and Fatemeh Ghane Sharbaf2
(1)
Department of Pediatrics Section of Nephrology, Rush University Medical Center, Chicago, IL, USA
(2)
Department of Pediatrics Section of Nephrology, Mashhad University of Medical Sciences, Mashhad, Iran
Principle of Continuous Renal Replacement Therapy
Dialytic intervention for infants and children with acute kidney injury (AKI) can take many forms [1–12]. Whether patients are treated by intermittent hemodialysis, peritoneal dialysis, or continuous renal replacement therapy (CRRT) depends on specific patient characteristics [13]. Modality choice is also determined by a variety of factors, including provider preference, available institutional resources, dialytic goals, and the specific advantages or disadvantages of each modality [14–25]. Our approach to AKI has benefited from the derivation and generally accepted defining criteria put forth by the Acute Dialysis Quality Initiative (ADQI) group [18]. These are known as the risk, injury, failure, loss, and end-stage renal disease (RIFLE) criteria. A modified pediatrics’ RIFLE (pRIFLE) criteria have recently been validated. Common defining criteria will allow comparative investigation into therapeutic benefits of different dialytic interventions [2].
While this is an extremely important development in our approach to AKI , several fundamental questions remain. Of these, arguably, the most important are “When and what type of dialytic modality should be used in the treatment of pediatric AKI?”
The choice of an appropriate modality for AKI depends on the clinical status of the patient as well as the dialytic indication. Clinically, several important patient conditions require attention. Initial patient assessment should focus on whether multiple organ systems are involved and to what extent. A patient with AKI solely will predictably have a better potential outcome than those with multisystem failure. If AKI is the only morbidity associated with the patient, then it is important that quantification of urine output (if present) be acknowledged. This will help determine the patient’s ability to handle fluid and solute load. Classically defined indicators for RRT initiation in the setting of AKI are extrapolations of those we have commonly used for end-stage renal disease (ESRD) and include metabolic/electrolyte imbalance, uremia with bleeding and/or encephalopathy, hypervolemia with pulmonary edema/respiratory failure, intoxications, inborn errors of metabolism, and nutritional support. While these may be recognized indicators, to date there has been no adequate definition of what “timing of initiation” means. The decision to initiate RRT may be affected by strongly held physician beliefs (in terms of indications), patient characteristics (including age/size, illness acuity, and comorbidities), and organizational characteristics (including resource availability, type of institution, type of ICU, type of provider, and perceived cost of therapy). All these factors will ultimately determine the appropriateness and availability of modality choice.
Specific indications for RRT typically include the need for ultrafiltration (i.e., fluid removal), either for symptomatic volume overload or to make space for nutrition, medications, and blood product support and/or solute removal (i.e., urea, potassium), either for uremia or for removal of a dialyzable toxin [5, 24, 26–41]. In addition to these clinical variables, the use of specific modalities in terms of need for nutritional support to aid in patient recovery from AKI or its underlying cause must be considered. The rapid removal of solute (urea) and correction of electrolyte abnormalities (particularly elevated levels of potassium) are of extreme importance in the setting of AKI. While peritoneal dialysis (PD), intermittent hemodialysis (IHD), and CRRT can rapidly correct hyperkalemia and uremia, IHD and CRRT provide greater clearance of higher-molecular-weight solutes than PD [13, 14, 40, 42–44]. The rapidity of solute generation and its particular urgency for removal, as in tumor lysis syndrome, inborn error of metabolism, hyperammonemia, symptomatic hyperkalemia, or ingestion of dialyzable toxins, require IHD or CRRT rather than PD, whereas mild uremia can be treated with any of the modalities. Urgent fluid removal required in patients with pulmonary edema and difficulty in ventilating may only be achieved by IHD or CRRT. Mild volume overload can be treated with any modality. For example, a hemodynamically unstable patient with overwhelming sepsis, fluid overload, respiratory compromise, pressor requirements, and renal involvement may necessitate initiation of CRRT for close fluid status control, whereas a postoperative cardiac patient with minimal fluid overload and hemodynamic instability may be better served by PD. The physical characteristics of the type of solute to be removed (e.g., molecular size, percentage of protein binding) may determine the need for IHD versus PD versus CRRT (CVVH vs. CVVHD). The metabolic status of the patient may also reflect the type of dialytic solution required (Table 3.1). [5, 23, 24, 26–41, 47].
