, Per H. Nilsson3, 4, Tom Eirik Mollnes3, 4, 5, 6, 7, Dirk Roos8 and Kathleen E. Sullivan9
(1)
Department of Cellular and Molecular Nutrition, School of Nutrition and Dietetics, Tehran University of Medical Sciences, Tehran, Iran
(2)
Dietitians and Nutrition Experts Team (DiNET), Universal Scientific Education and Research Network (USERN), Tehran, Iran
(3)
Department of Immunology, Oslo University Hospital, Oslo, Norway
(4)
K.G. Jebsen Inflammation Research Center, University of Oslo, Oslo, Norway
(5)
Research Laboratory, Nordland Hospital, Bodø, Norway
(6)
Faculty of Health Sciences, K.G. Jebsen TREC, University of Tromsø, Tromsø, Norway
(7)
Centre of Molecular Inflammation Research, Norwegian University of Science and Technology, Trondheim, Norway
(8)
Sanquin Research and Landsteiner Laboratory, Department of Blood Cell Research, Academic Medical Center, University of Amsterdam, Amsterdam, The Netherlands
(9)
Division of Allergy and Immunology, The Children’s Hospital of Philadelphia, University of Pennsylvania Perelman School of Medicine, Philadelphia, PA, USA
Keywords
Complement deficiencyAngioedemaInnate immunityAcquired immunityPrimary8.1 Introduction
The complement system is an essential part of innate immunity, with vital interconnections with the adaptive immune system. Complement was discovered shortly before 1900, when it was recognized as a heat-labile component in serum that “complemented” the bacteriolytic effect of antibodies [81]. Today, around 50 components have been described, comprising molecules present in the fluid phase as well as on the cell surface, involved in complement activation, regulation, and cellular effects [118]. Conventionally, complement is viewed as a first line of defense against microbial particles, essential for the recognition and elimination of foreign structures of pathogenic origin, but also of damaged self-cells or cell debris. Although the innate immune effect is the obvious task of the complement system, additional functions within a broader sense of defense have now also been recognized as complement dependent [85, 118]. The complement system has several interconnections with the adaptive immunity, e.g. via bridging antibody recognition to complement-dependent opsonization and lowering the threshold for antigenic B-cell stimulation [23] and regulating T cells [74, 89]. The complement response has crosstalk connections with the Toll-like receptor-dependent signaling [52, 167] and with the coagulation and kallikrein-kinin systems [43, 62, 94]. Complement activation has also been shown to be involved in embryonic and synaptic development [119, 144] and tissue regeneration [93, 145].
The complement system is activated via the classical, lectin or alternative pathway (Fig. 8.1), which are all initiated by different structures and by different mechanisms [159, 160]. The system is based on the recognition of molecular patterns that lead to the formation of enzyme complexes with proteolytic activity that cleave complement components in a cascade-like manner. The formation of C3- and C5-convertases is central in the cascade. These convertases cleave the components C3 and C5, respectively, leading to the key effects of complement activation. Effector molecules are produced throughout the cascade, leading to opsonization for enhanced phagocytosis (C3b, iC3b and C4b) [35], generation of proinflammatory peptides (C3a and C5a) [78], direct lysis of cells (C5b-9) [51] and co-stimulation of B-cell activation (C3d) [23].

Fig. 8.1
Complement activation pathways
Complement activation is a continuously ongoing process, in which principally all surfaces exposed to complement are prone to trigger activation. These potentially harmful effects are, on healthy host cells, efficiently counteracted by an array of fluid-phase and membrane-bound regulators. Most regulators are focused at the convertase formation and at the degradation of C3b [170].
Complement proteins are primarily produced in the liver by hepatocytes and constitutively secreted in plasma, or induced by inflammatory cytokines during the acute-phase response, but local synthesis of complement proteins by resident or infiltrating cells is pivotal to drive the inflammatory processes. Some proteins, like C1q, C7 and factor D, are mainly produced extra-hepatically, e.g. C1q by macrophages and factor D by adipose and renal cells [100].
Complement deficiencies, acquired or hereditary, have been recognized for almost all of the known components of the complement system (Table 8.1). Acquired deficiency may be caused by infections or immune-complex disorders. Most inherited deficiencies of complement components are expressed in an autosomal recessive pattern. One exception is properdin deficiency, which is inherited in an X-linked manner [47], and another one is C1 inhibitor (C1INH) deficiency, which is a dominant trait [95]. A complement gene defect may give rise to a dysfunctional protein or to a complete absence of the protein. Parents of patients with a complete deficiency of a complement component are usually heterozygotes for the mutation, resulting in approximately half-normal levels of the protein [162]. Half-normal levels of several complement factors, e.g., components of the terminal pathway [164], usually suffice to exhibit normal complement effects, but family studies are needed to identify affected family members [45]. (See Table 1.7 and Fig. 1.14 for updated classification of complement deficiencies).
