STAT1 or STAT2 deficiency do not or not sufficiently respond to IFNα/β in terms of forming ISGF and inducing transcription of genes such as Mx that are regulated by ISGF. The respective genetic defects should of course be confirmed by sequencing. Electron microscopy of muscle and fibroblasts from the affected STAT2-deficient patients revealed long mitochondria, suggesting defective mitochondrial fission [295]. The mitochondrial fission protein DRP1 (dynamin related protein 1) was inactive after abnormal phosphorylation pattern induced by STAT2 absence.
All TYK2 deficient patients displayed impaired cellular responses to IL12, IFNα/β, IL23, IL10 and IFNλ [185]. In contrast, TYK2 is redundant for signaling downstream of receptors specific to IFNγ, IL21, and IL27. All TKY2 deficient patients except the first one responded normally to IL6. The expression of exogenous wild-type TYK2 in the first patient’s cells did not rescue IL6 hyporesponsiveness, an evidence against the role of TYK2 mutation as a cause [185].
The mutant IRF7 alleles fail to induce type I and type III interferons in pDCs. Moreover, increased influenza virus replication was documented in affected patient’s iPSCs-derived pulmonary epithelial cells [61].
6.7.5 Management
In complete AR STAT1 deficiency, HSCT should be attempted. Response to anti-viral and anti-mycobacterial medication is poor in contrast to the partial form [30]. In this case, additional therapy of mycobacterial infections with IFNγ and prophylactic therapy with azithromycin as in other partial defects of the IFNγ/IL12 axis may be considered. It is not yet clear to what extent adaptive immunity can compensate for the defect in anti-viral defense in the course of life. Any possible dangerous viral infection especially herpes encephalitis should be prompted by adequate antiviral medication as early as possible. The same may be true for STAT2 deficiency. At least some live viral vaccines such as measles are probably contraindicated [132]. In case of contact, immunoglobulins with sufficient titers should be applied. TYK2 deficient patients have been managed with specific therapies directed against each microbial or viral infection.
6.8 Warts, Hypogammaglobulinemia, Infections, Myelokathexis (WHIM) Syndrome
6.8.1 Definition
The WHIM syndrome is very rare and comprised of warts, hypogammaglobulinemia, infections, and myelokathexis (OMIM*193670) [122, 342]. Myelokathexis is the retention of mature neutrophils in the bone marrow [353]. Most patients with WHIM syndrome carry heterozygous gain of function mutations in the gene coding for the chemokine receptor CXCR4 [141].
6.8.2 Etiology
CXCR4 (CD 184) is a G protein-coupled receptor, including seven transmembrane regions, an amino-terminal extracellular domain, and an intracellular carboxy terminus. It is coded by CXCR4 gene (OMIM*162643) and expressed in the immune system and throughout the central nervous system [127]. The ligand of CXCR4, SDF1 (stromal cell-derived factor-1) or CXCL12, is produced by bone marrow stromal cells and a variety of other tissues. SDF1 interaction with CXCR4 plays a central role in bone marrow homing and trafficking of hematopoietic progenitor cells to the bone marrow [192, 303]. Many but not all WHIM cases have been linked to heterozygous mutations in CXCR4, all of which are clustered in the cytoplasmic tail of the receptor [127, 141]. Mutant receptors display gain-of-function properties leading to absent receptor desensitization, due to defective ligand-mediated receptor internalization and/or decreased phosphorylation of activated mutant CXCR4 [128, 173]. Increased responsiveness to SDF1 leads to pathological retention of post-mature leukocytes in the bone marrow (myelokathexis) [18, 290]. In mouse models with desensitization-resistant CXCR4 receptors, suppression of T and B lymphopoiesis and dysfunction of the immunological synapse were demonstrated [17, 168].
The mechanism for the susceptibility to human papilloma virus (HPV) infection is unknown. It is disproportional compared to other viruses. Moreover, an unusual Human Polyoma Virus has been detected in the skin of a WHIM patient (HPγ-10) [41]. WHIM patients show a significant decrease in plasmacytoid and myeloid dendritic cells [311], which may be important for antiviral immunity. Alternative to and independent of immunodeficiency it has been hypothesized that the mutant CXCR4 signalling directly stimulates keratinocyte transformation [59].