Table 3.1
Mechanisms of solute removal: intermittent hemodialysis versus continuous renal replacement therapya
Solutes |
Intermittent hemodialysis |
CRRT |
---|---|---|
Small solutes (MW < 300) |
Diffusionb |
Diffusionc (CVVHD)
Convection (CVVH) |
Middle molecules (MW 500–5000) |
Diffusion
Convection |
Convection
Diffusion |
Large molecules (MW 5000–50,000) |
Convection
Adsorption |
Convection
Adsorption |
Large proteins (MW > 50,000) |
Convection |
Convection |
The physical condition of a patient in terms of underlying disease process, size, previous surgical procedures, and overall stability will often dictate choice of modality. Contraindications to PD may include diaphragmatic hernia, recent intra-abdominal surgery, intra-abdominal sepsis, lack of an adequate peritoneal surface, or intra-abdominal malignancy. Also, in AKI secondary to HUS, gut involvement may be severe enough to preclude PD, as may necrotizing enterocolitis in a neonate. Severe hypotension may prohibit the use of IHD. A patient’s size may prevent successful vascular access or even temporary PD. Indeed, in our smallest neonates, vascular access may not be achievable with double-lumen catheters in the neck or groin, and single lumen 5 Fr. catheters may need to be placed in the umbilical vessels. In the same neonatal patient, even temporary PD may be impossible when automated machinery is used, due to tubing “dead space.” Such considerations must be taken into account in determining the feasibility of dialysis delivery in our smallest patients. The presence of a coagulopathy may impact on performance of IHD or CRRT or the ability to establish vascular access for either modality.
CRRT is a mode of renal replacement therapy that is used for hemodynamic instable, fluid overload, and septic patients complicated by AKI in the critical care/intensive care unit setting [16, 22, 27, 30, 36, 45, 48–55]. CRRT provides a slow, gentle treatment of AKI and fluid removal much like the native kidney (ultrafiltration up to 120 mL/h) and is generally well tolerated by critically ill, hemodynamically unstable patients. The CRRT is intended to substitute for impaired renal function over an extended period of time and applied for 24 h a day. It provides slower solute clearance per unit time as compared with intermittent hemodialysis therapies, but over 24 h may even exceed clearances with intermittent hemodialysis.
Peritoneal or intermittent hemodialysis is not suitable for patients suffering from sepsis because it cannot remove the inflammatory mediators (large-molecular-weight particles). In addition, critically ill neonates and infants with recent abdominal surgery and compromised cardiopulmonary function often are unable to tolerate peritoneal therapy. Likewise, hemodynamically unstable children may not tolerate rapid ultrafiltration with conventional hemodialysis therapy [3, 10–12, 22, 26, 28, 40, 43, 45, 52–54, 56–64].
CRRT differs considerably from intermittent hemodialysis , relying heavily upon continuous ultrafiltration of plasma water. It has the potential for removal of large quantities of larger-molecular-weight drugs, such as glycopeptide antibiotics, from plasma [13]. Moreover, control of anemia, acid–base balance, and fluid volume can be achieved easily and continuously maintained with CRRT. In addition, CRRT removes inflammatory mediators of sepsis such as TNF-alpha, interleukin, and complement [60]. CRRT is also used to allow other supportive measures, such as nutritional support. Feeding patients in critical care may require large amounts of fluid. CRRT offers a fluid balance mechanism, ultrafiltration, to assist with balancing fluid and to remove metabolic waste products [24, 33–35, 65–68].
CRRT is usually performed through a double-lumen venous hemodialysis catheter situated in a large vein (usually an internal jugular), an extracorporeal circuit, a hemofilter, a blood pump, and an effluent pump to establish a steady blood flow into the circuit either as hemofiltration (CVVH), hemodialysis (CVVHD), or a combination of the two: hemodiafiltration (CVVHDF) [1, 4, 5, 19, 29, 53, 57, 69–73].
CRRT technique may differ significantly according to the mechanism of solute transport, the type of membrane, the presence or absence of dialysate solution, and the type of vascular access. CVVH and CVVHD are the two most commonly used hemofiltration techniques for treatment of neonatal AKI [29, 74].
CVVH uses a predominantly hydrostatic pressure gradient (convection) to pump solute across a filter membrane to achieve clearance.
CVVHD , on the other hand, uses diffusion across a membrane to effect clearance of solute. This is achieved by generating a continuous concentration gradient using countercurrent flow of plasma and dialysate fluid, between which equilibration occurs. However, the filtration flow rates in CVVHD are relatively low because its effectiveness in drug clearance is mainly achieved through diffusion. As a result, drugs with a higher molecular weight are removed more slowly and show a lower clearance than drugs with smaller molecular weight. CVVHDF uses a combination of the connective (ultrafiltration) and diffusion (dialysis) techniques to remove solutes.
The impact of CRRT on drug removal is variable depending on the various techniques that are used in the management of AKI, the ultrafiltrate and dialysate flow rates, the filter, and the patients’ residual renal and non-renal drug clearance.