Table 8.1
Characteristics of primary complement deficiencies
Complement components |
Deficiencya |
Gene |
Inheritance |
Associated features |
---|---|---|---|---|
Classical pathway |
C1q |
C1QABC |
AR |
SLE, Rheumatoid disease, Infections |
C1r |
C1R |
AR |
SLE, Rheumatoid disease, Infections | |
C1s |
C1S |
AR |
SLE, Rheumatoid disease, Infections | |
C4 |
C4A, C4B |
AR |
SLE, Rheumatoid disease, Infections | |
C2 |
C2 |
AR |
SLE, Vasculitis, Polymyositis, Infections | |
Lectin pathway |
MBL |
MBL2 |
AR |
Pyogenic infections |
Ficolin 3 |
FCN3 |
AR |
Recurrent infections | |
Collectin 11 |
COLEC11 |
AR |
3MC syndrome | |
MASP-2 |
MASP2 |
AR |
Pyogenic infections, SLE | |
MASP-3 |
MASP1 |
AR |
3MC syndrome | |
Alternative pathway |
Factor D |
CFD |
AR |
Neisserial infection |
Properdin |
PFC |
XL |
Neisserial infection | |
C3 |
C3 |
C3 |
AR |
Recurrent pyogenic infections, Glomerulonephritis |
Terminal pathway (Membrane attack complex) |
C5 |
C5 |
AR |
Neisserial infections, SLE |
C6 |
C6 |
AR |
Neisserial infections, SLE | |
C7 |
C7 |
AR |
Neisserial infections, SLE, Vasculitis | |
C8a |
C8α |
AR |
Neisserial infections, SLE | |
C8b |
C8β |
AR |
Neisserial infections, SLE | |
C9 |
C9 |
AR |
Neisserial infections, SLE | |
Regulatory proteins |
C1 inhibitor |
C1NH |
AD |
Hereditary angioedema |
Factor I |
CFI |
AR |
Recurrent pyogenic infections | |
Factor H |
CFH |
AR |
Hemolytic-uremic syndrome, Membranoproliferative glomerulonephritisb | |
MCP |
CD46 |
AR |
Hemolytic-uremic syndrome, Glomerulonephritis | |
DAF |
CD55 |
AR |
Paroxysmal nocturnal hemoglobinuria | |
CD59 |
CD59 |
AR |
Hemolysis pyogenic infections | |
PIGA |
PIGA |
XL |
Paroxysmal nocturnal hemoglobinuria | |
CR3 |
ITGB2 |
AR |
Pyogenic infections |
Most of the inherited deficiencies are uncommon, but there are various ethnic and geographical influences on the prevalence of these deficiencies. For example, C9 deficiency is the most common complement deficiency in Japan, where it may occur in up to 0.1 % of the population [36], while it is rare in the Western countries. On the other hand, C2 deficiency has a frequency of up to 0.01 % in the Western countries, but it is extremely rare in Japan [113, 114]. It has been estimated that the prevalence of a hereditary complete complement component deficiency is 0.03 % in the general population, excluding deficiency of mannan-binding lectin (MBL), which is present in homozygous form in as much as 5–25 % of the general population [137, 152], depending on how the deficiency is defined.
Primary complement deficiencies are in particular associated with an increased susceptibility to recurrent and invasive bacterial infections and with autoimmune disorders [9]. Deficiency of C1INH, the main inhibitor of the classical and lectin pathways of complement activation, leads to angioedema. However, this is primarily a result of an increased generation of bradykinin caused by decreased inhibition of the kallikrein-kinin system [18], and therefore is not to be regarded as a real complement deficiency disease.
Patients with a deficiency of an early complement component in any of the activation pathways, which leads to decreased activation of C3, often manifest with recurrent pyogenic infections, principally with encapsulated bacteria such as Streptococcus pneumoniae and Haemophilus influenza type-b. This is primarily because opsonization of micro-organisms followed by phagocytosis is a main host defense against these organisms [114]. For deficiencies of the terminal complement components (C5-9), recurrent systemic neisserial infections are the dominant manifestations, because the clearance of these bacteria is highly dependent on C5b-9-mediated lysis [28]. However, the severity of the neisserial infections in these patients is usually mild. Properdin deficiency usually leads to severe neisserial infections.
Autoimmune systemic lupus erythematosus (SLE)-like diseases are typically seen with classical pathway component deficiencies, in particular with C1q deficiency [156]. Nine out of ten C1q-deficient patients suffer from SLE or SLE-like disease [126]. The frequency is especially high also in C1r/s- and C4-deficient patients. This highlights the importance of the classical pathway in the clearance of apoptotic cells, tolerance, and processing immune complexes.
Newly discovered mutations within two genes of the lectin pathway were recently connected to a developmental disorder connected to the Malpuech, Michels and Mingarelli-Carnevale (3MC) syndrome [22, 119, 134]. This is the first evidence that a human deficiency of a complement component can lead to a developmental disorder.
Although complement deficiencies are uncommon in the general population, individuals with such deficiencies frequently suffer from serious and often life-threatening diseases, which need specific therapy, including vaccines and easy access to antibiotics. Therefore, patients with recurrent or invasive bacterial infections, certain kidney diseases, familial autoimmune disease or angioedema, should always be tested for complement deficiencies [162].
