Drug
Potency
(relative to cortisol)
Salt-retaining properties
Half-life
Biologic half-life
Oral bioavailability (%)
Cortisone acetatea
0.8
Present
1.5
8–12
90
Prednisolone
5
Moderate
2
12–36
100
Methylprednisolone
4
Low
2.3
12–36
80–100
Triamcinolone
4
Virtually absent
1.5
12–36
100
Betamethasone
30
Virtually absent
5
36–54
90
Dexamethasone
30
Virtually absent
3.5
36–54
80–90
In plasma, GC binds two proteins: transcortin (also called CBG) and albumin. GCs are extensively metabolized and excreted as metabolites, some of which have weak androgenic activity. Among the old-generation GCs, the half-life tends to be longer in the newer compounds (e.g., betamethasone and dexamethasone) than in the older ones (e.g., prednisolone and triamcinolone). However, the GC half-life never exceeds 5–6 h. The duration of GC effects in target tissues (referred to as the biological half-life) is far superior to the plasma half-life owing to the genomic properties of GCs that change cell function over time. Newer GCs of the old generation are also more potent and thus can be used at lower doses. They also have much lower sodium-retention activity.
New Generation of Topical GCs
New-generation GCs (Table 2) are used to treat diseases that require long-term treatment, and can also benefit local treatments. They are effective and have a decreased frequency of adverse systemic effects; therefore, new-generation GCs are the first choice for the topical treatment of asthma, allergic rhinitis, rhinosinusitis, nasal polyposis, and inflammatory bowel diseases. By contrast, old-generation GCs should not be used to treat these diseases unless systemic treatment is required owing to a lack of response to the topical treatment.
Table 2
Properties of new-generation GCs
Drug name | Pro-drug | Relative potencya | Bioavailability (%) | Biochemical properties [41] | Treatment dose | ||||
---|---|---|---|---|---|---|---|---|---|
Intranasal delivery [20] | Inhalation [15] | Lung deposition (pmol/g)c | Normalized lipophilicitye | Tendency to form lipid conjugatesg | Allergic rhinitis (mg/day (pedex))h | Asthma (mg/day) | |||
Beclomethasone dipropionate | Yes | 13b | 42 | 2 (62b) | –d | –d | –d | 0.08 | 0.3 |
Budesonide | No | 9 | 33 | 6–13 | 250 | 1 (2,500f) | ≈100 % | 0.1 | 0.2 |
Fluticasone propionate | No | 8–19 | 2 | 12–17 | 500 | 3.2 | 0 % | 0.1 | 0.2 |
Fluticasone furoate | No | 30 | <1 | 15 | –d | –d | –d | 0.05 | 0.2 |
Mometasone furoate | No | 12–22 | <0.1 | 11 | 150 | 2 | 0 % | 0.05 | 0.2 |
Ciclesonide | Yes | 12b | <0.1 | 22 | 180 (180b) | 4 (2.5b) | ≈50 % (≈70 %b) | 0.04 | 0.1 |
New-generation GCs can also be used in other topical treatments (e.g., eye or skin) but there is no reason to prefer them. In fact, for these treatments, the systemic side effects are infrequent and similar to those of old-generation GCs because of the low doses and the relatively low adsorption rate.
The key feature shared by all new-generation GCs is that they are much more active locally compared with the whole organism, due to pharmacokinetics properties summarized in Table 2 [15, 20, 41]. For example, studies have examined hypothalamic–pituitary–adrenal (HPA) axis suppression with intranasal GCs and have found they have minimal effects on the HPA axis [11]. The local effectiveness and systemic toxicity of inhaled GCs can be influenced by a number of drug-related systemic factors including oral bioavailability, plasma protein binding, and systemic clearance, as well as by local factors such as oral and pulmonary deposition, lipophilicity, and lipid conjugation. Local safety is influenced by the oropharyngeal deposition and activity (pro-drug vs. active drug) of the GC.