In general, drugs that are predominately removed by the normal kidneys require a dose reduction in patients with AKI. If CRRT is initiated, some of the drugs may be eliminated by CRRT. The extent of drug removal determines whether supplemental dosing is necessary during CRRT to avoid the drug underdosing (see sections “Drug Removal During CRRT: Basic Principles,” “Drug Dosing During CRRT,” and “Factors Influencing the Clearance of Drugs During CRRT”).
Advantages of Continuous Renal Replacement Therapy
CRRT has several theoretical advantages over intermittent blood purification techniques, including better hemodynamic tolerability, more efficient solute clearance, better control of intravascular volume, and better clearance of middle and large-molecular-weight substances.
Hypotension is one of the most common complications associated with intermittent hemodialysis, occurring in approximately 20–30 % of all treatments. Some of the causes are dialysis specific, such as excessive or rapid volume removal, changes in plasma osmolality, and autonomic dysfunction. In critically ill patients who may be hemodynamically unstable, it would be desirable to minimize this complication, as it may lead to further organ ischemia and injury. Several prospective and retrospective studies have demonstrated better hemodynamic stability associated with CRRT [13, 20, 42, 43, 53, 75]; however, this observation has not been validated in a randomized controlled trial.
Another advantage of CRRT is the improved efficiency of solute removal . Although the clearance rate of small solutes is slower per unit time with CRRT (17 mL/min vs. more than 160 mL/min with conventional hemodialysis), CRRT is continuously administered; therefore, urea clearance is more efficient after 48 h than with alternate-day intermittent hemodialysis. Clark et al. developed a computer model based on 20 critically ill patients to compare solute clearance in intermittent and continuous renal replacement therapies and found that for a 50 kg male, an average of four dialysis sessions/week would be required to achieve equivalent uremic control [17]. In patients with a weight greater than 90 kg, equivalent uremic control could not be achieved with intermittent therapies even if daily dialysis was prescribed.
Fluid management is often a difficult issue in ICU, where nutritional requirements (TPN) and the use of IV medications necessitate the administration of large amounts of fluid to critically ill patients. The inability to severely restrict fluid intake in ICU patients results in excessive volume overload, which may compromise tissue perfusion and has been associated with adverse outcomes [33, 34, 49]. Attempts to restrict fluid in this setting may additionally compromise adequate nutrition. The capacity to adjust fluid balance on an hourly basis, even in hemodynamically unstable patients, is largely responsible for the growing popularity of CRRT.
CRRT may also have an immunomodulatory effect. The rationale for the use of CRRT for the treatment of sepsis arises from the observed association between sepsis severity mortality rate and serum concentrations of various cytokines including TNF, IL1, IL6, and IL8. Most of these middle-molecular-weight molecules are water-soluble and are theoretically removable by hemofiltration-based plasma water purification [40, 60, 67]. At the present time the immunomodulatory effects of CRRT remain theoretical and have not been shown to affect outcome in human studies [65].
Despite its apparent advantages over intermittent therapies , superiority of CRRT with respect to mortality or recovery of renal function has not been demonstrated. In the largest randomized controlled trial to date (n = 166), intermittent hemodialysis was associated with significantly lower in-hospital (48 vs. 65 %) and ICU mortality (42 vs. 60 %). However, patients with hypotension were excluded from participating in the study, and there was a significant difference in severity scores between the treatment arms despite randomization [13, 23, 45, 76]. Two recently published meta-analyses compared intermittent with continuous renal replacement therapies in unselected critically ill patients [23, 76]. Both concluded that there was no difference in terms of renal recovery. However, while Kellum concluded that there was improved survival with CRRT [23], Tonelli found no survival benefit with either modality [76]. Moreover, the sample size required showing a 20 % mortality difference between intermittent hemodialysis and CRRT would be in excess of 1200 patients.
There are also significant cost implications associated with modality choice for treatment of ARF in the ICU setting. A study comparing CRRT to alternate-day IHD showed CRRT to be significantly more expensive, primarily because of the cost of CRRT fluid. Cost differences also depend on whether these procedures are performed by critical care nurses or by renal unit nurses and whether interunit charges are applied. Further studies are needed to define the subset of patients with ARF who benefit from this therapy.
Indications for CRRT
The precise decision of CRRT initiation is usually a matter of clinical judgment and depends upon patient hemodynamic stability, the availability of vascular access, and supporting nursing staff and technical resources [5, 77–80]. Hemofiltration is usually indicated in children with anuric AKI complicated by edema, electrolytes abnormalities, catabolic patients with increased nutritional needs, patients with septic shock, inborn error of metabolism, diuretic unresponsive edema, poisoning, and hepatic coma.
Absolute indications for dialysis include symptoms or signs of uremia (e.g., changes in mental status, pericardial rub or effusion, uremic platelet dysfunction) and management of volume overload, hyperkalemia >6 mEq/L with ECG changes, or metabolic acidosis (pH < 7.15) that is refractory to medical therapy [27, 32, 45, 46].