Screening tests for complement component deficiency have traditionally included functional hemolytic tests for the classical (CH50 test) and alternative pathways (AH50 test, also called AP50 test). These assays utilize sensitized sheep erythrocytes and rabbit erythrocytes, respectively, for complement activation. The amount of serum necessary for 50 % erythrocyte lysis is determined. Low levels of CH50 or AH50 necessitate additional evaluation. If both CH50 and AH50 are low or absent, one or more of the common and terminal components (C3, C5, C6, C7, C8, and C9) may be missing. If the CH50 is low or absent but the AH50 is normal, a classical/lectin pathway component may be missing, whereas if the AH50 is low or absent but the CH50 is normal, an alternative pathway component may be missing [162].
Recently, a novel enzyme-linked immunoassay (ELISA) has been developed for separately revealing deficiencies of classical, lectin or alternative pathway components [129]. This is an enzyme-linked immunoassay, measuring the function of complement based on selective binding of classical pathway components to surface-bound IgM, lectin pathway components to mannan and alternative pathway components to lipopolysaccharide (LPS). The read-out is common for the three pathways, namely detection of polymerized C9. From this functional complement screening test it can be deduced which components might be deficient; e.g. an MBL defect will be revealed by a low lectin pathway activity, a C2 defect will show low activity in both the classical and the lectin pathway, C3 or C5-C9 deficiencies will result in low activity in all three pathways. An advantage with this screening system is that properdin deficiency consistently shows low alternative pathway activity, which is not always the case with the hemolytic AH50 assay.
Measurement of the fragments formed during the enzymatic reaction cascade is another useful technique for evaluating the complement system activity [104]. For instance, C4a, C4d and C4bc are used for the determination of classical or lectin pathway activation, Ba, Bb and the convertase C3bBbP are measured for evaluating alternative pathway activation, C3a, iC3b, C3bc and C3dg to detect C3 activation, and finally C5a and soluble C5b-9 to determine terminal pathway activation [162]. Importantly, activation fragments must be specifically detected in the presence of the corresponding native proteins. This is usually accomplished by using monoclonal antibodies binding to neoepitopes exclusively exposed in cleaved fragments or complexes formed subsequent to complement activation [104].
Individual components of the complement system can be measured by immunochemical methods, including immunoprecipitation assays such as nephelometry, turbidimetry, radial immunodiffusion and radio- and enzyme-immunoassays [162]. In certain cases, functional assays are required for further evaluation, despite the presence of normal amounts of component protein detected by immunochemical assays [112].
Although effective management for complement deficiencies is restricted, most complement-deficient patients will undoubtedly benefit from a correct diagnosis [136]. In case of some complement inhibitor deficiencies, like C1INH and factor H, it is crucially important to make the diagnosis, since these are potentially life-threatening diseases that can be effectively treated.
If a complement deficiency is identified, management focuses on the associated disease, such as infection or autoimmunity. Prevention of infections by vaccination and immediate treatment with appropriate antibiotics are crucial. In some of these patients, antibiotic prophylaxis may be considered, and special attention should be given to vaccination against encapsulated organisms such as Streptococcus pneumoniae, Haemophilus influenzae and Neisseria species. Also early recognition and management of autoimmune diseases are necessary [162]. In case of C1INH deficiency, prophylactic treatment can be required, as well as acute treatment with C1INH concentrate.
In 2007, the first complement inhibitor, eculizumab (Soliris®), was approved for the treatment of paroxysmal nocturnal hemoglobinuria (PNH) [60]. The inhibitor is a humanized monoclonal antibody prepared on the IgG2/4 chimeric backbone, targeting C5 and preventing its cleavage; i.e. C5a is not released and the lytic C5b-9 complex is not formed. Eculizumab is now also approved for the treatment of atypical hemolytic uremic syndrome (aHUS) [50]. In aHUS, kidney transplantation may also be considered along with the symptomatic treatment, primarily for those with mutations in the membrane cofactor protein (MCP).
8.2 Deficiencies of Classical Pathway Components (C1q/C1r/C1s Deficiencies, C4 Deficiency, C2 Deficiency)
8.2.1 Definition
The classical pathway is, in general, activated by clusters of IgG or IgM, whose Fc regions are bound by the recognition molecule C1q. C1q has a hexameric structure, which exhibits low affinity for monomeric IgG but binds much stronger to aggregated IgG [77]. There is an IgG subclass difference in activation, i.e. IgG3 is the most reactive, followed by IgG1 and IgG2. C1q does not bind to IgG4 [11]. C1q also does not bind to free IgM, but antigen-bound IgM exposes new sites in the CH3 domain for C1q recognition and complement activation [111]. Although the antigen-bound IgG/IgM is viewed as the traditional target for C1q binding and classical pathway activation, C1q is a pattern recognition molecule, and a growing number of structures are now acknowledged as C1q ligands. These ligands include pentraxins (C-reactive protein) [2], abnormal endogenous proteins (prions, β-amyloloid fibers) [131], structures associated with cell death (phosphatidylserine, DNA) [108, 109] and glycosaminoglycans/proteoglycans [38, 53].