The absence of mutations in the CXCR4 gene in some patients supports the view that WHIM syndrome is genetically heterogeneous [18, 141]. Pathways linked to CXCR4 signalling and candidate genes in CXCR4 negative WHIM are GPCR Kinase-3 (GPK-3), Rac-2 (Rac-related C3 Botilinum toxin substrate 2) and Sphingosine-1 phosphatase receptor-5 (S1P5) [4].
6.8.3 Clinical Manifestations
HPV-induced warts may already appear in early childhood. While some individuals have relatively few or no warts, others are afflicted with extensive cutaneous verrucosis, including genital condyloma acuminata with dysplastic changes [122, 342].
Recurrent bacterial infections from infancy including pneumonia, sinusitis, otitis, cellulitis, periodontitis, and abscesses are caused by common pathogens. The clinical course is relatively benign, however, deaths from mycobacterial disease have been reported [81, 89, 127]. Malignancies comprise EBV positive B cell lymphomas, T-cell lymphomas, HPV induced vulvar, basal cell and oral squamous cell carcinomas [51, 153]. The overall cancer risk is estimated to be 30 % by the age of 40 years [19]. Finally, complex congenital heart defects (double aortic arch, tetralogy of Fallot) have been described infrequently [19].
6.8.4 Diagnosis
WHIM should be suspected in any patient with warts, leukopenia, neutropenia and hypogammaglobulinemia. Examination of bone marrow shows myeloid hypercellularity with morphologic abnormalities (Fig. 6.5) consistent with apoptosis (pycnotic, hypersegmented nuclei, and multiple small cytoplasmic vacuoles) [127]. Neutropenia in WHIM is severe, with absolute counts usually below 300/μL [82, 127]. During acute systemic infections, neutrophils can be recruited to the blood; even leukocytosis with neutrophilia is observed. Lymphopenia is less common than neutropenia. B lymphopenia has been described in several patients [13, 122] with marked reduction of CD27+ memory B cells [128]. Hypogammaglobulinemia is variable and may affect all isotypes [13, 122, 128]. T lymphocyte subsets and in-vitro lymphocyte proliferation to mitogens are abnormal in some but not all patients [127, 342]. Verification of disease causing mutations in CXCR4 (when present) confirms the diagnosis.


Fig. 6.5
Peripheral blood smear of a patient with WHIM syndrome. Polymorphonuclear cells showing hypersegmented nuclei with long filaments connecting nuclear lobes
6.8.5 Management
Plerixafor (Mozobil) is a small molecule CXCR4 antagonist. It was originally designed to inhibit HIV entry into CXCR4+ CD4 cells and then licensed for stem cell mobilization in the context of stem cell transplantation. In phase I trials it has now been used in WHIM at dosages from 0.02/mg/kg s.c. to 0.24 mg/kg at 2–4 day intervals and resulted in significant and sufficient recruitment of neutrophils into blood [73, 214]. Administration of granulocyte colony stimulating factor (G-CSF) or granulocyte-macrophage colony stimulating factor (GM-CSF) also causes a rapid increase in the neutrophil count and may normalize bone marrow cytology [146, 340, 341]. Prophylactic antibiotics and regular infusions of intravenous immunoglobulin are non-specific but may reduce the incidence of bacterial infections.
Warts are usually resistant to local therapy. The high risk of malignant transformation of genital warts is of concern and requires careful monitoring [83]. In one WHIM patient, immunization with a quadrivalent HPV vaccine led to HPV specific humoral and cellular immunity [134]. To what extent WHIM patients can be protected by HPV and other vaccines is unknown.