A. Renal Causes [27]
1.
AKI with septicemia, fluid overload, cardiovascular instability, and pulmonary edema
2.
AKI with electrolytes and acid–base imbalance
3.
Oliguric AKI with high fluid requirements (nutrition, blood products)
CRRT is indicated in the pediatric population for hypervolemic anuric AKI, electrolyte abnormalities, catabolic patients with increased nutritional needs, patients with sepsis, poisoning, inborn errors of metabolism, diuretic unresponsive hypervolemia, and hepatic or drug-induced coma. Intoxicants amenable to hemofiltration are vancomycin, methanol, procainamide, thallium, lithium, methotrexate, and carbamazepine.
Severe sepsis and septic shock are the primary causes of multiple organ dysfunction syndrome including AKI. Variety of water-soluble mediators with pro- and anti-inflammatory activities such as INF, IL-6, IL-8, and IL-10 play a major role in the pathogenesis of septic shock leading to the activation of the complement cascade and coagulation pathways. Early CVVH/CVVHD interventions utilizing higher ultrafiltration rate greater than 35 mL/kg/h coupled with adsorption to a membrane with increasing pore size to enhance middle molecule have shown to improve removal of water-soluble sepsis mediators.
Practical Considerations for Pediatric CRRT
Peritoneal or intermittent hemodialysis dialysis is not suitable for patients suffering from sepsis because it cannot remove the inflammatory mediators (large-molecular-weight particles) [13, 15]. Furthermore, critically ill neonates and infants with recent abdominal surgery and compromised cardiopulmonary function often are unable to tolerate peritoneal therapy. Likewise, hemodynamically unstable children may not tolerate rapid ultrafiltration with conventional hemodialysis therapy.
CRRT provides a slow, gentle treatment of AKI and fluid removal much like the native kidney (ultrafiltration up to 120 mL/h) [1–3, 14, 20, 35, 52–55, 69, 71, 73, 81–83]. Moreover, control of azotemia, acid–base balance, and fluid volume can be achieved easily and continuously maintained with CRRT. CRRT provides a slow, gentle treatment of AKI and fluid removal much like the native kidney and is generally well tolerated by critically ill, hemodynamically unstable patients. In addition, CRRT removes inflammatory mediators of sepsis such as TNF-alpha, interleukin and complement. CRRT is also used to allow other supportive measures, such as nutritional support. Feeding patients in critical care may require large amounts of fluid. CRRT offers a fluid balance mechanism, ultrafiltration, to assist with balancing fluid and to remove metabolic waste products [6, 7, 9, 25, 40, 42–44, 47, 59, 61, 63, 75, 78, 79, 84–87].
Timing of CRRT
There is no commonly accepted definition for the timing of initiating renal replacement therapy in AKI, although several observational and retrospective analyses have suggested improved survival with earlier initiation of renal support [70, 88].
It has been suggested that patient outcome can be improved by early or more intensive dialysis to keep the BUN under 80–100 mg/dL (29–36 mmol/L). However, because the BUN may reflect many factors other than the timing of initiation, no absolute value for BUN or creatinine should be used to determine when to initiate dialysis.
Only one randomized controlled trial has looked at the effect of timing of initiation of renal replacement therapy on outcome [56]. Bouman et al. randomized 106 critically ill patients with ARF to early versus late initiation of dialysis. Early initiation was started within 12 h of patients meeting the following criteria: low urine output (<30 cc/h) × 6 h refractory to optimization of hemodynamics and diuretics and creatinine clearance of <20 mL/min. The late initiation group was started on dialysis when the classic indications for dialysis were met (volume overload, hyperkalemia, urea greater than 40 mmol/L). There was no significant difference between the groups in terms of ICU or hospital mortality and no difference with respect to recovery of renal function. The results of this study must be interpreted with some caution, however, as the study was underpowered to detect a clinically significant difference and the mortality rate in all treatment groups was very low.
The dialysis working group consensus statement on renal replacement therapy makes no recommendations on the timing of initiation of renal replacement therapy beyond those defined by the conventional criteria that apply to chronic renal failure [18].
Kinetic Modeling of Solute Clearance
At the present time, a urea kinetic model (UKM) -based calculation of plasma solute clearance is the most common method measurement of dialysis dosage with both intermittent and continuous forms of dialysis, although it is not entirely clear that the calculated clearance values can be directly compared. This calculation is called Kt/V, where K is clearance, t is duration of dialysis, and V is the volume of distribution of urea.
