Figure 16.1 Irvine McQuarrie (1891–1961), chief of paediatrics, University of Minnesota.
Figure 16.2 Histology of HI. (a) Focal lesion. There is hyperplasia of beta cells (black stars), with exocrine (black arrowhead) and ductal (black arrow) components within the lesion. (b) Diffuse HI. Endocrine cells show nucleomegaly (black arrows) within an otherwise normal islet of Langerhans.
Focal HI consists of a single focus of adenomatous islet cell hyperplasia surrounded by normal pancreatic tissue. Focal lesions respect the limits of the pancreatic lobules (as opposed to insulinomas, which are well demarcated and do not respect the limits of pancreatic lobules). Beta cells within focal lesions have an enlarged cytoplasm and typically normal nuclei, although some can have nucleomegaly. They accumulate in clusters and are surrounded by nonbeta islet cells. The exocrine cells are pushed towards the periphery, but there are always some exocrine and canalicular cells intermixed within the endocrine cells [6]. Focal lesions vary in size from a few millimetres to centimetres or more and can be located on the surface or deep within the pancreas. In our series of more than 190 operated focal lesions, 45% were located in the pancreatic head, 25% in the neck or body, 15% in the tail and 15% in other locations, which included unusually large lesions that extended beyond a single pancreatic segment, and one case of lesions located in ectopic pancreatic tissue [7].
In diffuse HI, the appearance of the pancreas is normal except for one hallmark feature: beta cell nucleomegaly. Nucleomegaly is defined as nuclei that occupy an area three times larger than the nuclei of the adjacent nonbeta endocrine cells or four times larger than the nuclei of the adjacent acinar cells. The abnormal beta cells are distributed homogeneously throughout the organ in the majority of diffuse HI cases, and the total number of beta cells is not different than in organs from healthy age-matched individuals [8].
Of all patients with HI, 30%–40% have focal disease and 60%–70% have diffuse disease. In our experience of more than 360 HI patients since 1999 who underwent surgery (which represent about two-thirds of all HI patients treated at CHOP during that time period), 53% had focal disease and 47% had diffuse disease.
In addition to the focal and diffuse forms of HI, there are rare cases that do not fit in any of the two categories and are called atypical. Among those are cases of focal lesions that occupy a large segment of the pancreas, cases with remarkable endocrine hyperplasia in the context of Beckwith–Wiedemann syndrome and patients with features of diffuse HI restricted to a single area of the pancreas distributed in a mosaic pattern, which we term localised islet nuclear enlargement (LINE). Patients with atypical forms of HI are clinically heterogeneous and require a medical and surgical treatment plan that is individually crafted according to the severity of each case.
16.2.3 Pathogenesis and genetics
The liver accumulates glucose in the form of glycogen (glycogenogenesis) during the postprandial state, while the reverse happens during the fasting state (glycogenolysis). Multiple hormones and factors stimulate hepatic glycogenolysis to elevate plasma glucose concentration: glucocorticoids, glucagon, catecholamines, somatostatin and others. By comparison, insulin is the only endogenous hormone that reduces plasma glucose concentration, and it does it through two mechanisms: inhibition of hepatic glycogenolysis and stimulation of glucose uptake in peripheral tissues. When the plasma glucose concentration rises, glucose enters into the beta cell and is immediately metabolised, initiating a chain of events that will result in insulin secretion. The intracellular concentration of ATP increases (as does the ATP/ADP ratio); the ATP-dependent potassium channels (K-ATP channel) of the membrane become inactive and close; potassium accumulates on the inner surface of the membrane, depolarising it; and this then activates the voltage-dependent calcium channels, allowing calcium to enter the cell and accumulate in cytoplasm, triggering a calcium-dependent insulin exocytosis. When the K-ATP channel is defective due to loss-of-function genetic mutations, it remains closed at all times regardless of the plasma glucose level, generating a nonregulated, persistent insulin release that leads to hypoglycaemia. This is the most common pathophysiologic mechanism of HI. Insulin levels, however, are never strikingly elevated in HI.
