and Lilliana Ciotta1
(1)
Obstetrics and Gynecological Pathology, P.O. “S. Bambino”, University of Catania, Catania, Italy
Keywords
Menstrual disordersAnovulationInfertilityHirsutismAcneAndrogenic alopeciaHypertensionDiabetesDyslipidemiaObesityNAFLDGestational diabetesPregnancy-induced hypertensionOocyte qualityRecurrent pregnancy loss3.1 Endocrine Aspects of PCOS
Polycystic ovary syndrome (PCOS) is a chronic and self-perpetuating endocrine disorder, whose clinical, endocrine, and metabolic manifestations affect the whole life course of a patient. In PCOS, in fact, we can distinguish two sides of the same coin: endocrine and metabolic aspects.
A polycystic ovary appears enlarged with a thickened albuginea that has a porcelain appearance. In the subcapsular layer, there are many follicles measuring 2–10 mm in diameter, reduced number of granulosa cells, and a characteristic theca cell hyperplasia.
Thus, the fundamental abnormality is the presence of a raised number of follicles recruited with primary maturation block and increased atretic follicles.
3.1.1 Endocrine Pattern
3.1.1.1 Gonadotropins
PCOS is considered a normo-gonadotropic normo-estrogenic anovulatory disorder, but it is characterized by elevated LH serum concentrations with an inverted FSH/LH ratio [1].
PCOS follicles are present in large numbers, but they are arrested at an early to mid-developmental state and fail to mature even when they are exposed to normal FSH levels [2–4]. On the other hand, FSH levels do not increase during the early follicular phase to stimulate follicular maturation [5].
The resulting low estrogen and progesterone levels do not produce a negative feedback on LH secretion, and this is the major cause for the high serum LH concentrations in women with PCOS [6].
3.1.1.2 Sex Hormones
Hyperandrogenemia is the biochemical feature of PCOS. Elevated circulating androgen levels are observed in 80–90 % of women with oligomenorrhea [10].
In particular, a decreased SHBG (sex hormone-binding globulin) production with a consequent increase in free testosterone levels is reported. Furthermore, some authors suggest that, vice versa, SHBG levels are decreased in PCOS due to the effects of testosterone and insulin of decreasing hepatic production of SHBG [11, 12].
Ovaries are the main sources of increased androgens in PCOS, but even adrenal androgen excess is a common feature of the syndrome (approximately 20 % of PCOS women): an increased secretion of adrenocortical precursor steroids basally and in response to ACTH, such as pregnenolone, 17-hydroxyprogesterone (17-OHP), dehydroepiandrosterone (DHEA), and androstenedione (A), was demonstrated [13, 14].
It has been suggested that androgens enhance apoptosis in the granulosa cells of preantral and early antral follicles [15]. Moreover, a study found that the exposure to excessive androstenedione stimulates a premature luteinization of granulosa cells, most likely due to the loss of communication between the oocyte and the granulosa cell [16].
Due to the pulsatility of LH, only one blood parameter is not enough for the PCOS diagnosis, and there is no unanimous consensus on which androgen blood levels should be considered for a precise diagnosis (total or free testosterone, testosterone/SHBG ratio, or androstenedione). Usually, elevated levels of only DHEA or 17-OHP may exclude the diagnosis of PCOS [17].
3.1.1.3 Estrogens and Progesterone
Estradiol levels are constant, without the normal mid-cycle increase, while the levels of estrone are increased because of extraglandular aromatization of increased circulating androstenedione levels [18–20].
As a consequent of anovulation, progesterone levels are low in PCOS women; moreover, some authors reported that endometrial responsiveness to progesterone is reduced in PCOS women [21, 22] and that total endometrium PR (progesterone receptor) expression is higher in women with PCOS who have anovulation compared to women with PCOS who still ovulate [23].