CVVH sieving coefficient for sodium and potassium are 0.99, chloride 1.05, bicarbonate 1.12, BUN 1.05, creatinine 1.02, glucose 1.04, calcium 0.64, magnesium 0.90, phosphate 1.04, albumin 0.01, and total protein 0.02.
The limitation of this method rests in the observation that critically ill patients with ARF are frequently catabolic and have highly variable fluid volumes; both conditions violate several of the assumptions implicit in urea kinetic modeling.




CRRT Dosing Recommendation
Similarly, there is no consensus as to what the minimal dialysis dose should be in patients with AKI. It seems reasonable to suggest a minimum Kt/V of 1.2 should be delivered at least three times a week in patients with AKI. However, several recent studies support the belief that more intensive dialysis may be beneficial in this patient group. A randomized dose-intensity study of CVVH in 425 critically ill patients demonstrated a significant decrease in patient mortality when ultrafiltration rates of 35 mL/kg/h were used as compared with 20 mL/kg/h [7, 74, 86, 89]. A randomized trial of intermittent hemodialysis comparing daily with alternate-day dialysis showed a reduction in mortality from 46 to 28 % (p < 0.05). Unfortunately the delivered dialysis dose in the alternative day group as measured by weekly Kt/V was less than 3.6, the minimally acceptable dose in chronic dialysis patients, thus the issue of minimal adequate dose remains unresolved.
CRRT Modality Choice
CRRT solute clearance is dependent on the molecular size of the solute, the pore size of the semipermeable membrane, and the type of CRRT modality. The higher the ultrafiltration rate, the greater is the solute clearance.
CRRT can be performed with ultrafiltration (SCUF), hemofiltration (CVVH), or hemodialysis (CVVHD) or a combination of both techniques (CVVHDF). Of these the CVVH and CVVHD are often used in children requiring CRRT [1, 3, 7, 8, 23, 25, 42, 56, 63, 69, 73, 75–77, 87, 90, 91].
Arteriovenous modes of CRRT using the patient’s own cardiac output to drive blood flow through the dialysis circuit are no longer used due to the development of external circuit pump and the high access complications rate [1, 3, 55].
Small-molecular-weight substances, such as urea, is cleared equally by both diffusive and connective modes (CVVH, CVVHD, CVVHDF), but for large-molecular-weight substances such as proteins, vancomycin, or cytokine clearance, the convection mode (CVVH) is superior over the diffusive mode of therapy [5, 26, 28, 39, 40, 47, 60, 74, 92]. In septic and highly catabolic patients, there is an advantage of convective over diffusive clearance [39, 40, 74]. There is always a greater risk of underdosing medications and sieving nutrition from patients with the convection than diffusion mode of therapy.
Slow Continuous Ultrafiltration
Slow continuous ultrafiltration (SCUF ) is the simplest CRRT modality that aims for excess fluid volume removal without administration of a replacement solution. Mechanism of water transport in SCUF is ultrafiltration. Primary indication for SCUF is management of fluid overload without metabolic imbalance. The amount of fluid and its composition in the effluent bag are the same as the amount of fluid removed from the patient.
In SCUF blood enters the extracorporeal circuit through an access line, passes through the hemofilter, and returns to the patient circulation via the return line [4, 5, 39, 74]. Here is the schematic for SCUF. Notice the blood pump, effluent pump, and the PBP which are accessible (Fig. 3.1).
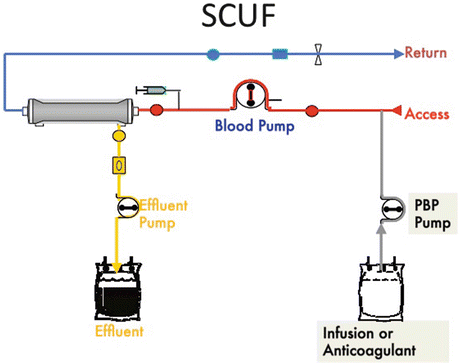
Fig. 3.1
Slow continuous ultrafiltration (SCUF) (Gambro Training Manual, used with permission)
As the blood passes through the filter, ultrafiltration takes place and effluent collects in the effluent bag. Effluent is any fluid that exits the hemofilter and is delivered to a waste bag [17]. Pumps control blood flow and fluid removal rates. The maximum patient fluid removal rate is 200 mL/h.
Small molecules easily pass through a membrane driven by diffusion and convection. Middle- and large-size molecules are cleared primarily by convection. Semipermeable membrane removes solutes with a molecular weight of up to 50,000 Da. Plasma proteins or substances highly protein-bound will not be cleared.
The sole objective of SCUF therapy is to provide fluid balance in the patient by removing plasma water, up to 2000 mL/h, through ultrafiltration across a semipermeable membrane. SCUF requires a blood and an effluent pump. SCUF is useful for safe and large fluid removal in patients with congestive heart failure who is fluid overloaded and does not respond to diuretics but does not require solute balance. Safe fluid removal up to 2 L/h can be achieved.