Currently, about 50% of all patients with HI have a known genetic mutation. The most common mutations cause a loss of function in the K-ATP channel of the beta cell membrane. This channel is composed of the subunits SUR1 (a sulfonylurea receptor, the regulatory subunit) and Kir6.2 (the ion pore), which are encoded by two genes located next to each other in the p15.4 region of chromosome 11: ABCC8 and KCNJ11.
The diffuse form of HI occurs most frequently due to mutations of the SUR1/Kir6.2 complex inherited in a recessive manner. There are currently more than 200 known mutations in the ABCC8 and KCNJ11 genes, some of which are highly prevalent in certain populations [9,10]. Rare mutations of the ABCC8 and KCNJ11 genes are inherited in a dominant manner, and compound heterozygous mutations in the ABCC8/KCNJ11 genes have also been identified as a cause of diffuse HI, but the clinical presentation of these patients is milder than in patients with recessive disease [11,12]. Diffuse HI can also occur due to mutations in the genes of nine other enzymes and metabolic factors involved in the glucose metabolism: glucokinase (GK, 7p15.3–15.1), hexokinase (HK1, 10q22.1) glutamate dehydrogenase (GDH, 10q23.3, “hyperinsulinism-hyperammonemia syndrome”), short-chain hydroxyacyl-CoA dehydrogenase (SCHAD, 4q22–26), hepatocyte nuclear factor 4 alpha (HNF4A, 20q12–13.1), hepatocyte nuclear factor 1 alpha (HNF1A, 12q24.31), monocarboxylate transporter 1 (MCT1, 1p13.2– 12, “exercise-induced hyperinsulinism”), phosphoglucomutase (PGM1, 1p31.3) and the uncoupling protein 2 (UCP2, 11q13) [13–20].
The focal form of HI occurs through a ‘two-hit’ phenomenon: first, the individual inherits a mutation in the paternal allele of the SUR1/Kir6.2 complex, and second, the maternal 11p15 region containing the normal maternal allele is lost in a single pancreatic beta cell. The loss of the maternal 11p15 region is a completely random event that has no inheritable component. The result of this event is called loss of heterozygosity (only the allele from one progenitor is present). The beta cell affected not only will oversecrete insulin but also will develop an adenomatous hyperplastic proliferation due to an imbalance in a series of genes that control cell proliferation that are also contained in the 11p15 region and are subject to genomic imprinting. The 11p15 region contains the tumour suppressor gene H19 and the cell cycle regulator p57kip2. H19 (strongly imprinted and of exclusively maternal monoallelic expression) exerts an antagonistic effect on the insulin-like growth factor 2 (IGF2) expressed from the paternal allele. The imbalance between IGF2 and H19 is the reason for the adenomatous proliferation of the affected beta cells. The loss of the maternal allele in a focal lesion can be evidenced by genetic testing and immunohistochemistry [21].
When an infant is diagnosed with HI in the absence of a family history, the parents and the patient must undergo genetic testing, if available. In cases of diazoxide-responsive HI, the genetic testing only has a diagnostic purpose. On the other hand, in diazoxide-resistant cases (which theoretically have mutations in the SUR1/Kir6.2 complex), the genetic testing becomes more relevant since it can help differentiate between diffuse and focal HI, determine the need for imaging studies and provide prognostic information.
16.2.4 Diagnosis
The diagnosis of HI is established through a series of simple blood tests. The following three metabolic criteria must be present to confirm HI:
1. Fasting and postprandial hypoglycaemia with unsuppressed HI (neonatal hypoglycaemia is defined as a glucose plasma concentration of <50 mg/dL [SI ≈ <2.7 mmol/L] after the first 24 h of life with a plasma insulin concentration of >36 pmol/L)
2. Suppression of lipolysis and suppression of ketogenesis at the time of the hypoglycaemia (lipolysis and hepatic ketogenesis are a normal physiologic response to hypoglycaemia and are physiologically inhibited by insulin)
3. Positive glycaemic response to a dose of glucagon, a direct insulin antagonist (glucose must increase by 30–50 mg/dL [SI ≈ 1.6–2.7 mmol/L] after 0.25–1 mg of intravenous glucagon)
These criteria must be present for a prolonged period of time and outside certain clinical circumstances, such as perinatal stress and sepsis.