Furthermore, the increased PR expression in epithelial cells is greater than that instromal cells in women with PCOS, suggesting that lower binding of progesterone in stromal cells could lead to the promotion of estradiol-induced epithelial cell proliferation in PCOS women.
It has been hypothesized that lack of progesterone-induced and PR-mediated stromal cell proliferation could be a cause of progesterone resistance in PCOS patients [24].
3.1.1.4 AMH
Anti-Mullerian hormone (AMH) belongs to the transforming growth factor-β (TGF-β) superfamily. In women, AMH is produced by the granulosa cells of follicles from the stage of the primary follicle to the initial formation of the antrum. In female newborn, AMH is undetectable, but it increases gradually until puberty, remaining stable in the reproductive period [25].
Reduction of AMH levels in serum is the first indication of a decline in the follicular reserve of the ovaries. Moreover, AMH concentration remains stable during the cycle [26].
Since AMH level reflect the number of developing follicles, its measurement may be used as a marker of ovarian follicle damage in PCOS.
AMH levels are also probably related to the follicular arrest, during the selection process of the dominant follicle: AMH inhibits the recruitment of primordial follicles into the pool of growing follicles and decreases their receptiveness to FSH [27–29].
The first studies regarding AMH levels in PCOS women showed that AMH levels are higher than in healthy controls [30, 31]. Subsequent data indicated that these levels are related to increased number of small antral follicles of 2–5 mm diameter [32]: this correlation was found to be the strongest one [33].
The cause of the increased AMH production in PCOS is unknown: it is mainly ascribed to the increased production of AMH by each follicle, and it is not just a consequence of an increased follicle number, suggesting intrinsic granulosa cell dysregulation in PCOS [34, 35].
AMH levels are increased in proportion to PCOS clinical severity, as reflected by the antral follicle count [36, 37].
Furthermore, blood AMH appears to be associated with androgen levels, and so it has been proposed as a diagnostic marker for ovarian hyperandrogenism [38].
Some studies demonstrated, in fact, that AMH is positively correlated with total testosterone levels in normal-weight PCOS women [39].
AMH levels, as written before, decrease with age in women with normal ovulatory cycles; in PCOS women, this decline has a slower reduction rate, and it could be because of a decelerated ovarian aging, probably due to the negative effect of AMH on the recruitment of primordial follicles.
3.1.2 Clinical Endocrine Features
The clinical scenario of PCOS is very heterogeneous, and the symptoms are related to the ovarian dysfunction and hyperandrogenism.
This section describes the clinical characteristics of a PCOS woman, while the diagnostic pathway can be found in Chap. 6.
3.1.2.1 Menstrual Disorder
Since menarche, or after a short period, menstrual cycles show an irregular rhythm. In many cases they gradually distance themselves from each other, up to result in oligomenorrhea or in permanent amenorrhea. Menstrual dysfunction in women affected by PCOS may manifest in different ways, but the most common way is anovulation with erratic bleedings.
Although the presence of oligomenorrhea indicates ovulatory dysfunction, apparent eumenorrhea does not completely rule out anovulation [40].
Therefore, ovarian dysfunction usually manifests as oligomenorrhea/amenorrhea resulting from chronic oligo-ovulation/anovulation. The majority of women complaining oligomenorrhea (up to 80–90 %) are affected by PCOS [41].
A significant relationship between the degree of menstrual dysfunction and the degree of insulin resistance present was observed. After adjusting for BMI, age, and race, all PCOS subjects with menstrual cycles longer than 35 days had significantly higher mean HOMA-IR levels than controls, with those with cycle length longer than 3 months having the highest one [42]. Confirming these findings, it was reported also that among PCOS women insulin resistance was significantly worse in amenorrheic patients [43].
As consequent, prolonged anovulation can be the cause of dysfunctional uterine bleeding, which may mimic regular menstrual cycles.
In addition, the chronic anovulation implies prolonged estrogen excess (particularly in obese phenotype women) and lack of progesterone, resulting in atypical endometrial hyperplasia, which is the precursor of endometrial carcinoma [44, 45].