While not every ligand will activate complement, C1q binding may induce clearance of particles via direct opsonization, implicated especially in the removal of apoptotic cells [103, 155]. The majority of C1q forms a calcium-dependent C1 complex with C1r2 and C1s2 [169]. Upon activation, a conformational change within C1q occurs, which leads to enzymatic cleavage and thereby activation of the serine protease C1r, and this in turn cleaves and activates the serine protease C1s. C1s cleaves C4 into C4a and C4b, with C4b covalently attaching to the adjacent activation site (e.g. a cell coated with antibodies) and the small C4a fragment diffusing into the fluid phase. C2 associates with C4b and is cleaved by C1s into C2a and C2b. The C2a and C4b form on the target cell surface the classical pathway C3 convertase (C4bC2a), which cleaves C3 into C3a and C3b. C3a is released into the fluid phase as an anaphylatoxin and C3b is deposited on the target cell where it serves as an opsonin [capable of binding to the complement receptor (CR)1 on phagocytes and erythrocytes] or is further cleaved to iC3b as another opsonin [capable of binding to the CR3 receptor (CD11b/CD18) on phagocytes]. Moreover, C3b interacts with C4bC2a to form the C5 convertase of the classical pathway (C3bC4bC2a).
The surface-bound C3b is also a trigger for direct alternative pathway activation, which underlines the importance of the classical pathway for initiating an activation that subsequently is amplified within the alternative pathway. Even when the trigger of complement activation is solely dependent on the classical or lectin pathway, a large fraction of C3 cleavage can actually arise from an amplification loop within the alternative pathway. This pathway is initiated spontaneously by a certain amount of constant hydrolysis of the C3 thiol-ester bond, thus rendering C3(H2O), which is “C3b-like” and binds factor B [55]. All three complement activation pathways (classical, lectin and alternative pathway) share the same terminal C5-C9 activation sequence (Fig. 8.1).
Complement deficiencies within the classical pathway have highlighted the importance of this pathway in the elimination of pyogenic encapsulated bacteria, especially Streptococcus pneumoniae and Haemophilus influenzae strains. Classical pathway activation effectively opsonizes these bacteria for phagocytosis by myeloid cells. Furthermore, classical pathway deficiencies increase the susceptibility for autoimmune disorders, especially for SLE or an SLE-like disease; this is particularly evident for C1q deficiency [141]. The mechanism for this increased susceptibility is not yet fully understood, but lack of C1q leads to impaired clearance of immune complexes and apoptotic cells, thereby increasing the exposure of autoantigens (the waste-disposal hypothesis) to the immune system [92]. The importance of C1q for the maintenance of B-cell tolerance against self-antigens and C1q-dependent cytokine response may also play a role in the pathogenesis of SLE upon C1q deficiency [156, 165].
8.2.2 Etiology
Classical pathway deficiencies (C1, C4, and C2) are inherited in an autosomal recessive pattern. Deficiencies of C1 and C4 are rare, and the genetics are complex, involving multiple genes for each component. C1q (encoded by C1QA, OMIM*120550, C1QB, OMIM*120570 and C1QC OMIM*120575), C1r (C1R, OMIM*613785), and C1s (C1S, OMIM*120580) are required for C1 function. Thus, if any one of these subunits is missing, the functional complex cannot form. Deficiency of C1q is either due to a failure in synthesis or to the synthesis of a non-functional low-molecular weight C1q [88]. Twelve causative mutations within C1QABC have been described, in 64 confirmed cases from 38 families (2011) [126]. Fourteen cases of C1r and C1s deficiency have been reported (2010) [88]. The C1r and C1s genes are closely linked, and patients deficient in C1r are usually low in C1s as well [88]. C4 exists in humans in two forms, C4A (acidic) and C4B (basic), encoded by two adjacent coexisting genes (C4A, OMIM*120810 and C4B, OMIM*120820). All four alleles must be deleted or defective to cause total C4 deficiency [125]. Moreover, both genes may be present in up to seven copies and contain numerous polymorphisms. Complete C4 deficiency is extremely rare and has only been described in 28 cases (2010) [88].
C2 deficiency (C2, OMIM*613927) is the most common classical pathway component deficiency, with an incidence of 1 homozygous case per 20,000 in Western white populations [114]. C2 deficiencies are classified into two types: type I representing absence of C2 synthesis and type II representing failure in protein secretion [67]. Nine out of ten cases are of type I, with a majority caused by a 28-bp deletion in exon 6 [146].
8.2.3 Clinical Manifestations
Patients with a deficiency of an early classical pathway component (C1, C4, or C2) are predisposed to infections with pyogenic encapsulated bacteria, but the infections are usually milder than those observed in patients with a deficiency of properdin, C3, or a terminal component. A study of 40 C2-deficient patients in Sweden reported 57 % of them to have invasive infections, of which 30 % had repeated infections [68]. Most significant, deficiencies of classical pathway components are frequently associated with the development of autoimmune syndromes, particularly SLE. Individuals with total deficiency of any component of the C1 complex or C4 display a higher occurrence of SLE and usually more severe manifestations of the disease compared to a deficiency of C2 [88]. The onset is in general early, and the female to male ratio in C1/C4 deficiency is 1:1, in contrast to deficiency of C2, in which the epidemiology of SLE has a later onset and with a female predominance. Of the 64 described cases of C1q deficiency, 88 % exhibited SLE or SLE-like disease [126].