The patient fluid removal rate may be set to balance the effects of infusions to the patient, such as parenteral nutrition or drug administration, or to correct a fluid overload condition. Blood flow rate may be set between 18 and 180 mL/min. The effluent dose is recommended at 35 mL/kg/h. This type of ultrafiltration utilizes no replacement or countercurrent replacement solution (dialysate), but only removes fluid. The patient fluid removal rate is the net amount that the system removes from the patient each hour. SCUF is usually performed at ultrafiltration rates below 8 mL/min. Although it is very rare to have a patient who can tolerate high fluid removal rates, the SCUF system can remove up to 2 L of fluid per hour from the patient.
This therapy might be used for a patient who is simply fluid overloaded but does not require solute balance, for example, a patient with congestive heart failure admitted with fluid overload. They do not respond well to their diuretics. If their kidneys cannot excrete excess fluid, SCUF can do the job.
Continuous Veno-venous Hemofiltration (CVVH)
CVVH is a highly effec tive method of solute removal and is indicated for uremia, severe metabolic acidosis, or electrolyte imbalance with or without fluid overload. CVVH is particularly efficient at removing small and large molecules (e.g., B12, TNF) via convection utilizing a pre- and/or post-filter replacement solution at about 35 mL/kg/h. Solutes can be removed in large quantities while easily maintaining a net zero or even a positive fluid balance in the patient. The amount of fluid in the effluent bag is equal to the amount of fluid removed from the patient plus the volume of replacement fluids administered. No dialysis solution is used [1, 5, 28, 39, 49, 56, 72, 74, 93].
CVVH adds use of pumped replacement fluids, either pre- or post-filter, to enhance middle molecule clearance by convection [17]. The maximum patient fluid removal rate is 1000 mL/h.
Here is the schematic for CVVH (Fig. 3.2). Notice that all pumps are accessible.
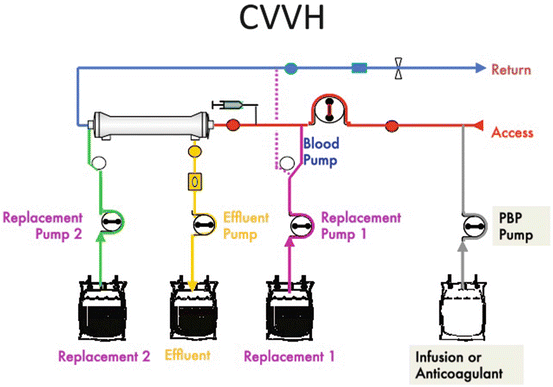
Fig. 3.2
Continuous veno-venous hemofiltration (CVVH) (Gambro Training Manual, used with permission)
Replacement solutions in CVVH are infused into the blood circuit using replacement pump 1 through the purple line of Prismaflex set and/or the replacement pump 2 through the green line of the Prismaflex set. Replacement pump 1 infuses solution pre- or post-filter, and pump 2 infuses fluid post-filter only.
Primary therapeutic goal of CVVH is water and solute removal across a semipermeable membrane to provide fluid balance as well as control electrolyte balance. Continuous hemofiltration with the aid of a blood pump provides solute removal by convection. A replacement solution is required to drive convection [28, 93]. This type of convection utilizes no countercurrent dialysate solution (Fig. 3.2). It offers high volume ultrafiltration using replacement fluid, which can be administered pre-filter (Fig. 3.3) or post-filter (Fig. 3.4). By reducing the HCT at the blood inlet, pre-dilution is believed to reduce clotting. Post-dilution will require less replacement fluid to achieve a given clearance (dose). A combination of pre-dilution, for example, by the PBP pump, and post-dilution by the replacement pump would provide benefit in several areas and provide flexibility required to treat a given patient. Pre-dilution also means a loss between 15 and 35 % of clearance (dose), depending on the flow rates. The pump guarantees adequate blood flow to maintain required UF rates [17]. Venous blood access is usually femoral, jugular, or subclavian using a double-lumen cannula [94–96].