6.9 Epidermodysplasia Verruciformis
(EVER1 deficiency, EVER2 deficiency)
6.9.1 Definition
Epidermodysplasia verruciformis (EV; OMIM*226400) is a rare, hereditary disorder with an extremely increased susceptibility to HPV infection affecting the skin. EV was described by dermatologists from Basel, Switzerland, Lewandowsky and Lutz in 1922 and was probably both the first description of a PID and the first evidence for the involvement of HPV infection in the development of skin cancer [197, 202, 245, 248]. The majority of cases (approximately 75 %) is caused by mutations in the EVER1 (TMC6) and EVER2 (TMC8) genes [273]. Early development of widespread, refractory flat warts and pityriasis versicolor-like lesions are distinctive features. There is a specific susceptibility to β-HPV 3, 10 in plane warts, to β-HPV 5, 8 in skin carcinomas which emerge in over one-third of cases [202, 310].
6.9.2 Etiology
HPVs are small, non-enveloped double-stranded DNA viruses measuring about 55 nm in diameter [246], present in normal skin and are ubiquitous. EVER proteins are located in the endoplasmatic reticulum, forming a complex with zinc-transporter-1 (ZnT-1) [195]. A dysregulation of zinc-dependent transcription factors in keratinocytes might lead to their malignant transformation. A similar mechanism might be responsible for dysregulated T-cell activation, explaining EV in primary/secondary immunodeficiencies [194]. EV-like disease has been described in T-cell deficiencies such as SCID, RHOH, MST1/STK4 [1, 67, 68, 301, 346, 347] and secondary immunodeficiencies, e. g., HIV. In EV tumors, loss of EVER protein function may lead to increased transcription of the HPV genes E6 and E7 genes, which encode for the major oncoproteins responsible for the oncogenic potential of HPV. UV light is likely to be involved in the progression from benign warts to malignancy [256, 275]. EV is genetically heterogeneous, autosomal-dominant inheritance has also been described [213, 276].
6.9.3 Clinical Manifestations
EV patients are prone to a subset of HPVs, but not to other infections. There are two presenting conditions in EV [69]. First, disseminated, benign, plane warts (HPV3 and 10) begin during infancy and early childhood [158, 206]. The lesions start on the sun-exposed areas, are hypo- or hyperpigmented and may generalize to the limbs, neck, and trunk. The mucous membranes are rarely involved. The typical lesions are flat or presenting as pityriasis versicolor-like macules (Fig. 6.6). Second, there are malignant, verrucous lesions (HPV5 and 8), most frequently localized in sun-exposed areas, which develop in over one-third of cases after the second decade of life [202, 310]. Lesions converting to malignancy appear usually as actinic keratoses and are located mainly on the forehead [246]. Cancers develop slowly and are mainly locally destructive, but progressive extension and metastases have been observed in some patients [157, 203].


Fig. 6.6
Flat wart lesions and hypo/hyperpigmented (tinea versicolor-like) macules in a patient with epidermodysplasia verruciformis
6.9.4 Diagnosis
HPVs can be detected by in situ hybridization or polymerase chain reaction on skin tissue specimens. The classic histologic presentation of EV is a verruca plana-type lesion with minimal hyperkeratosis and acanthosis [234]. The cells of the upper epidermis have a clear, blue-gray pale cytoplasm and a central pycnotic nucleus. Appearance, clinical course, histopathological findings of skin lesions, and molecular HPV typing lead to the right diagnosis. EV can be confirmed in approximately 75 % of all cases by mutational analysis of the EVER1 or EVER2 genes. If αHPV infections occur with lymphedema and anogenital dysplasia this is called WILD (warts, immunodeficiency, lymphedema, dysplasia) syndrome [186].
6.9.5 Management
A definitive therapy for EV has not been available up to now. Lesions are refractory to conventional therapies and complete regression of HPV associated lesions has never been observed. Various topical (5-Fluorouracil, imiquimod) and systemic (interferon (alfa-2a) combined with retinoids, cimetidine) therapies have been tried with variable results [25, 126, 206]. Surgical removal is used for localized malignant and large disfiguring benign lesions. Strict sun avoidance should be started once the syndrome is diagnosed. There is a life-long risk of cancer; however, it may be helpful and reassuring for the patient to point out that over 60 % of patients do not develop skin cancer.