It is generally recommended that greater than four cycles per year may protect the endometrium [46].
3.1.2.2 Infertility
PCOS is the most common cause of anovulatory infertility: 90 % of women attending infertility clinic for anovulation disorder are affected by PCOS.
Despite these data, 60 % of women with PCOS are fertile, while time to conceive is often increased [41].
Moreover, infertile PCOS women are overweight in 90 % of cases.
Fifty percent of PCOS women experience recurrent pregnancy loss [47]: it is not clear whether these defects are caused by uterine dysfunction itself, by possible interrupted interaction between uterine cells and the developing embryo, or by insulin-related disorder.
The new guidelines suggest that PCOS is a risk factor for infertility only in the presence of oligo-ovulation or anovulation. However, there are no clear data about the fertility of PCOS patients who have normal ovulatory function [48].
3.1.2.3 Hirsutism
Hirsutism is defined as the presence of excessive terminal hairs in areas of the body that are androgen dependent and usually hairless or with limited hair growth, such as the face, chest, areolas, and abdomen [49].
Terminal hair is different from “vellus” hair, because the latter is the prolonged version of “lanugo” (the hair that covers fetuses and is shed gradually after birth) which covers all body surface except lips, palms, and soles; specifically, terminal hair is the pigmented, longer, coarser hair that covers the pubic and axillary areas, scalp, eyelashes, eyebrows, male body, and facial hair [50].
Hirsutism should be differentiated from hypertrichosis, which is the overgrowth of vellus in a nonsexual pattern distribution, usually related to persistence of the highly mitotic anagen phase of the hair cycle [51, 52].
Terminal hair growth requires androgen stimulation, specially testosterone and dihydrotestosterone (DHT) that can bind to the androgen receptor and promote hair follicle changes [50, 53].
Androgens, in fact, are the most significant hormones associated with hair growth modulation. They are necessary for terminal hair and sebaceous gland development and cause differentiation of pilosebaceous units into either a terminal hair follicle or a sebaceous gland. They are involved in keratinization, increased hair follicle size, hair fiber diameter, and the proportion of time that terminal hair spends in the anagen phase [54].
Thus, hyperandrogenemia is the cause of hirsutism, but the percentage of hair growth is not proportional to the degree of hyperandrogenism, supporting the important role for androgen receptor localization (keratinocytes, sebaceous glands, hair dermal papilla cells) and sensitivity in the development of hair patterns [55].
3.1.2.4 Acne and Seborrhea
Sebaceous glands are also androgen-dependent structures: sebocytes are highly sensitive to androgen signaling, which is worsened in PCOS, leading to the development of acne and seborrhea [56].
Androgens stimulate sebocyte proliferation (particularly in the mid-back, chin, and forehead) and secretion of sebum, which is a mixture of lipids including glycerides, squalene, free fatty acids (FFA), and cholesterol [57].
Local bacteria complicate the process by secreting lipolytic enzymes: they break down those triglycerides produced in the sebocyte; these FFAs are released into sebaceous ducts by apocrine glands, and they are responsible for the typical unpleasant odor [58].
3.1.2.5 Androgenic Alopecia
An opposite clinical feature is androgenic alopecia, which is a disorder characterized by miniaturized hair, due to an increased telogen/anagen ratio, and associated to genetic susceptibility related to increased 5α-reductase activity in the hair follicle. This increased enzymatic activity promotes the local conversion of testosterone into DHT, which has an increased androgen action.
3.1.2.6 Other Clinical Features
In rare cases, virilization patterns can be observed: they include increased size of clitoris, muscle mass hypertrophy, deep voice, temporal balding, and masculine aspect. In these cases, however, a lower ovarian or adrenal androgen-secreting neoplasm must be excluded.