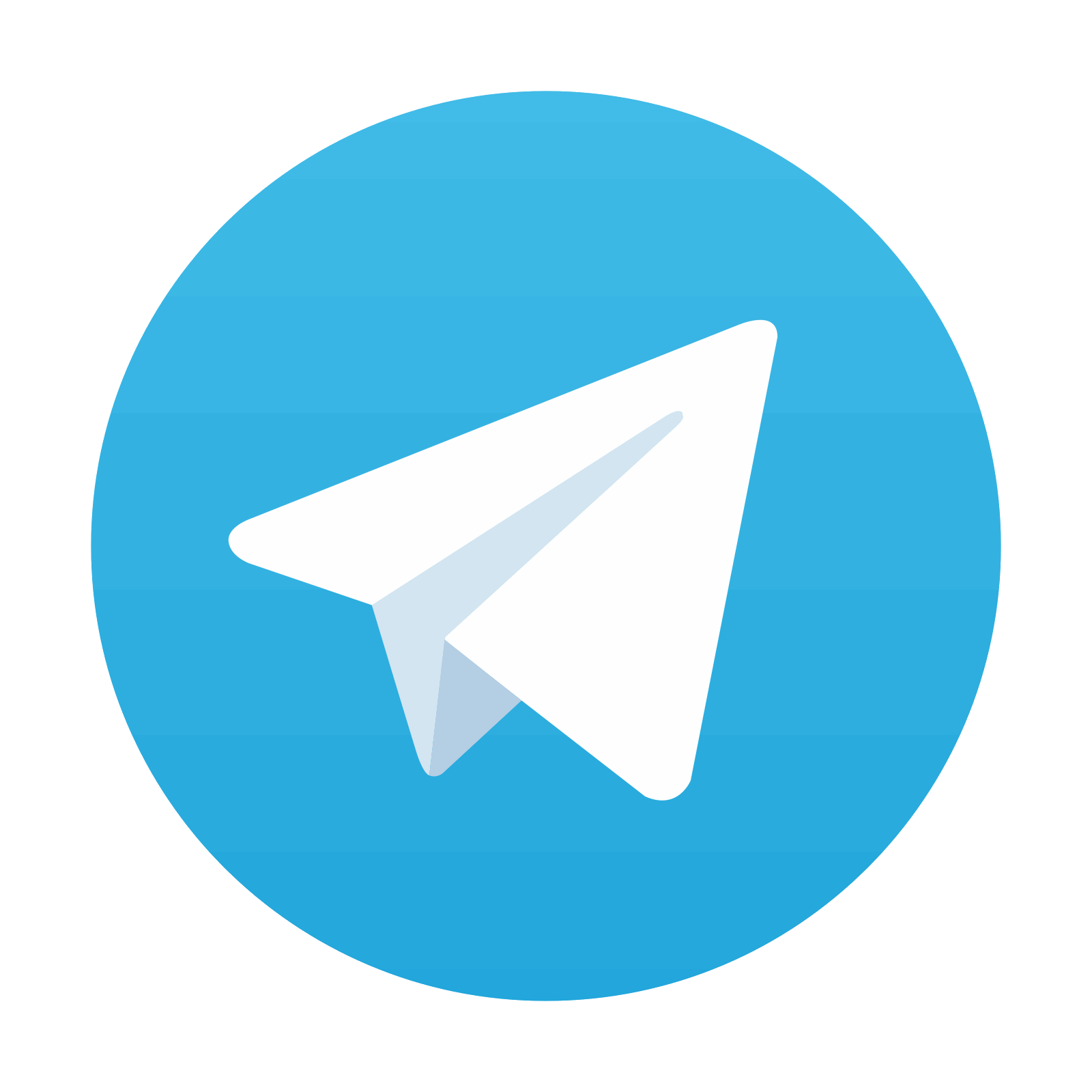
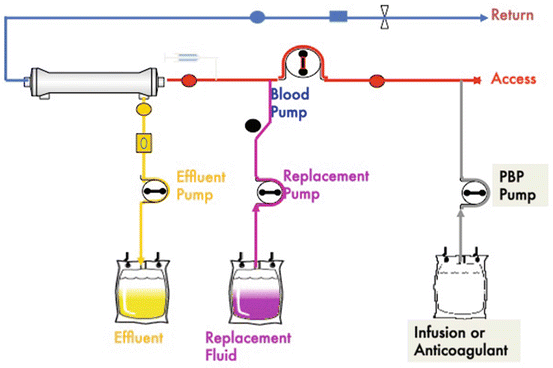
Fig. 3.3
Pre-dilution replacement solution
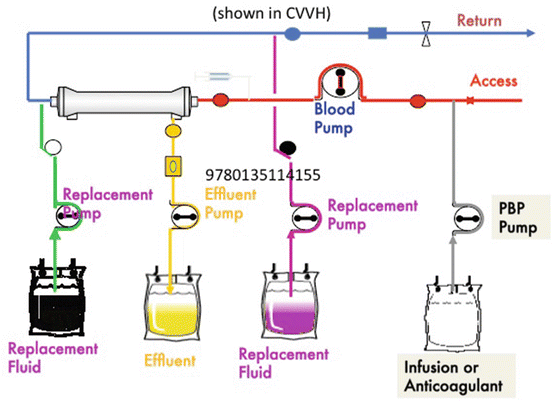
Only gold members can continue reading. Log In or Register to continue