In patients with asthma, delivery devices and pharmaceutical formulations that determine particle size are crucial for increasing the percentage of GCs in the target tissue (bronchioles) and reducing the doses [47]. Inhaled GCs are generally delivered either via hydrofluoroalkane metered-dose inhalers (HFA-MDI) or dry-powder inhalers (DPI). HFA-MDI can be formulated as solutions or suspensions, and it is thought that solutions are slightly better than suspensions and DPI at delivering a greater fraction of small particles that reach the target. However, these effects depend on the drug used. Extra-fine particle formulation of HFA-beclomethasone dipropionate showed improved total and small-airway deposition as well as greater effects on lung function compared with the older large-particle chlorofluorocarbon formulation [4]. Ciclesonide has been formulated as a solution HFA-MDI with the majority of particles within the 1.1–2.1-μm size range, resulting in a very high pulmonary deposition (52 %) [19]. In order to decrease local adverse effects (dysphonia, stomatitis, and dry mouth), it is recommended to administer medication with a spacer, gargling, rinsing the mouth, and washing the face after inhalation (if nebulizers are used), as well as washing the spacer [25]. However, no scientific evidence for such procedures is available with the exception of gargling, which was shown to be protective for female patients using DPIs [35]. In children aged 2–5 years, synchronization of breathing with drug delivery is not sufficient to allow proper administration. In these patients, spacer devices with valves are indicated and are more useful than nebulizers for GC delivery, considering the importance of particle size.
Most new-generation GCs are characterized by low bioavailability of the active drug, which is predominantly determined by four factors: significant first-pass effect, lipophilicity, strong tendency of some molecules to form lipid conjugates, and local activation (pro-drugs).
To understand the importance of the first-pass effect, it must be noted that even in the presence of an accurate delivery, the relevant percentage of an inhaled drug for asthma treatment is deposited in the mouth and first respiratory routes, and owing to mucus clearance is swallowed. A similar phenomenon is observed with GCs prescribed for allergic rhinitis treatment. Therefore, a significant first-pass effect greatly reduces the plasma concentration of the drug.
All new-generation GCs are not soluble in water with the exception of budesonide showing low water solubility. All have fairly good lipophilicity (Table 2). Moreover, budesonide and ciclesonide form esters with fatty acids (Table 2) and conjugates are pharmacologically inactive. The lipophilicity of new-generation GCs and their tendency to form lipid conjugates determine the high concentration of drug in the first cells encountered at feeding time and the slow release of active drug in the following hours. This mode of “deposit” is used to reduce the frequency and dosage of the drug, and slows drug redistribution. Moreover, beclomethasone and ciclesonide are pro-drugs that are activated more efficiently in the lung than in the oropharynx. In particular, ciclesonide is converted to the active metabolite desisobutyryl-ciclesonide (des-CIC) by esterases. Des-CIC has a much higher affinity for GR than ciclesonide has (~100 times higher) [48]. In the lung, Des-CIC forms reversible conjugates with fatty acids, such as Des-CIC oleate and Des-CIC palmitate.
New-generation GCs appear to have similar efficacies as older-generation GCs and a better safety profile. Although some pharmacological properties might suggest that mometasone and ciclesonide have a better toxicological profile compared with the other new-generation GCs, definitive data will be provided by clinical studies, particularly data assessing long-term adverse effects in children. In this context, the most interesting parameter is growth inhibition of GC-treated children with asthma. These studies are required to be performed over long periods and should provide a direct comparison between new and old GCs and how they affect growth. Moreover, growth inhibition does not necessarily become pronounced over time, but this requires even longer studies [38].
Regarding local effects, a recent study demonstrated that ciclesonide, a pro-drug, has local adverse effects similar to mometasone, which is not a pro-drug [7], suggesting that clinical translation of preclinical data is not necessarily linear. In conclusion, it is difficult at present to establish which new-generation GC should be the first choice.