6.10 Chronic Mucocutaneous Candidiasis
(IL17RA deficiency, IL17F deficiency, IL17RC deficiency, STAT1 gain-of-function mutation, ACT1 deficiency)
6.10.1 Definition
Fungal infections are a huge albeit still largely unappreciated problem affecting an estimated one billion people worldwide. Of these, Candida infections are amongst the most frequent causing both chronic mucocutaneous and acute systemic disease [39].
Chronic Mucocutaneous Candidiasis (CMC) is an umbrella term describing a clinical phenotype presenting as persistent or recurring, debilitating infections of the skin, nails and mucous membranes with the yeast Candida, mostly albicans, although alternative strains (C. glabrata, C. kruseie, C. dubleniensis) are increasingly seen. Candida is an opportunistic yeast colonising gastrointestinal and urogenital mucosa in about 30–50 % of healthy humans, but in permissive circumstances such as primary or secondary immune deficiencies, will cause disease even death (Fig. 6.7) [198, 254, 329].


Fig. 6.7
Clinical presentation of chronic mucocutaneous candidiasis. Oral thrush and nail involvement in a child with the gain-of-function-STAT1 mutation (Courtesy of M. Abinun; Newcastle, UK)
Secondary CMC, usually presenting as oral thrush, can be precipitated by a range of recognised permissive factors (dentures, antibiotics, inhaled steroids, immunosuppressive drugs, diabetes, HIV/AIDS) and will resolve once these factors are eliminated. Interestingly, vulvovaginal candidiasis (VVC) is most frequently seen in otherwise healthy women, suggesting that in this condition, local protective mechanisms play a vital role [39, 198]
Primary CMC is caused by inborn errors of immunity, resulting in PID and susceptibility to infection. CMC (especially oral Candida infection – thrush), may occur in the context of a conventional PID, defined by an overt immunological phenotype resulting in a broad susceptibility to a range of microorganisms including viruses, bacteria and fungi, when the underlying defect damages T cell immunity (e.g. in severe combined immune deficiency – SCID). In contrast, CMC can present as a non-conventional PID defined as selective susceptibilities to weakly pathogenic and/or opportunistic microorganisms, in this case specifically to the fungus Candida [254, 329].
6.10.2 Etiology
Accumulating evidence suggests that mucocutaneous fungal infections such as CMC are seen in patients with primary defects affecting innate and adaptive immune responses that activate the Th17 pathway. Interestingly, CMC patients, with the exception of CARD9 deficiency (see below) rarely if ever develop invasive fungal disease [329].
The innate immune system recognises a range of conserved fungal PAMPs, initiating a rapid, conserved response, which activates inflammatory cells, that have a dual role of eliminating the intruder and initiating the appropriate adaptive immune response. The latter is achieved by engaging host PRRs that bind to fungal PAMPs, triggering signalling pathways which result in targeted cytokine production, recruitment and polarization of relevant T, B and NK lymphocyte subsets [227]. Fungal PAMPs are recognised by several families of PRRs: The most important fungi in human pathology (Candida, Aspergillus, Cryptococcus) are recognised by TLR2, TLR4 and TLR9 and heterodimers containing TLR1 and TLR6 [227]. Genetic mutations of TLRs and their adaptors (MYD88, IRAK4, TLR3) have been linked to increased susceptibility to bacterial [260] and/or viral [288], but interestingly, not to fungal infections, although TLR1 and TLR4 polymorphisms have been associated with increased susceptibility to invasive fungal disease [228] and TLR3 to cutaneous candidiasis [226]. C-type lectin receptors (CLRS) are PRRs that recognise carbohydrate structures on fungal and other pathogens. Dectin1 binds β-1,3-glucan, activating the Syk tyrosine kinase via a downstream complex of the cytosolic proteins caspase recruitment domain-containing protein 9 (CARD9), Bcl-10 and MALT1 to the NF-κB which acts as a central regulator in the production of inflammatory cytokines in neutrophils, macrophages and dendritic cells as well as promoting IL23 production and subsequent Th17 cell induction [93]. Polymorphisms of dectin1 [106] and mutations of CARD9 [121] were reported to underlie CMC in certain patients. (See Sect. 6.11 or more details) Defects of other CLRs (dectin-2, mannose receptor), NLRs, RigI helicases, etc. have not as yet been associated with fungal disease in humans [227].