The corresponding fractions of patients with SLE in C1s/r and C4 deficiency are 57 % and 75 %, respectively [114]. Also partial C4 deficiency is associated with the disease, primarily regarding C4A. Homozygous C4A deficiency is up to 5 times more prevalent in the SLE patient cohort compared to the healthy population [88]. Approximately 10 % of the C2-deficient subjects develop SLE [114]. The highly significant relation between classical pathway deficiencies and the development of an SLE-like disease underline the critical function of the classical pathway, primarily connected with the clearance of immune complexes and apoptotic cells [9]. C2 deficiency is also associated with an increased prevalence of atherosclerosis and cardiac disease [68].
8.2.4 Diagnosis
Classical pathway deficiency can be detected by the traditional CH50 assay, based on hemolysis of sensitized sheep erythrocytes, or by the novel ELISA screening assay for all pathways [129]. An absence or a decrease of CH50 in the presence of normal AH50 indicates that at least one of the early components of the classical pathway is missing or low [115]. Complete deficiencies of C1q, C1r or C1s will induce a low classical pathway activity, but a normal lectin and alternative pathway activity in the ELISA screening test. A complete deficiency of C4 or C2 will lead to a low classical and lectin pathway activity, whereas the alternative pathway activity will be normal.
The identification of the missing component follows from the recovery of complement activity in either of these tests by the addition of a purified component, by immunochemical tests, or by gene sequencing of the component in question.
8.2.5 Management
Treatment of patients with complement deficiencies is based on treatment of the clinical manifestations. Identification of autoimmune diseases in patients with deficiencies of early components of the classical pathway is necessary, anti-inflammatory therapies should be used for management of the autoimmune disease. Recurrent infections in these patients should be managed with appropriate antibiotic therapy and vaccination [162]. Allogenic Hematopoietic stem cell transplantation (HSCT) has recently been applied for a few number of patients with C1q deficiency that were resistant to medical therapy to cure SLE [107]. While autoimmune disease is often the dominant clinical picture, death is most often due to infection. Therefore, education and strategies to ensure ready access to medical care and antibiotics is critical. Furthermore, studies suggest an important role for complement in atherosclerosis, and aggressive management of cardiac risks is important.
8.3 Deficiencies of Lectin Pathway Components (MBL Deficiency, MASP–2 Deficiency, MASP–3 Deficiency, Ficolin 3 Deficiency, Collectin 11 Deficiency)
8.3.1 Definition
The overall structure of the lectin pathway (Fig. 8.1) is similar to that of the classical pathway, and both pathways share C4 and C2 during downstream activation. The pattern recognition molecules of the lectin pathway comprise five lectins capable of initiating activation: mannan-binding lectin (MBL), ficolin 1, 2 and 3 (also termed M-, L-, H-ficolin) and collectin 11 (also termed collectin kidney 1) [24, 25, 54, 58]. Collectin 11 is the most recently characterized member of the complement-activating lectins, but has until now only been shown to activate complement in vitro [58, 90]. Currently, the data describing in which context and to what extent collectin 11-dependent opsonization and activation is significant, are limited.
Activation of the lectin pathway is initiated by any of these lectins binding specific carbohydrate structures (MBL) or patterns of acetyl groups (ficolins). Analogously to C1s/C1r in the classical pathway, serine proteases are associated to the recognition molecules of the lectin pathway. These proteases are termed MASP-1, MASP-2 and MASP-3. MASP-2 is ubiquitous for any lectin pathway activation; it becomes activated after lectin binding and cleaves both C4 and C2 [3, 56]. Recent data suggest MASP-1 to be equally important for lectin pathway activation, acting as a crucial activator of MASP-2 [56]. MASP-1 is not able to cleave C4 but has been indicated to substantially contribute to C2 cleavage [56]. Currently, the relevance of MASP-3 in complement activation is not clear [22]. The cleavage of C2 and C4 results in the formation of a C3 convertase (C4bC2a), which is identical to that of the classical pathway. The same is true for the subsequent downstream events.
8.3.2 Etiology
Deficiency of MBL (MBL2, OMIM*154545) is predominantly caused by one of three SNPs in exon 1 of the MBL2 gene, coding for the allelic variants referred to as B, C and D, with A being wild-type MBL [40, 87, 91, 147]. Any of these mutations affect the essential assembly of a functional MBL oligomer from MBL monomers [39, 40]. There are also additional SNPs in the promoter region of MBL2 that affect the serum MBL level [39, 40]. These variants of MBL2 are quite common, and thus, MBL deficiency is seen in many populations. MBL deficiency is not a complete deficiency but is related to a serum “cut-off” value, often <100 ng/mL (normal levels range from 0.5 to 5 μg/mL). Referring to this value, approximately 10 % of Caucasians are MBL deficient, and up to 30 % exhibit levels below the normal range [124, 151]. Thus, MBL deficiency is one of the most common protein deficiencies in humans. It has been claimed that the genetic evolution of MBL2 represents heterosis, implying that there is an advantage of being heterozygous [57]. According to this concept, a complete deficiency of MBL might increase infection susceptibility, whereas high levels of the protein may lead to host tissue damage caused by excessive complement activation (“the double-edged sword”) [99].