Selective GR Agonists
As summarized in the preceding sections, there are several mechanisms by which GR modulates gene transcription. However, GRE-dependent transactivation and transcription factor sequestration are the most common mechanisms for promoting gene upregulation and downregulation, respectively. Because most metabolic effects of GCs depend on gene upregulation and most anti-inflammatory effects depend on decreased production of pro-inflammatory cytokines, some researchers have hypothesized that GRE-dependent transactivation is the dark side of GCs, whereas transcription factor heterodimerization and sequestration forms the basis of their anti-inflammatory activity [17, 65]. Thus, chemists have synthesized so-called selective GR agonists (SEGRAs) that favor GR heterodimerization rather than homodimerization. Such compounds, which are cholesterol-derived or nonsteroidal GCs, remain in clinical development [17, 65].
One example, 21OH-6,19OP, is a synthetic, conformationally rigid, highly bent pregnane steroid. It can bind the thymic GR but not the kidney mineral corticoid receptor or uterus progesterone receptor. It acts as a dissociated GC because it can inhibit RelA and AP-1-induced gene activation while simultaneously failing to increase liver glycogen [75]. In addition, it lacks GC-associated chemoresistance in a mouse mammary model [51].
Some dissociated GR ligands are nonsteroidal molecules, such as Abbott ligand 438 (AL-438), which possesses anti-inflammatory activity but not bone reduction in vitro, or Compound A, a plant-derived GR modulator that ameliorates clinical signs of disease in inflammatory models but with decreased side effects compared with dexamethasone [14, 16, 78].
Although some of these compounds possess anti-inflammatory properties with reduced side effects, their actual potential is still a matter of debate. The rationale on which SEGRAs are based appears to be a misinterpretation of the GC mechanism of action. Indeed, one of the pivotal mechanisms that explains the powerful anti-inflammatory effects of GCs are the very rapid, GRE-dependent upregulation of inhibitory factors, such as GILZ [3], IκB [68], annexin-1 [55], and DUSP-1 [68]. Moreover, sequestration plays a role in adverse effects. For example, RUNX2, a transcription factor crucial for osteoblastic differentiation, heterodimerizes with activated GR and is thus inhibited [40].
A deeper understanding of the molecular mechanism of action of GCs is required to dissect the pathways of GC beneficial and adverse effects, both in healthy and diseased tissues [50].
Long-Circulating Liposomal GCs and Other Reformulations of Old GCs
Another attempt to increase the therapeutic index of GCs and to target them to the site of inflammation is represented by small-sized, long-circulating liposomal GCs (less than 150 nm). These were initially formulated in topical form for dermatological purposes, but were subsequently used for intravenous administration. In this way, long-circulating liposomal GCs accumulate in ultra-high concentrations at sites of inflammation in experimental rodent models of arthritis. However, free GCs are also observed, thus causing undesirable side effects [73]. Recently, the results of the first phase I study of 22 patients with active rheumatoid arthritis (RA) who were treated with liposomal prednisolone were reported [69]. The results are encouraging and show good efficacy and safety in these patients. Clearly, further preclinical and clinical studies are necessary before determining the usefulness of small-sized long-circulating liposomal GCs.
Modifications of existing GCs can improve the daily lives of patients with RA. Morning stiffness compels these patients to take GCs during the night. New modified-release prednisone tablets have recently been developed that permit bedtime administration with programmed GC release during the night [21]. Another very recently modified GC is hydrocortisone. Under a multiparticulate oral formulation, it can mimic the diurnal cortisol profile in patients with adrenal insufficiency [76]. Loaded in nanoparticles, hydrocortisone can achieve prolonged drug release in the treatment of atopic dermatitis [62]. Thus, it is possible to modify the molecular structure or formulation of existing GCs to improve their pharmacokinetics and better treat inflammatory chronic diseases or replace endogenous hormones.
Intersubjective Variability in the Response to GCs
Genetic Resistance to GCs
GC activity depends on GR activation. Because a portion of the population fails to respond to GC treatment, studies have been conducted to identify polymorphisms in the NR3C1 gene encoding GR.