Moreover, in PCOS women, nails could be affected by alterations, in the form of onycholysis [61] (separation of the nail plate from the nail bed caused by disruption of the onychocorneal band) and onychorrhexis [62] (splitting of nails in lengthway bridges). Nowadays the association of these nail diseases with hyperandrogenemia is not completely understood.
In literature, an unusual case of atypical oral hirsutism secondary to PCOS was described: a 19-year-old girl complained of the appearance of hairs on the sulcular epithelium of the retroincisor palatal papilla, relapsing after surgical excision. PCOS diagnosis was confirmed by clinical data (oligomenorrhea, face hirsutism, and acne), by serum studies, and by the symptom improvement after combined hormonal therapy [63].
3.1.2.7 PCOS and Thyroid Dysfunction
The most prevalent autoimmune disease in women is autoimmune thyroiditis (AIT), with a prevalence ranging from 4 to 21 %: it depends on age [64], diagnostic criteria, genetic differences, geographical origin, and iodine intake [65, 66]. In the past, a German study underlined the association between PCOS and AIT, but the pathogenesis of this relationship is not clear.
Few explanations were suggested, but none of these appears to be conclusive:
-
Probable common genetic predisposition
-
Imbalance between estrogens and progesterone, and the consequences of the stimulatory effect of estrogens on the immune system [67]
-
Low-grade inflammatory state characteristic of PCOS
Recently, it has been shown that there is a higher prevalence of subclinical hypothyroidism in young women with PCOS compared with that reported for the general young women population [68].
Moreover, Mueller et al. [69] observed that PCOS patients with subclinical hypothyroidism had a higher prevalence of IR and a higher BMI.
3.2 Metabolic Aspects of PCOS
“Metabolic flexibility” is the capacity of the body to rapidly switch from predominant lipid oxidation (with high rates of fatty acid uptake in low-insulin conditions) to predominant glucose oxidation and storage with suppression of lipid oxidation in high-insulin conditions [70].
Few studies showed that obese and/or diabetic and/or insulin-resistant individuals, as compared with healthy lean individuals, have an impaired metabolic flexibility [71, 72].
Insulin resistance and the associated metabolic abnormalities are frequent findings in women with polycystic ovary syndrome [73].
Many women with PCOS meet the criteria for the metabolic syndrome (MS), as they report a higher incidence of hypertension, dyslipidemia, and visceral obesity [74].
Up to 43 % of nondiabetic PCOS women meet MS criteria before the end of their fourth decade, and most of them before the end of their third decade of life [75, 76].
This prevalence is four times higher than that observed in women aged 20–30 years and twice that of women between the ages of 30 and 40 years [77].
The prevalence of metabolic syndrome is similar across racial backgrounds [78].
Moreover, it was found that the prevalence of metabolic syndrome is higher in adolescent girls with PCOS: 37 % against 5 % of control non-PCOS girls [79].
The most common phenotypes in parents of adolescents with PCOS were found to be excessive weight and metabolic syndrome, particularly in fathers in whom the prevalence of MS and central obesity was 1.5–2-fold greater than expected in the general population [80].
The essential components of MS include insulin resistance or central obesity with at least two of hypertension, elevated triglycerides, decreased HDL-C levels, or elevated fasting glucose [81].
Insulin resistance, and consequent compensatory hyperinsulinemia, appears to be the central pathophysiologic mechanism that links PCOS to its metabolic disorders; in fact, few studies reported that PCOS women are more insulin resistant than controls who are matched for age and BMI [78].
Disturbance in the insulin’s ability to bind to its receptor or in the transport mechanism across the cell membrane may lead to a state of a reduced sensitivity to insulin, or insulin resistance. Furthermore, pancreatic β-cell secretory dysfunction has also been reported [82, 83], and a reduction in hepatic insulin extraction contributes to the high insulin levels as well [84, 85].