16.2.5 Therapeutic classification
The mainstay drug in the treatment of HI is diazoxide, which inhibits insulin secretion by activating the K-ATP channel. Diazoxide binds to the SUR1 subunit of the K-ATP channel, but in order to be effective, both subunits must be structurally and functionally normal. From a treatment standpoint, HI is divided into two groups: diazoxide responsive and diazoxide resistant. Since the most common cause of HI is a mutated SUR1/Kir6.2 gene complex, the majority of HI patients do not respond to diazoxide. The ones that do respond are those with mutations in the GK, GDH, SCHAD and other HI-related genes. In our experience with more than 500 HI patients, about one-third of them were diazoxide responsive and two-thirds were diazoxide resistant, and these generally require surgery.
16.2.6 Prenatal diagnosis and counselling
Prenatal screening of all known mutations of all HI-related genes in the general population is not justified due to the very low incidence of the disease. On the other hand, prenatal diagnosis in families with affected probands is possible and justified because it allows immediate postnatal management [22]. The genetic mutations that cause diffuse HI respond to the Mendelian inheritance laws, with the exception of the de novo mutations. While the inheritance of an abnormal ABCC8/KCNJ11 gene from paternal origin does respond to Mendelian laws, the occurrence of focal disease in subsequent siblings of an affected individual is unpredictable given the fact that the second event in the pathogenesis of the disease (the loss of the normal maternal allele) occurs as a random event in a somatic cell. The likelihood of this occurrence is extremely low, but it has been reported [23].
16.3.1 Medical management
The priority in the treatment of infants with HI is to protect the brain by providing enough glucose to maintain normoglycaemia. This is achieved by a combination of high-concentration intravenous glucose infusion and frequent enteral feeds. The required glucose infusion rate (GIR), calculated as percent dextrose × intravenous rate × 0.169 per weight in kilograms, can be as high as 30 mg/kg/min, which is more than three times higher than the physiological hepatic glucose release during fasting periods in newborns. Along with the direct glucose administration, hyperglycaemic drugs must be initiated. The first-line drug is diazoxide, an agonist of the K-ATP channel. Diazoxide is not effective in patients with recessively inherited mutations in the ABCC8/KCNJ11 complex, but it can be partially effective in patients with dominant and compound heterozygous mutations in the ABCC8/KCNJ11 complex [11,12]. Diazoxide is effective in patients with mutations in the other HI-related genes of dominant inheritance known to date. After 5 consecutive days of diazoxide administration, the response is evaluated by a fasting test off intravenous glucose and other hyperglycaemic medications. Infants who can maintain a plasma glucose level of >70 mg/dL (SI ≈ >3.9 mmol/L) for at least 12 h are considered diazoxide responsive. For these patients, a regimen of frequent feedings is established and they are discharged home on long-term diazoxide treatment (Figure 16.2). The adverse effects of diazoxide are sodium and fluid retention (which can be controlled with diuretics) and hypertrichosis (which requires no treatment). Patients whose plasma glucose level falls below 70 mg/dL within the first hours of the fasting test are considered diazoxide resistant and are presumed to have recessively inherited HI. Diazoxide is discontinued, the intravenous glucose infusion is reestablished immediately and preoperative planning starts. Infants who are able to maintain the plasma glucose level above 70 mg/dL for several hours but do not reach the 12-hour mark are considered to have a partial response to diazoxide. These patients are managed with a combination of diazoxide and frequent or even continuous feedings.
