Fig. 25.1
Genetic mechanisms of congenital hyperinsulinism (see text for details)
Molecular genetic abnormalities have been identified in nine different genes which cause CHI: these include inactivating mutation of the KATP-channel genes (ABCC8 and KCNJ11), HNF4A, HNF1A, HADH, and UCP2 or activating mutations of GLUD1, GCK, and SLC16A1 [6, 7]. Mutations in ABCC8 and KCNJ11 genes are estimated to account for 40–45 % of all cases of CHI, while mutations in the remaining genes are identified in approximately 5–10 % of cases. The genetic etiology for the remaining 45–50 % of patients remains unknown.
25.2.1 KATP-Channel Mutations (ABCC8 and KCNJ11)
Inactivating mutations in the ABCC8 and KCNJ11 genes, which encode the SUR1 and Kir6.2 subunits of the KATP-channel, are the most frequent cause of CHI. Mutations in these genes impair the ability of MgADP to stimulate channel activity or affect the expression of the KATP-channels at the surface membrane, resulting in continuous depolarization and inappropriate insulin secretion. Many of these patients show a poor response to diazoxide therapy, which is evident considering the target of the drugs is the KATP-channel. The mode of inheritance of the KATP-channel mutations correlates with the histological type: the identification of two recessive mutations indicates a diffuse disease, whereas, when a single paternally inherited mutation is discovered, a focal lesion is suspected. Indeed focal lesions result from paternal uniparental disomy of chromosome 11p15.5-11p15.1 within a single pancreatic cell. In close proximity with the KATP-channel genes at chromosome 11p15.1, a region at 11p15.5 harbors maternally expressed tumor suppressors (H19 and CDKN1c) and a paternally expressed growth factor gene (IGF2). When paternal uniparental disomy occurs as a somatic mutation during the development of the pancreas, that β-cell loses KATP-channel activity. At the same time, the tumor-suppressor activities of H19 and CDKN1C are lost, and the activity of IGF2 is doubled. This leads to a growth advantage for the abnormal β-cells and eventually to a formation of a focal lesion [6, 8]. There are a few reports describing dominant mutations of KATP-channel genes, in patients with CHI responsive to diazoxide therapy.
25.2.2 Glutamate Dehydrogenase (GLUD1)
Mutations in the GLUD1 gene, which encodes the enzyme glutamate dehydrogenase (GDH), are the most frequent cause of CHI after ABCC8/KCNJ11 mutations. CHI induced by these mutations is also known as the hyperinsulinism/hyperammonemia syndrome. The enzyme GDH, activated by leucine, catalyzes the oxidative deamination of glutamate to α-ketoglutarate and ammonia. Activating mutations in GLUD1 lead to an increased conversion of glutamate to α-ketoglutarate and heightened sensitivity to stimulation by leucine. In β-cells, α-ketoglutarate enters the citric cycle and leads to an increased ATP/ADP ratio, the closure of KATP-channels and, eventually to an increased insulin secretion [9]. Inheritance is usually autosomal dominant. Patients with GLUD1 mutations usually present a milder form of CHI, with associated hyperammonemia. Hypoglycemia is rapidly precipitated by protein-rich meals. This type of CHI is usually diazoxide responsive, but patients also require a low-protein diet.
25.2.3 Hepatocyte Nuclear Factors (HNF4A and HNF1A)
Hepatocyte nuclear factor 4α (HNF4α), encoded by the HNF4A gene, is a transcription factor critical for liver development and hepatocyte-specific gene expression. In the pancreatic β-cell, HNF4α regulates several genes involved in glucose-stimulated insulin secretion. Heterozygous loss-of-function mutations in this gene have been shown to account for 5 % of cases of diazoxide-responsive hyperinsulinism [10]. The mechanism is currently unknown. Mutations in HNF4A cause also maturity-onset diabetes of the young (MODY1).
Mutations in HNF1A gene have been identified in macrosomic newborns presenting with diazoxide-responsive CHI. However, in later life, patients tend to shift from hypoglycemia to MODY3. The exact mechanism still remains unknown [6].
25.2.4 Glucokinase (GCK)
Congenital hyperinsulinism occurs when a heterozygous-activating mutation (inherited in autosomal dominant manner) in the GCK gene produce an enzyme with an increased affinity for glucose, resulting in the inappropriate insulin secretion at low blood glucose concentration. The age of presentation can vary widely from infancy to adulthood. Patients who require intervention are often responsive to diazoxide therapy, although unresponsive forms have also been described.