Compensatory hyperinsulinemia is important in the development of metabolic abnormalities and also contributes to the high androgen levels, peculiar of PCOS women. As largely described in Chap. 2, it is important to remember that insulin binds to its receptor on the ovarian theca cell and it acts enhancing LH-stimulated androgen production [86]. Moreover, insulin can also act indirectly to raise free testosterone serum concentration by inhibiting the hepatic production of SHBG [12].
Although obesity is a major factor for the development of insulin resistance in PCOS, it is now well known that a component of insulin resistance is independent of body weight [87].
To underline the link between PCOS and metabolic syndrome, it is important to report that coronary heart disease, as well as cerebrovascular disease, is more common in postmenopausal PCOS patients. Persisting high androgen levels through the menopause, obesity, and maturity-onset diabetes mellitus are proposed as the main mechanisms accounting for the increased risk [88].
3.2.1 The Role of the Adipocyte in Linking PCOS to Metabolic Syndrome
Adipose tissue is nowadays considered not only a storage tissue but also a proper endocrine organ, metabolically active [89–92].
Adipose tissue responds to chronic changes in energy balance and nutrient content by altering the proliferation of pre-adipocytes, their differentiation into mature adipocytes, the growth and hypertrophy of adipocytes, and, finally, their apoptosis and necrosis [93]. In addition, rates of angiogenesis, extracellular matrix remodeling, and the relative distribution of the resident immune cell population in adipose tissue are modified in response to changes in nutritional status [94]. Thus, it is evident that adipose tissue acts as an enormous endocrine organ, secreting a variety of signaling molecules that regulate feeding behavior, energy spending, metabolism, reproduction, and endocrine and immune function [95].
Adipocytes secrete adiponectin, leptin, visfatin, tumor necrosis factor alpha (TNF-α), interleukin 6 (IL-6), plasminogen activator inhibitor-1 (PAI-1), resistin, and angiotensinogen: thus, adipose tissue results to be metabolically active [96, 97].
Adiponectin is a 244-amino acid protein that is expressed in white adipose tissue [98].
This adipokine expression within adipocytes is downregulated in obesity [99], and the result is that serum levels of adiponectin are inversely correlated with body weight [100].
Adiponectin has insulin-sensitizing, anti-atherogenic, and anti-inflammatory properties [101, 102]. It is well known that adiponectin has an important role in mediating the effects of increased fat mass on insulin sensitivity [103]: in fact, its low serum levels seem to be involved in conditions associated with insulin resistance, such as type II diabetes and obesity [104–106]; moreover, lower levels of serum adiponectin are present in PCOS women [107].
It also has been reported that adiponectin inhibits theca cell androgen production: suppressed levels of adiponectin may allow enhanced ovarian androgen production in PCOS women [108].
Leptin controls the fat disposition modulating its accumulation in the heart, liver, and kidneys; besides, it is involved in the control of vascular tone by producing a pressure action and opposing the NO-mediated relaxing function [109]: this could be associated with cardio-metabolic syndrome.
In humans, there is a strong association between the percentage of body fat and serum leptin levels [110]; some authors found that hyper-leptinemia has a positive relationship with insulin-resistant PCOS women [111], even if more studies are needed to confirm it.
Hyper-leptinemia seems to lower the sensitivity of dominant ovarian follicles to insulin-like growth factor 1 (IGF-1), which is implicated in the mechanism of anovulation [112].
Visfatin is a multifunctional protein that plays a number of roles including the regulation of metabolism and inflammation, and it is also involved in the insulin resistance mechanism [113, 114].
Few recent studies have demonstrated that visfatin levels are significantly higher in PCOS women comparing to the healthy controls [115], even when considering only the overweight and obese subgroups [116].
It has been demonstrated that in women with PCOS, adipocyte diameter is 25 % greater than the diameter of adipocytes taken from obese control women with comparable BMI: adipocyte seems to be hypertrophic [117].