In contrast to MBL, deficiencies in the ficolins and in collectin 11 are rare. The ficolin genes are polymorphic, since both ficolin 1 (FCN1, OMIM*601252) and ficolin 2 (FCN2, OMIM*601624) present polymorphisms in the promoter and structural parts that affect the serum levels, but no cases of complete ficolin 1 or 2 deficiency have been described to date. In 2009, a complete ficolin 3 (FCN3, OMIM*604973) deficiency was described in a patient suffering from recurrent severe pulmonary infections [101]. A frameshift mutation (FCN3 + 1637delC) in exon 5 of FCN3 caused a complete ficolin 3 deficiency in the homozygous patient. The allele frequency of this mutation was reported to be about 0.01 [101, 102]. Collectin 11 (COLEC11, OMIM*612502) is highly conserved, and the function within the complement system is to date largely unknown. Recently, two reports by Sirmaci et al. and Rooryck et al. linked mutations within the MASP1 (OMIM*600521) and COLEC11 genes to developmental disorders classed in the 3MC syndrome [119, 134]. Mutations within the well-conserved carbohydrate recognition domain of COLEC11, with premature termination or deletion of exon 1–3, were found in seven families. Collectin 11 was further linked to embryonic development in zebrafish, in which a functional loss of collectin 11 leads to craniofacial abnormalities that could be rescued by injection of human collectin 11 mRNA [119].
MASP1 codes for the three splice variants MASP-1, MASP-3 and MAP44, the latter lacking the serine protease domain. Mutations within the exon specific for MASP-3 have been suggested to be causative for the 3MC syndrome, found in six families. MASP1 was also linked to the embryonic development in the zebrafish [119]. MASP-2 (MASP2, OMIM*605102) deficiency was first described in 2003 by Stengaard-Pedersen et al. in a patient with non-functional lectin pathway as a result of a homozygous missense mutation (p.D120G) in exon 3 of MASP2 [142]. Later studies have shown MASP-2 deficiency to be fairly common, caused by several different polymorphisms, of which the frequent p.D120G has an allelic frequency of 0.01–0.04 in Caucasians [13, 153].
8.3.3 Clinical Manifestations
Deficiency of MBL generally increases the risk of any type of infection but is mostly related to an increased frequency of pyogenic infections, including pneumococcal infection and sepsis, particularly in neonates/infants or in patients undergoing immunosuppressive treatment. The increased risk in infants can be explained by the “window” period from 6 to 18 months of life, in which the mother’s antibodies have disappeared and the levels of those of the child are still insufficient, resulting in reduced immune defense. Normally, MBL deficiency is not associated with an increased incidence of infections, but MBL may be of importance as a redundancy protein in immunosuppressed patients, e.g. those treated with cytostatics, irradiation for malignancy or after HIV infection. MASP-2 deficiency is associated with an increased susceptibility to infection and autoimmunity disease [142]. Lung infections and cystic fibrosis have been reported in patients with MASP-2 deficiency, but homozygous mutations are also found in the healthy population [37, 106, 153]. Ficolin 3 deficiency has been described in one patient suffering from recurrent respiratory disease and in two premature infants with severe necrotizing enterocolitis [101, 127]. A deficiency in the lectin pathway may lead to autoimmune diseases [114], but may also modify the progress of a disease to be more benign, as seen in rheumatoid arthritis patients [41], or to be more serious, as seen in cystic fibrosis [42]. A 2- to 3-fold increased incidence in MBL deficiency has been reported in patients with lupus erythematosus [75]. Some studies have shown that MBL deficiency is related to cardiovascular disease, in which a deficiency might be either beneficial or detrimental [105, 121]. Thus, at present, any conclusion with respect to the clinical consequences of MBL deficiency can hardly be drawn.
Whole exome sequencing has revealed a link between mutations within the MASP1 gene and the cause of the 3MC syndrome [134]; this was later extended also to mutations within the COLEC11 gene. Patients with the 3MC syndrome experience a spectrum of developmental disorders, of which the most common are growth and mental retardation, facial dysmorphism (e.g. hypertelomerism, cleft lip and palate, high arched eyebrows) and hearing loss [154]. Complement has, in experimental animal models, been implicated in embryonic development [4, 143, 157], but this is the first link in humans in which mutations within complement genes are related to the pathogenesis of a developmental disorder.
8.3.4 Diagnosis
MBL-dependent activation of the lectin pathway can be assessed by a screening ELISA [129]. MBL deficiency and MASP-2 deficiency will cause low lectin pathway activity, whereas classical and alternative pathway activities will be normal. Moreover, a functional assay of MBL binding and capacity to activate C4 has also been described, in which the amount and function of later complement components are not of importance [112]. Further analysis includes immunochemical quantitation of MBL and genotyping of MBL and MASP-2.