The Tth111I polymorphism is located in the NR3C1 promoter and was found to be associated with GC resistance in the presence of an ER22/23EK mutation [an arginine (R) to lysine (K) change at position 23] in exon 2. Indeed, ER22/23EK changes the tertiary structure of the GR domain, which is responsible for activation of transcription of GR mRNA. As a result, the GRα-A/GRα-B ratio changes, thereby decreasing gene transactivation. Another example is the GRβ polymorphism A3669G in the 3′-UTR of NR3C1, which causes an increased expression of GRβ protein owing to enhanced stability of GRβ mRNA. As explained in another chapter of this book, GRα is the fully active isoform, whereas GRβ is unable to bind any GCs and is transcriptionally inactive [27, 53]. Recently, a polymorphism in the promoter region of glucocorticoid-induced transcript-1 gene (GLCCI1), a protein of unknown function, was found to be correlated with reduced lung response in patients with asthma who inhaled GCs [72].
Therefore, individual heterogeneity to endogenous or exogenous GCs should be taken into account when administering GCs. Moreover, polymorphisms may be predictive of GC response, thus helping to tailor appropriate GC therapies.
Acquired Resistance to GCs
Many patients develop resistance to GC therapy during chronic inflammation, with an overall decrease in the maximum response to GCs. Because GR acts in a very complex manner and modulates expression and function of a great number of proteins, there are multiple mechanisms that can alter the pharmacological response to GCs during the development of inflammation, including different levels and function of target proteins. Moreover, the acquired resistance of inflamed tissues to GC has been postulated to correlate with a potentially low receptor reserve.
In studies in cell lines, GC resistance depended on variations in the expression of chaperone proteins such as FKBP51 and FKBP52 [64]. In patients with asthma, many molecular mechanisms correlate with GC resistance, including impaired GR nuclear translocation, decreased GR levels, increased GRβ expression, or increased AP-1 expression, which is hypothesized to impair GR function [37]. In GC refractory asthma, reduced HDAC2 expression correlates with decreased recruitment of GR to DNA sites [31]. In patients with systemic lupus erythematosus (SLE), TLR7 and TLR9 activation in plasmacytoid dendritic cells is involved in GC-induced resistance [28]. In GC-resistant ulcerative colitis, increased expression of cytokines such as tumor necrosis factor-α or interleukin-6 downregulates GR [33]. Finally, macrophage migration inhibitory factor expression has been proposed to be a common factor involved in GC-resistant inflammatory diseases, including SLE, asthma, and RA [77].
Cancer cells can be very sensitive to GC-induced apoptosis. However, they often develop resistance during chronic treatment. Some lymphoid malignancies, particularly acute lymphoblastic leukemia, can be efficiently treated with GCs because of their sensitivity to apoptosis. Because of the multiple complex mechanisms of GC action, how GCs induce apoptosis in these cells is still under investigation. One of the most recent explanations of GC resistance is site-specific phosphorylation of GR and decreased GR potency due to an altered epigenetic state in a lymphoblastic cell line. Treatment with a compound that causes DNA methylation restored GC sensitivity [46]. Other mechanisms involved in GC resistance include GR mutations and GRβ upregulation, which have been found in patients with leukemia and in cell lines. Additionally, modulation of expression of Bcl-2 family members, which are physiological targets of GCs in lymphoblasts, causes resistance to GC therapy in vivo [66].
Intracellular and extracellular signals can induce GC resistance; therefore, it is difficult to find appropriate treatments to overcome resistance once it occurs. Some attempts have been successful in preclinical studies but the complexity of GR signaling makes recovery of GC sensitivity difficult to accomplish.