Adipocyte hypertrophy in PCOS may be a consequence of variations in storage and/or adipocyte lipolytic capacity. Thus, obesity in women with PCOS is mainly characterized by an increase in fat cell size (hypertrophic obesity) rather than an increase in fat cell number (hyperplastic obesity) [118].
In PCOS subcutaneous adipocytes, there is a reduced catecholamine-mediated lipolysis [119], and maybe there is an implication of testosterone as a possible contributory factor in this process [120]. Greater lipolysis within visceral adipocytes results in hepatic insulin resistance through increased hepatic influx of portal free fatty acids; reduced lipolysis within subcutaneous adipocytes is likely to be one explanation for adipocyte hypertrophy and consequent insulin resistance [108].
Studies on the molecular insulin signaling pathways within PCOS adipocytes have demonstrated that the number of insulin receptors and the affinity of these receptors for insulin are normal [121–123]. Moreover, there are evidences that, in PCOS adipocytes, basal auto-phosphorylation of the insulin receptor β-subunit is normal, but insulin-dependent auto-phosphorylation is significantly reduced [123].
The existing literature suggests a large number of possible defective post-insulin receptor molecular mechanisms that may explain adipocyte’s insulin resistance in PCOS, although the actual mechanism involved and the determinants of adipocyte size in PCOS are not fully understood [108].
In the past, Danforth hypothesized that the inability to differentiate sufficient new subcutaneous adipocytes in response to chronic excessive energy intake may explain the metabolic dysfunction observed in some obese women: thus, a deficiency in either the proliferation or differentiation capacity of adipocytes leads to the redistribution of fat from subcutaneous to visceral depots and also to other tissues such as the liver and skeletal muscle, where the ectopic fat causes insulin resistance [124].
Furthermore, steroidogenic activity within the adipocyte plays a crucial role in the development of PCOS, particularly the hyperandrogenemia associated with PCOS: this could be the link between obesity and hyperandrogenemic features [108].
Although the predominant source of raised androgens in PCOS women is ovarian, adrenal androgen secretion is also important [125]; moreover, peripheral conversion of androstenedione and DHEAS accounts for up to 50 % of circulating testosterone in PCOS women, and the major peripheral site is the adipose tissue [126].
The 5α-reductase enzyme is present in the adipocyte cell and it converts testosterone into the more potent 5α-dihydrotestosterone (DHT), and it is also involved in the catabolism of cortisol [125].
It is supposed that PCOS women have enhanced peripheral 5α-reductase activity compared with age and BMI-matched control women [127–130]. This causes an increased production of DHT, increased catabolism of cortisol, and consequent reduced feedback of cortisol on the pituitary corticotroph cells [125].
Furthermore, increasing adipose tissue mass is directly associated with increasing levels of angiotensin II from the increased secretion of angiotensinogen by adipose tissue; this increase could contribute to hypertension and worsen insulin resistance [131].
On the other hand, few molecules secreted by adipocytes are involved in macrophage function, including monocyte chemoattractant protein-1 (MCP-1), macrophage migration inhibitory factor, and macrophage inflammatory protein (MIP)-1α which are upregulated in obesity [132]. Adipose tissue resident macrophages from obese women are activated and also express few proteins such as MIP-1α, MCP-1, and related inflammatory cytokines, which may play a role in the development of obesity-induced insulin resistance [118]. Most adipose tissue TNF-α, inducible nitric oxide synthase, and IL-6 seem to be expressed by adipose tissue macrophages, rather than adipocyte [94]. IL-6 inhibits lipoprotein lipase activity, stimulates aromatase activity, and increases the hepatic production of triglycerides [133]. IL-6 is stimulated by TNF-α: the latter stimulates C-reactive protein (CRP), which is highly associated with obesity, insulin resistance, and endothelial dysfunction; PCOS women seem to have higher levels of CRP [134, 135] and, specifically, of hs-CRP (high-sensitive CRP), which is the most specific marker [136].