The adaptive immune response is triggered upon binding of the cognate antigen to the TCR of naive CD4+ T cells, which are then initiated to differentiate into three effector lineages, depending on the local cytokine milieu provided by innate immune cells. The classical T helper type (Th1) cell is induced by IL12, regulated by the transcription factor T-bet and secretes IFNγ that promotes cell-mediated immunity, essential for dealing with intracellular microorganisms. Th1 cells were previously believed to be crucial in antifungal immunity but this role has now largely been taken over by Th17 cells [103, 271]. Th2 cells are induced by IL4, regulated by GATA3 and produce IL4, IL5 and IL13, which promote antibody production essential for protection against extracellular microorganisms. However, although fungi induce antibody production, humoral immunity is generally not protective [198]. Th17 cells develop in the presence of IL1β, TGFβ and IL6 which signals via STAT3 to induce expression of the Th17 lineage defining transcription factor retinoic acid-related orphan receptor (ROR)γt, while IL23 promotes further Th17 cell expansion and maintenance. Th17 cells secrete IL17A, IL17F and IL22 which promote cutaneous and mucosal immunity by activation of epithelial cells, granulopoesis, recruitment of neutrophils, production of chemokines and antimicrobial factors and are believed to play a crucial role in fungal immunity [269]. IL17 is a dimeric molecule that is biologically active as a homodimer of the IL17A chain, a homodimer of the IL17F chain, or a heterodimer of an IL17A and an IL17F chain. Any of these dimers can signal through binding to IL17RA [269].
In recent years, several mutations affecting the innate and adaptive pathways of fungal immunity have been reported, confirming and increasing our understanding of fungal immunity, specifically the crucial role of the IL17 pathway (Table 6.3).
Table 6.3
PIDs with increased selective susceptibility to mucocutaneous and invasive fungal infections
Genetic defect | Inheritance | Clinical phenotype |
---|---|---|
IL17F deficiency | AD | CMC, folliculitis |
IL17RA deficiency | AR | CMC, folliculitis |
IL17RC deficiency | AR | CMC |
ACT1 deficiency | AR | CMC, blepharitis, folliculitis, and macroglossia |
GOF-STAT1 mutation | AD | Various fungal, bacterial, and viral (HSV) infections Autoimmunity (thyroiditis, diabetes, cytopenia) Enteropathy |
CARD9 deficiency | AR | Invasive candidiasis infection Deep dermatophytoses Exophiala spinifera Phialophora verrucosa |
RORC deficiency | AR | CMC, mycobacterial infections |
AIRE deficiency | AR | CMC, Autoimmune Polyglandular Syndrome 1 |
6.10.3 Clinical Manifestations
In 2011, Puel et al. [268] reported the first two “proof of concept” genetic aetiologies of CMC: AR deficiency in the IL17 receptor IL17RA and AD deficiency of the IL17 cytokine IL17F. IL17RA deficiency was complete, abolishing cellular responses to IL17A and IL17F homo and heterodimers. By contrast, IL17F deficiency was partial, with mutant IL17F-containing homo and heterodimers displaying impaired but not abolished activity. In 2015, AR mutations of IL17RC were added as a third proof-of-concept genetic aetiology of CMC [199].
AR IL17RA deficiency (OMIM*613953) was found in a patient who presented with neonatal C. albicans skin infection and subsequent S. aureus dermatitis. He was homozygous for a nonsense mutation in the IL17RA gene, resulting in the creation of a premature stop codon at the position that would prevent production of the extracellular domain of IL17RA. Consistent with this mutation, IL17RA was not detected on the surface of the patient’s fibroblasts and PBMCs. In addition, when the patient’s fibroblasts were stimulated with various concentrations of IL17A and IL17F homo or heterodimers, the patient failed to respond by production of IL6 or GROα. Transfection of the patient’s fibroblasts with wild-type IL17RA restored responsiveness to IL17. The parents and siblings of the patients were heterozygous for the mutation and were healthy.