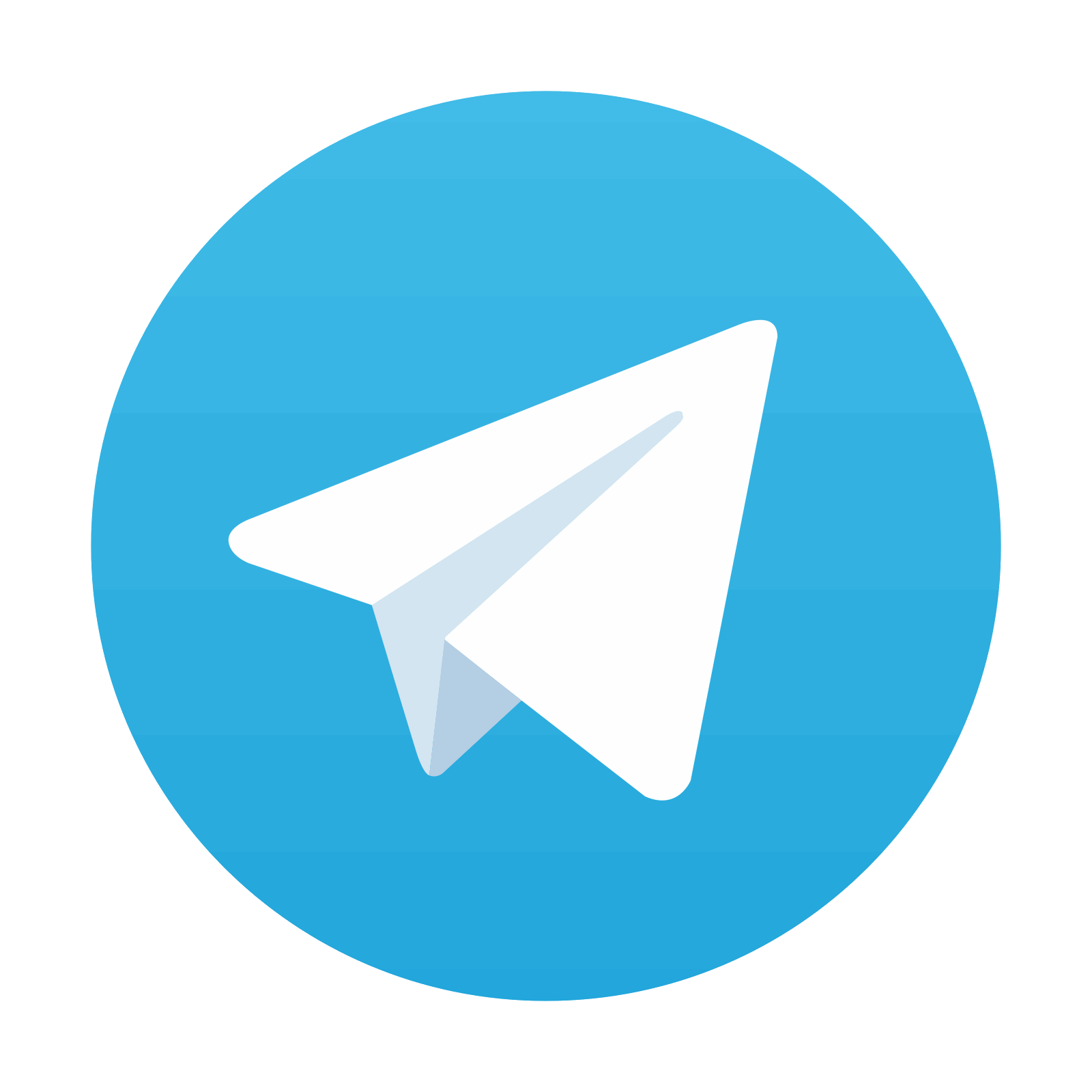
Stay updated, free articles. Join our Telegram channel

Full access? Get Clinical Tree
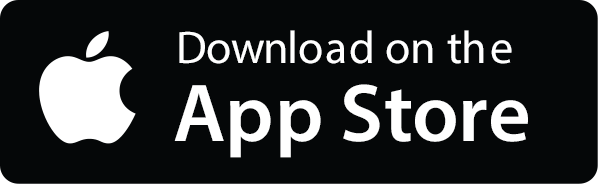
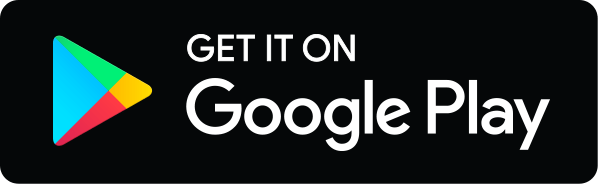