Elevation of these inflammatory markers is in accord with the hypothesis that atheroma formation is primarily an inflammatory condition [137].
3.2.2 The Role of Vitamin D in the Development of Metabolic Syndrome in PCOS Women
Few evidences suggest that vitamin D deficiency could be a causal factor in the pathogenesis of metabolic syndrome in PCOS women [138].
It is well known that the vitamin D receptor gene regulates 3 % of the human genome, including genes essential for glucose and lipid metabolism and blood pressure regulation [139–141].
In fact, clinical studies had reported insulin resistance and obesity association with hypovitaminosis D [138, 142, 143].
The mechanism underlying the association between low vitamin D levels and insulin resistance is not completely assumed. The suggested hypotheses are the following:
-
Vitamin D may have a positive effect on insulin action by stimulating the expression of insulin receptor and improving insulin receptiveness for glucose transport [140].
-
Vitamin D regulates extracellular and intracellular calcium, which is important for insulin-mediated intracellular processes in insulin-responsive tissue (skeletal muscle and adipose tissue) [140].
Moreover, it is not fully understood whether vitamin D insufficiency results from obesity and/or whether obesity is a consequence of hypovitaminosis D.
Despite there is not a clear consensus regarding its optimal value, a level of 30 ng/ml indicates a sufficient vitamin D status [139]; concentrations of 20–30 ng/ml are considered as vitamin D insufficiency, while a level less than 20 ng/ml represents a vitamin D deficiency [139].
In a recent study, 72.8 % of PCOS women showed values below the abovementioned normal cutoff; a significant association of hypovitaminosis with increased levels of both fasting and stimulated glucose and insulin, elevated HOMA-IR, and incidence of MS was demonstrated [146].
Furthermore, vitamin D levels were significantly lower in hirsute woman, as shown in previous study [138, 147]. For example, distress caused by the excessive hair might lead to hypovitaminosis D because of the decreased sun exposure of hirsute women.
Vitamin D receptor is present in keratinocytes of the outer root sheath as well as in cells of the bulge, indicating an important role of vitamin D in hair follicle cycling [144].
3.2.3 Metabolic Syndrome and Associated Disorders
3.2.3.1 Visceral Obesity
A likely explanation for the mechanism underlying the development of obesity in women with PCOS is the combined effect of a genetic predisposition to obesity in the context of an “obesogenic” environment (poor diet and reduced exercise). The development of obesity in PCOS patients, in turn, amplifies and unmasks the biochemical and clinical abnormalities characteristic of this condition [125].
Obese PCOS women have lower levels of SHBG, DHEAS, DHEA, IGF-1, and HDL and higher LDL compared with the nonobese PCOS controls [150].
It is important to underline that all overweight/obese people are not insulin resistant, and those who are insulin resistant are not all obese.
Previous researches have studied the importance of fat distribution patterns as risk factor for cardiovascular and metabolic disease such as diabetes mellitus [151, 152].
In fact, gluteo-femoral obesity is less associated with insulin resistance than is central or android obesity [153].
The gynoid type of fat distribution, where fat accumulates around the hips, thighs, and buttocks, is developed during female puberty and is maintained during the fertile phase [152, 154].
Approximately 50–60 % of PCOS women are characterized by a so-called “android” distribution of body fat, whereby a disproportionate quantity of adipose tissue is distributed in the visceral depot [155, 156]: they have a higher trunk/peripheryfat ratio [157].
This upper body fat distribution has been explained mainly by androgen excess [158], and it is an independent factor of BMI [159]: the pathogenic mechanisms involved have not yet been defined.
In women affected by PCOS, android body fat distribution per se contributes to hyperandrogenemia, through its adverse effects on insulin sensitivity and consequent ovarian co-gonadotropic effects of hyperinsulinemia.