25.2.5 Hydroxyacyl-Coenzyme A Dehydrogenase (HADH)
Hydroxyacyl-coenzyme A dehydrogenase (HADH) catalyzes the penultimate step in fatty acid β-oxidation in the mitochondria and is highly expressed in the pancreatic β-cells. Recessively inherited mutations in the HADH gene have been reported, up to now, only in 10 patients, and the mechanism that leads to the unregulated insulin secretion is still unclear [6].
25.2.6 Solute Carrier Family 16, Member 1 (SLC16A1)
Solute carrier family 16, member 1 (SLC16A1) gene encodes a transporter required for the intracellular transport of pyruvate and lactate. The SLC16A1 gene is not usually transcribed in pancreatic β-cells. Mutations that cause inappropriate transcription of this gene in the pancreatic β-cells make these cells sensitive to extracellular levels of lactate and pyruvate and cause exercise-induced hyperinsulinism (EIHI) [11]. In case of EIHI, lactate and pyruvate, produced during anaerobic exercise, enter into the β-cells and are metabolized increasing the ATP/ADP ratio and inducing insulin secretion. Patients are diazoxide responsive, but treatment is not always necessary, as hypoglycemic episodes may be prevented by avoiding strenuous exercise.
25.2.7 Uncoupling Protein 2 (UCP2)
The UCP2 gene encode for the mitochondrial uncoupling protein 2, which uncouples oxidative phosphorylation from ATP synthesis. Under normal condition, UCP2 reduces ATP synthesis and thus suppresses the glucose-stimulated insulin secretion. A loss-of-function mutation in the UCP2 gene was recently suggested as a candidate gene for CHI [12].
25.3 Histopathology
Congenital hyperinsulinism does not correspond to a single pathological entity: many different forms have been reported on the basis of specific genetic defects. Nevertheless, concerning the surgeon’s point of view, there are two main pathological subtypes: diffuse (50–70 % of patients) and focal (30–50 % of patients).
In the diffuse type of CHI, the pancreas appears normal both macro- and microscopically. Careful analysis reveals the presence of large β-cells with abnormally large nuclei (as observed in hyperactive endocrine cells). Immunostaining with a specific antibody to proinsulin shows a large Golgi apparatus, reflecting a very high proinsulin synthesis. However, labeling with insulin antibody is very weak, indicating low insulin storage, because of unregulated insulin release.
The focal CHI is a focal adenomatous hyperplasia, characterized by the presence of a small endocrine lesion (3–10 mm in diameter). Histologically this lesion corresponds to the confluence of hyperplastic but normally structured islets, with a large core of β-cells and a peripheral rim of endocrine non-β-cells. Within the focal lesion, the β-cells are hyperactive, with enlarged cytoplasm and a large Golgi apparatus, rich in proinsulin, and with low insulin storage. Outside the focal lesion, the islets are small; their β-cells are resting, with a high storage of insulin and weak production of proinsulin [13].
25.4 Clinical Presentation
The majority of the babies with CHI are macrosomic at birth. Fetal hyperinsulinemia also accounts for the hypertrophic cardiomyopathy and hepatomegaly (increased storage of glucose as glycogen), commonly observed in patients with CHI. The clinical presentation is variable, and patients complain either mild unspecific symptoms of hypoglycemia (poor feeding, lethargy and irritability) or more severe symptoms, as apnea, seizures, or even coma. Seizures are generalized, tonic-clonic, and occur in half of the cases. Other common symptoms are tremors, hypotonia, cyanosis, and hypothermia. Older children and adolescent may present with sweating, confusion, and behavioral changes; in these patients, insulinoma should be considered as alternative diagnosis. Facies is typical with a high forehead, large nose with a short columella, smooth philtrum, and thin upper lip.
In most of cases, symptoms of hypoglycemia appear within 72 h from birth. Hypoglycemia is severe and permanent (both fasting and postprandial). Typically, newborns with CHI have greatly reduced fasting tolerance, and increased intravenous glucose infusion rate is required to maintain adequate blood glucose levels. If not promptly treated, these children are highly susceptible to develop neurological damage, psychomotor retardation, and even death.
25.5 Diagnosis
The main laboratory criteria for the diagnosis of CHI are inappropriately elevated plasma insulin (and/or C-peptide) in the presence of hypoglycemia, low plasma free fatty acids and ketone bodies, and inappropriate response to glucagon.
Some authors suggest that any detectable level of insulin during hypoglycemia is abnormal, whereas others propose different cutoffs; the most frequent value accepted for the diagnosis is serum insulin concentration >3μU/mL in the presence of blood glucose <50 mg/dL.
As insulin inhibits lipolysis, during hypoglycemia, beta-hydroxybutyrate level <1.5–2 mmol/L and free fatty acids <1–1.5 mmol/L are also used as diagnostic adjuncts.