8.3.5 Management
8.4 Deficiencies of Alternative Pathway Components (Factor D Deficiency, Properdin Deficiency)
8.4.1 Definition
In contrast to the classical and lectin pathways, the alternative pathway is triggered primarily not by the exposure of molecular patterns of pathogenic or damaged cells, but instead by any surface that lacks sufficient complement activation regulation. The trigger of the alternative pathway is C3b and C3(H2O), the latter being a C3b-like molecule that at a slow rate is produced in plasma by spontaneous hydrolysis of native C3 (a process termed “C3 tick-over”) (Fig. 8.1) [110]. Once C3b/C3(H2O) is exposed on surfaces or in the fluid phase, respectively, it associates with factor B, which subsequently is cleaved by factor D into Ba and Bb. Ba is released into the fluid phase, and Bb forms, together with C3b/C3(H2O), the C3 convertase of the alternative pathway C3bBb [27]. Analogous to the C4b2a, C3bBb cleaves C3 into fluid phase-released C3a and surface-deposited C3b. C3b of any origin (classical, lectin or alternative pathway) triggers further alternative pathway activation. In this way, the alternative pathway is responsible for a potent amplification of complement activation, referred to as the “amplification loop” [55, 82]. Alternative pathway activation/amplification is efficiently counteracted by membrane-bound and fluid-phase regulators present at or in close proximity to host cells, of which a majority is focused at the level of convertase formation and C3b degradation. Properdin is a positive regulator of the alternative pathway, as it acts by stabilizing the C3bBb complex. The properdin-stabilized C3bBbP has a 10-fold higher stability than C3bBb [26]. Properdin has been proposed to be a pattern recognition molecule of the alternative pathway, suggested to bind directly to surfaces (e.g. to LPS) and attract C3b, thereby triggering alternative pathway activation [76, 139]. This may explain the increased susceptibility to neisserial infection in cases of properdin deficiency. An alternative pathway C5 convertase is formed when C3b associates to C3bBb, thus activating the terminal pathway as in the classical and lectin pathways.
8.4.2 Etiology
Early alternative pathway deficiencies of factor D (CFD, OMIM*134350) and properdin (CFP, OMIM*300383) are rare. No factor B (CFB, OMIM + 138470) deficient individuals have been found to date. Total homozygous deficiency of factor D has been described in three families [5, 59, 140], of which two were defined at the molecular level [5, 137]. Properdin deficiency is found more frequently than factor D deficiency. The properdin gene is located on the X-chromosome, therefore the properdin deficiency is generally present only in males, whereas female carriers typically have half of the normal plasma level [32]. Properdin deficiency is classified into three types [135]; type I representing complete absence in protein, type II with diminished protein levels (typically 1–10 % of normal levels) but still functionally active and type III presenting with properdin in normal levels but not functional. Type I is the most frequent phenotype of properdin deficiency, predominantly caused by frameshift mutations and premature stop codons, leading to transcription of a truncated protein, which is rapidly degraded [32].
8.4.3 Clinical Manifestations
Alternative pathway component (properdin, factor D) deficiency is associated with severe, fulminant infections by Neisseria gonorrhoeae or N. meningitidis, with a high mortality rate [32]. All index patients in factor D-deficient families presented with Neisseria meningitides infection [140]. Properdin deficiency is significantly linked to meningococcal disease, the risk of fulminant and fatal meningococcal infection was estimated to be 250 times higher in the type I-deficient patients compared to the general population [31]. The disease is often seen with sepsis, and the lethality is higher in deficient patients compared to patients with normal properdin levels [31]. The deficiency of alternative pathway components is not associated with autoimmune diseases [9].
8.4.4 Diagnosis
The traditional AH50, based on the lysis of rabbit or guinea pig erythrocytes, has been used for screening of alternative pathway-component deficiencies but is hampered by not always detecting properdin deficiency. This problem does not occur with the ELISA screening assay [129]. A defect in alternative pathway components (factor B, factor D or properdin) will yield low alternative pathway activity with normal classical and lectin pathway activity. Alternative pathway components can be further evaluated by functional and immunochemical tests, and by gene sequencing.
8.4.5 Management
Patients with alternative pathway-component deficiencies should be vaccinated with the tetravalent meningococcal vaccine, particularly important in properdin-deficient persons [136]. Early antibiotic treatment is mandatory.
8.5 Deficiency of Complement Component C3
8.5.1 Definition
Present at approximately 1.3 mg/mL in blood, complement component 3 (C3) is the most abundant complement protein in plasma. C3, together with factor B and MASP, is thought to be among the most ancient complement proteins [166]. By displaying a variety of conformations and fragments, it exerts multiple functions in the cascade, including alternative pathway activation, convertase formation, opsonization (C3b/iC3b), stimulation of inflammatory cells (C3a), and B-cell stimulation (C3d).
Recent crystal structure determination of native C3, C3c [66] and C3b in complex with factor B and D [33] has led to new insights into this complex component. Native C3 is a rather inert molecule, consisting of an alpha-chain and a beta-chain held together with a disulfide bond [122]. Upon proteolytic cleavage by a C3 convertase, C3a is split from the alpha chain. The remaining C3b undergoes a major conformational change that leads to the exposure of a previously hidden reactive thioester. In the vicinity of a surface, this thioester mediates the covalent attachment of C3 to hydroxyl groups or amines, whereas in the absence of an accepting surface, it hydrolyzes within fractions of a second [49]. Surface-bound C3b is able to interact with factor B in an alternative pathway C3 convertase as well as with complement receptors (CR) 1, 3, and 4. Following surface attachment, C3b is prone to inactivation by factor I, in which case the alpha chain is cleaved into iC3b, which has even higher affinity for the important CR3 phagocytic receptor. iC3b can then be further degraded to C3c and C3dg [19]. Apart from being proteolytically activated, C3 can adopt a C3b-like conformation through the spontaneous hydrolysis of the thioester of fluid phase C3. C3(H2O) still retains C3a in the alpha chain but has C3b-like functions, including alternative pathway C3 convertase formation and receptor interactions [49].