AD IL17F deficiency (OMIM*613956) was found in 5 of 17 kindreds who clinically all suffered with CMC and had a missense mutation of the IL17F gene. The mutated gene was also found in two asymptomatic family members suggesting incomplete clinical penetrance. This mutation replaced a highly conserved serine residue at position 65 with a leucine at a site predicted by computational analysis to be important for cytokine binding to its receptor. The mutation had no effect on the production of IL17F as a recombinant protein. Likewise, the mutant IL17F was able to form homodimers and heterodimers with IL17A. However, the mutant IL17F–containing homodimers or heterodimers had reduced binding to IL17RA on the surface of fibroblasts. IL17F homodimers also displayed much reduced induction of IL6 and GROα compared with wild-type IL17F homodimers, IL17A/mutant IL17F heterodimers, or IL17A homodimers. Thus, CMC in this kindred resulted from a mutation causing a hypomorphic, dominant-negative IL17F allele, which leads to production of IL17F homodimers and IL17A/IL17F heterodimers that have reduced binding to IL17RA and, hence, impaired bioactivity.
Patients were treated with courses of oral fluconazole with varying and temporary effect. Skin infections and dermatitis were treated with antibiotics.
These two mutations confirmed that human IL17 is essential for mucocutaneous immunity against C. albicans and definitely demonstrate the potential for disruption of the IL17 circuit to cause CMC (Fig. 6.8). Importantly, this puts into perspective recent efforts to target IL17 in the treatment of inflammatory disorders where potential adverse consequences, such as mucocutaneous infections must be considered [115].


Fig. 6.8
Inborn errors of IL17 immunity underlie chronic mucocutaneous candidiasis. Mutated proteins are marked in black
AR IL17RC deficiency (OMIM*616445) was recently identified as a cause of isolated CMC [199]. Three children from unrelated kindreds displayed AR IL17RC deficiency due to homozygous mutations for different nonsense alleles that prevented expression of IL17RC on the cell surface. The defect was complete, abolishing cellular responses to IL17A and IL17F homo and heterodimers although the response to IL17E was maintained. These experiments of nature indicated that human IL17RC is essential for mucocutaneous immunity to C. albicans but is otherwise largely redundant.
AR ACT1 deficiency (OMIM*615527) was identified in 2013 by the same group caused by biallelic missense mutations in the adaptor protein ACT1 (TRAF3IP2) located in the SEFIR region [33]. This mutation impaired the homotypic interaction of ACT1 with the IL17 receptor abolishing responses to IL17A and IL17F in fibroblasts as well as to IL17E in leukocytes. It did not affect interactions with other proteins (HSP90, HSP70 or CD40) which are mediated by other domains of the ACT1 protein. Healthy individuals who were homozygous for a common missense polymorphism located in a different ACT1 domain (D10N) had impaired but not absent responses to IL17 cytokines. These findings demonstrated that human IL17A and IL17F depend on ACT1 to mediate protective mucocutaneous immunity although other ACT1-dependent IL17 cytokines seem to be largely redundant in host defense.