When hypoglycemia is caused by a defect in glycogenolysis or in gluconeogenesis, the patient does not respond to intramuscular/intravenous injection of glucagon. An inappropriate glycemic response to glucagon, with an increase in blood glucose greater than 30 mg/dL at the time of hypoglycemia, is consistent with excess insulin action and is useful in order to confirm the diagnosis [14].
When diagnosis of CHI is confirmed, from a practical point of view, it is important to differentiate focal type from diffuse CHI. When a focal lesion is identified preoperatively, resection of the lesion or partial pancreatectomy can cure the patients without postoperative complications. In case of diffuse type, surgery should be reserved for patients with failure of medical management or poor compliance to therapy, because of the high risk either of persistent hypoglycemia or diabetes, after near-total pancreatectomy [15].
Although focal lesions detected with contrast-enhanced CT scan have been reported [16], they cannot usually be detected using conventional imaging modalities such as ultrasonography, computed tomography (CT), magnetic resonance imaging, and angiography.
Two interventional radiology tests have been used in the past. The arterial stimulation with venous sampling (ASVS) technique involves selective pancreatic angiographic stimulation using intra-arterial calcium and venous sampling. An immediate rise in insulin from stimulation in gastroduodenal artery suggests focal form in pancreatic head; if the test is positive in superior mesenteric artery, the lesion is localized in the uncinate process or in the neck; a rise in insulin from stimulation in splenic artery identifies a lesion in pancreatic body or tail. If the stimulation is effective in all three arteries, a diffuse CHI should be suspected. In transhepatic portal venous catheterization and selective sampling of pancreatic vein (THPVS), the pancreatic venous insulin levels are compared with simultaneous plasma levels of insulin and glucose [17]. Both ASVS and THPVS are technically challenging and have limited specificity and sensitivity, and these techniques have been replaced by 18-Fluorine-l-dihydroxyphenylalanine positron emission tomography (18F-DOPA-PET), usually combined with computed tomography with multiphase contrast media protocols (18F-DOPA-PET/CT). l-DOPA is absorbed by neuroendocrine cells and by pancreas islet cells and metabolized into dopamine. Beta cells of the pancreas have dopamine receptors, and the uptake of 18F-DOPA is very increased in foci with high insulin synthesis rates. 18F-DOPA-PET/CT detects focal lesions as small as 5 mm. This technique, described for the first time in 2003 [18], nowadays represents the most precise method used to differentiate between focal and diffuse form of congenital hyperinsulinism, with a sensitivity of 94 % combined with a specificity of 100 % [19].
25.6 Treatment
In CHI, it is vital to make a prompt diagnosis and to begin early management, as delay in treatment may cause brain damage and permanent neurodevelopmental disorders. Some factors as age, comorbidities, severity, duration, and frequency of hypoglycemic episodes could affect the neurological outcome.
The primary goals of therapy are to achieve normoglycemia and restore production of ketone bodies, inhibiting inappropriate insulin secretion.
The increase in glucose concentration can be achieved either by giving additional glucose or by administration of glycogenolytic and gluconeogenic hormones such as glucagon. Force-feeding must be prevented in order to avoid feeding refusal behavior or severe gastroesophageal reflux. If necessary, intravenous glucose infusion should be used in order to maintain blood glucose >60–70 mg/dL. In neonates with CHI glucose infusion rate necessary to maintain euglycemia is higher than in unaffected newborns (>6–8 mg/kg/min compared to a normal of 4–6 mg/kg/min).
On the other hand, for decreasing serum insulin, medications that inhibit insulin secretion (diazoxide, octreotide) and/or pancreatic resection will be required.
25.7 Medical Therapy
Diazoxide is a potent inhibitor of insulin secretion that binds the SUR1 subunit of the KATP-channel, activating it. This drug is considered the first-line drug for treatment of CHI but requires an intact KATP-channel. Therefore, children with diffuse disease due to inactivating mutations in ABCC8 and KCNJ11 and most patients with focal lesions are unresponsive to diazoxide. Diazoxide is daily administered orally in three divided doses, at 5–15 mg/kg/day. Most common side effects include hypertrichosis and salt and fluid retention, which could lead to severe complications, such as congestive hearth failure and reopening of the ductus arteriosus [20]. Other frequent side effects of the therapy with diazoxide are nausea, vomiting, and loss of appetite. When diazoxide is used in higher dose (20 mg/kg/day), it could lead to paradoxical hypoglycemia. In addiction to diazoxide, administration of a thiazide diuretic (chlorotiazide 7–10 mg/kg/day in two divided doses) is advised to prevent fluid retention and for its synergistic effect on the suppression of insulin secretion.