In patients with diazoxide-resistant HI, alternative drugs can be tried in order to maintain euglycaemia, but they are mainly used as stabilising agents prior to surgical intervention or to reverse profound hypoglycaemic episodes. Octreotide
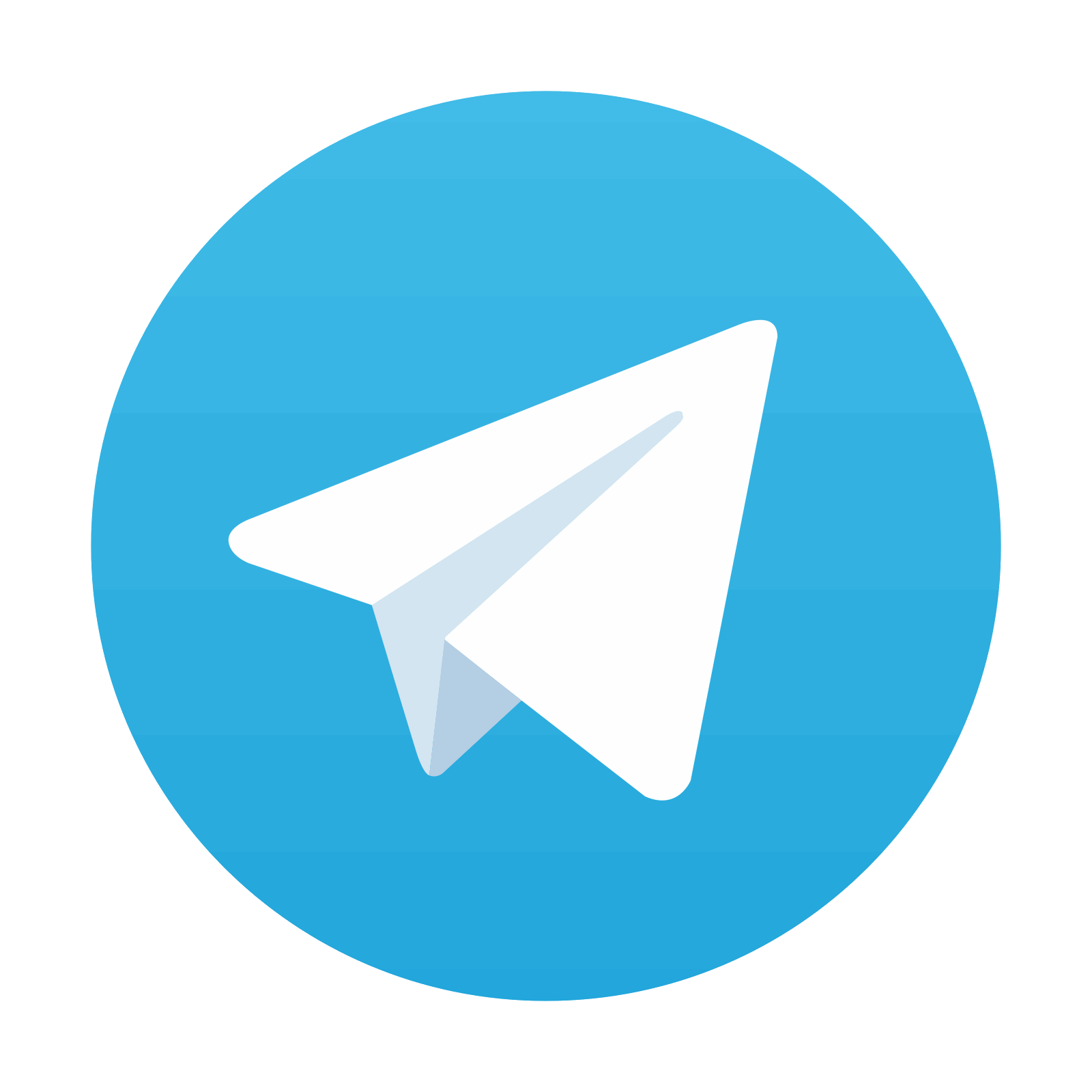
Stay updated, free articles. Join our Telegram channel

Full access? Get Clinical Tree
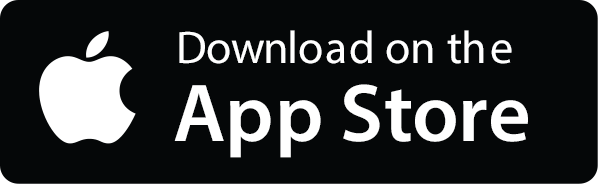
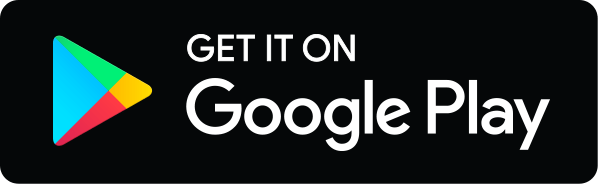