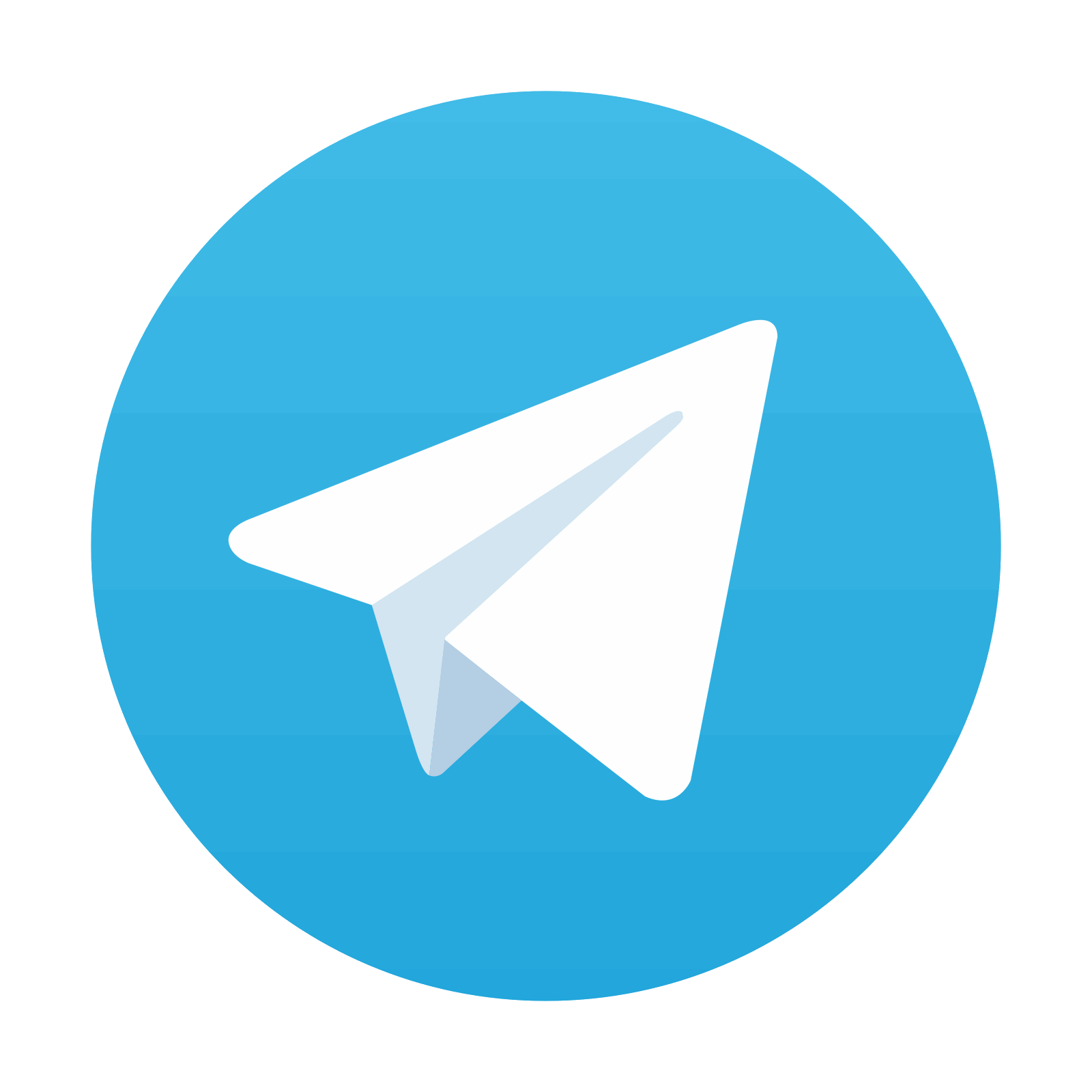
Stay updated, free articles. Join our Telegram channel

Full access? Get Clinical Tree
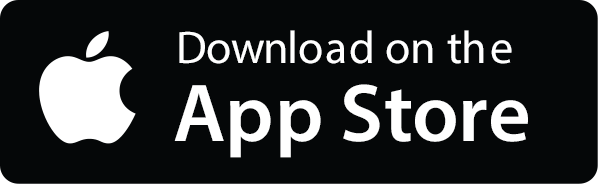
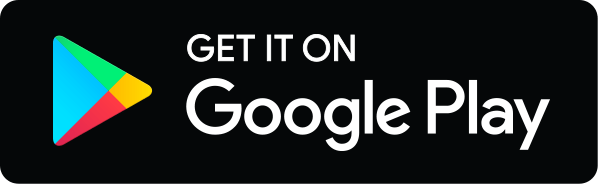