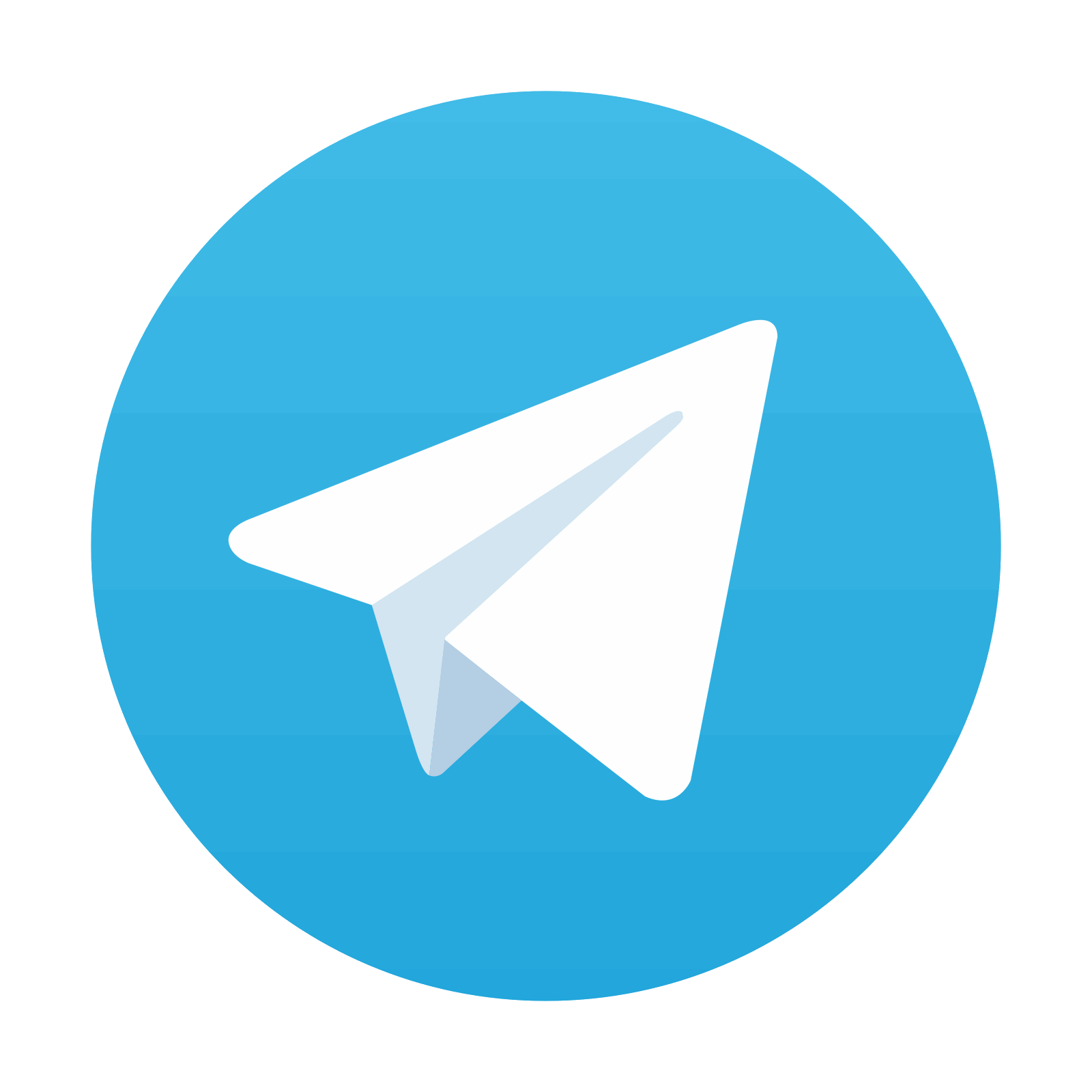
Stay updated, free articles. Join our Telegram channel

Full access? Get Clinical Tree
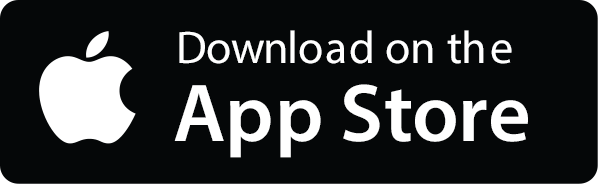
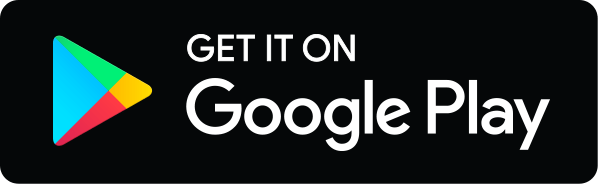