GCs and Gender
GCs have recently been found to act differentially in male and female animals. In these studies, rat liver was studied as a classic target of GC action and, interestingly, unique profiles of GC-regulated genes were observed between the sexes. Among the pathways differentially altered by GC treatment in a gender-specific manner are innate immunity pathways, such as interferon or endoplasmic reticulum signaling, which account for sex-specific inflammatory stress responses. In addition, in vivo experiments showed gender-specific anti-inflammatory actions of GCs. This agrees with data showing that some chronic inflammatory autoimmune diseases (RA, SLE) occur predominantly in female patients. In addition, male and female patients respond to inflammation differently [23]. Accordingly, GC-controlled gene expression in liver is differentially regulated because GCs primarily repress genes in male and induce genes in female animals in a sexually dimorphic manner. Therefore, GC therapies should be reexamined while simultaneously taking into account that male patients respond better to anti-inflammatory treatments than their female counterparts do [59].
Molecular Basis of GC Interactions
Considering the remarkable number of effects GCs exert on various cell types in an organism, it is not surprising that molecular interactions with other drugs exist. From a clinical point of view, the most interesting is the positive interaction between GCs and β2-agonists, and GCs and anti-muscarinic agents (section “Synergism in the treatment of asthmatic patients”). However, some interactions are deleterious, such as those observed in patients with diabetes, glaucoma, and hypertension. There are also pharmacokinetic interactions to consider (section “Pharmacokinetic interactions”).
Synergism in the Treatment of Asthmatic Patients
It is well known that prolonged treatment with high doses of β2-adrenergic agonists causes receptor desensitization. Several years ago, studies in animal models suggested that desensitization was prevented by treatment with GCs [43, 63]. In vitro studies demonstrated that GC-dependent lack of downregulation depends on the upregulation of β2-receptors, independent of β2-receptor triggering [43, 79]. This effect was more recently demonstrated in humans [30]. Moreover, it has been shown that β2-adrenergic agonists can regulate GR transactivation activity by mechanisms that remain to be defined but that involve G-protein β-γ subunits and PI3-K [67].
These findings led to the evaluation of the association of β2-adrenergic agonists and GCs in the treatment of asthma. Recently, combinations of long-acting β2-agonists (LABAs, such as formoterol) and inhaled new topical GCs (e.g., mometasone furoate, and fluticasone) have provided definitive evidence proving the greater efficacy of combination drugs compared with the individual components in patients with asthma [9, 45].
Furthermore, the addition of a long-acting anti-muscarinic agent to an inhaled GC is as effective as LABA/GC combinations in patients with both asthma and chronic obstructive pulmonary disease [56]. In addition to clinical data, synergistic pharmacokinetic actions of LABAs and GCs have been suggested [49].
Pharmacokinetic Interactions
GCs can impact drug metabolism by altering expression of drug-metabolizing cytochromes P450 (CYPs). The mechanisms by which this occurs are complex and include regulation of CYP expression by nuclear receptors, which are directly modulated by GCs. Dexamethasone has been reported to upregulate hepatic CYP3A1, thus increasing CYP3A-dependent erythromycin demethylation [22]. In addition, GC-mediated upregulation of transcription factors such as CAR, PXR, and RXR2 and binding of activated GR to the GRE induces upregulation of CYP2C [10]. Moreover, CYP2B isozymes can be induced by GCs in liver. Variations in GC concentrations are another critical factor that either upregulates or downregulates CYP: CYP2A and CYP2C are induced at low GC concentrations but inhibited at high GC concentrations [39].