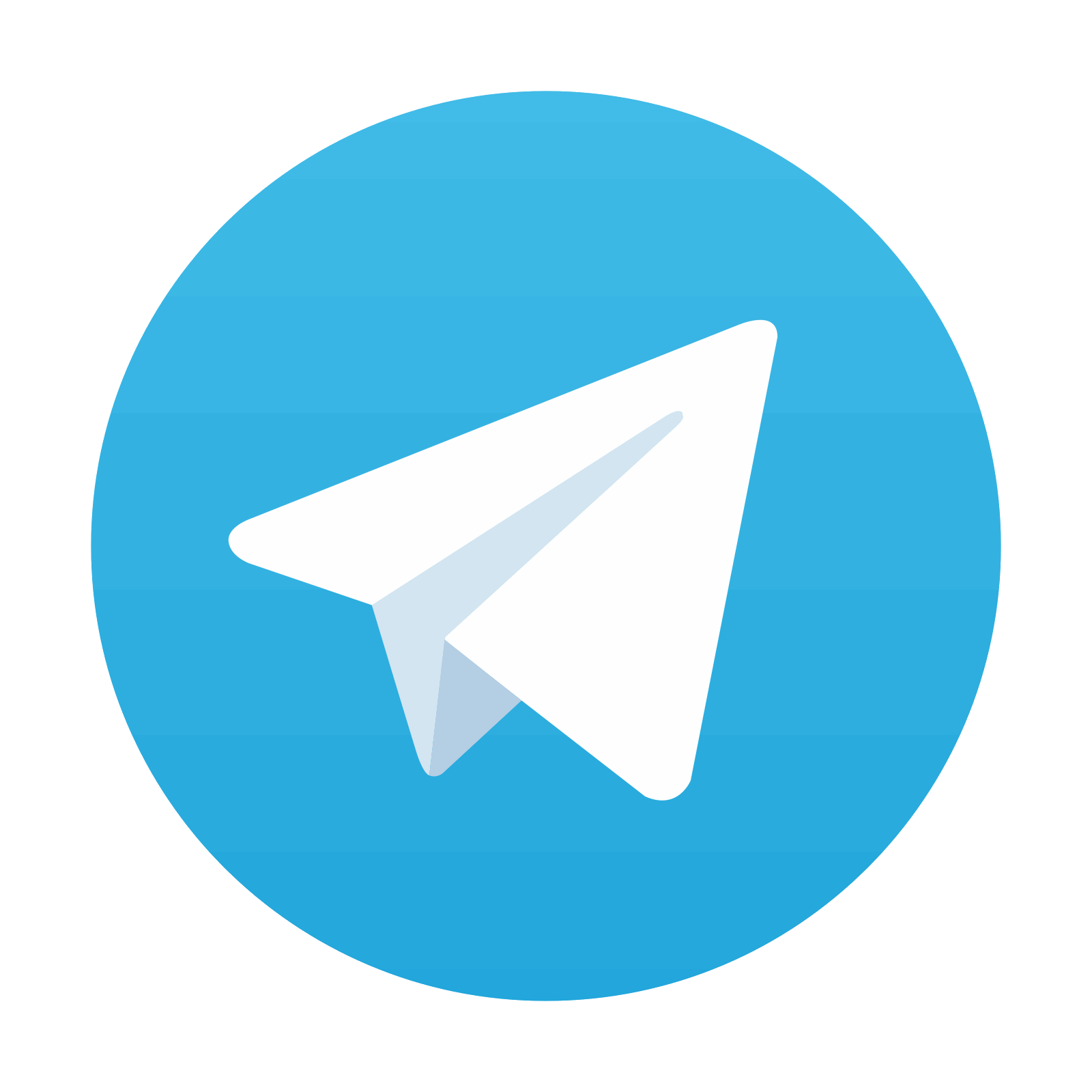
Stay updated, free articles. Join our Telegram channel

Full access? Get Clinical Tree
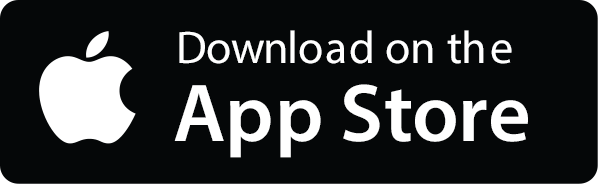
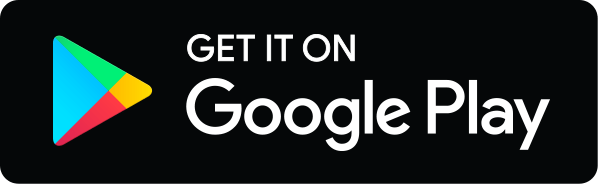