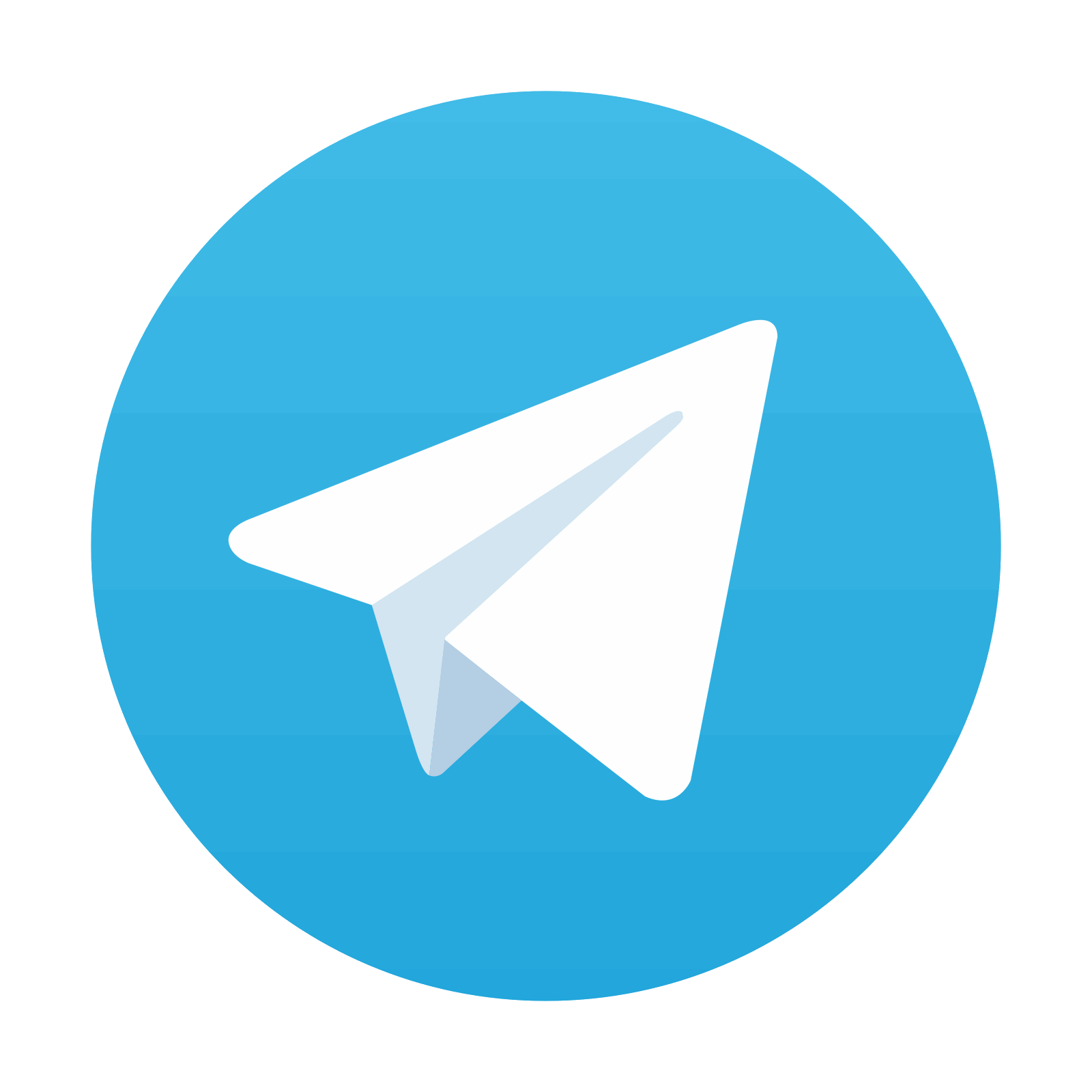
Stay updated, free articles. Join our Telegram channel

Full access? Get Clinical Tree
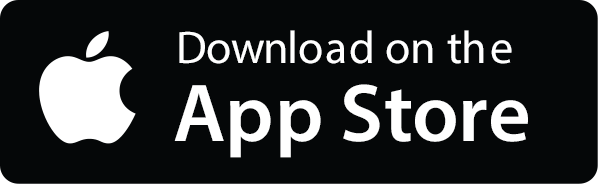
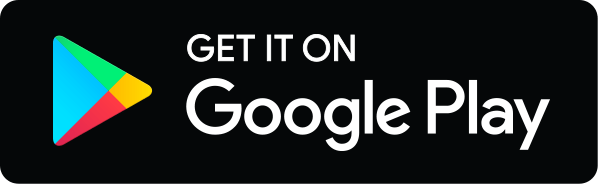