Hyperinsulinemia itself contributes to obesity by the anabolic effect on fat metabolism through the adipogenesis process: the result is an increased uptake of glucose into adipocytes, the production of triglycerides, and the inhibition of hormone-sensitive lipase [160].
Therefore, there is a vicious cycle in which android fat produces android fat and exacerbates the predisposition toward weight gain [125].
Visceral fat, or abdominal fat, is metabolically distinct from subcutaneous fat; it is resistant to the anti-lipolytic effects of insulin and releases excessive amounts of free fatty acids, which leads to IR in the liver and muscle. In response to it, in the liver, there is an increased gluconeogenesis, and in the muscle there is an inhibition of insulin-mediated glucose uptake [161–163].
Excess fat itself contributes to IR at the level of the adipocyte: when fat cells become too large, they are unable to store additional lipids, and then, fat is stored in the muscle, liver, and beta cells of the pancreas [164]. Visceral fat also produces excess of 11-beta-hydroxysteroid-dehydrogenase-1 [163], an enzyme that converts inactive cortisone to the biochemically active cortisol: the latter is able to promote central adiposity and IR [165].
3.2.3.2 Dyslipidemia
The probability of a metabolic disorder in families of PCOS patients is 2.7-fold higher compared with normal families, and the relative risk for developing dyslipidemia is 1.8 [166].
Dyslipidemia is reported in up to 70 % of patients who have PCOS, according to the National Cholesterol Education Program (NCEP) guidelines [167].
Dyslipidemia in PCOS women seems to be well understood, but which are the determinant factors of this pattern? Insulin, estrogens, and androgens are each well known to alter lipoprotein lipid metabolism [168]. All of them influence hepatic lipase activity, which is important in reductive metabolism of intermediate-density lipoproteins to small dense LDL particles; greater activity of this enzyme was found in PCOS women [169].
Insulin stimulates lipogenesis in arterial and adipose tissues via an increased production of acetyl CoA and the entry of glucose and triglycerides [170].
PCOS women have higher Apo-CIII levels compared to non-PCOS controls [171]: understanding its metabolism is helpful to deeply comprehend the pathophysiology of dyslipidemia in PCOS.
In states of IR, it has been shown an increased synthesis of ApoC-III [172]: in PCOS, with central obesity more free fatty acids flow into the portal vein and more glucose is available, causing altered apolipoprotein lipid metabolism. The ratio of ApoC-II/CII is increased and triglycerides carried in VLDL are broken into more atherogenic small LDL particles, which circulate and enter the arterial wall to initiate inflammation. With elevated triglycerides, VLDL lipolysis is slowed, causing greater residence time for ApoB, remnant particles, LD particles and small LDL-particles. ApoC-I, recently shown to be elevated in normal-weight PCOS women, blocks lipoprotein lipase, cholesterol ester transferase, lecithin cholesterol acyltransferase, VLDL receptors and LDL receptors in the liver. All of these events lead to more exposure of the blood vessel wall to entry of atherogenic particles with the potential for setting inflammation and atherogenesis. [168]
Moreover, insulin increases the levels of HMG-CoA reductase, the rate-limiting enzyme in the synthesis of cholesterol: this effect may contribute to the raised cholesterol level, which is also a feature of hyperinsulinemia [173].
Hyperandrogenism and lipid metabolism are closely related: in fact, it has been observed that testosterone has a deleterious effect on lipid profile [174, 175]. Testosterone has been involved in lowering HDL-C levels, an effect attributed to the upregulation of two genes implicated in the catabolism of HDL: scavenger receptor B1 (SR-B1) and hepatic lipase [176].
The most common lipid profile found in PCOS individuals is characterized by [176–182]:
-
Increased levels of LDL cholesterol (especially raised amounts of types III and IV small LDL particles [176])
-
Increased VLDL cholesterol
-
Increased triglycerides
-
Reduced levels of HDL cholesterol (particularly decreased HDL2, the most anti-atherogenic HDL subtype)
Particularly, a study has demonstrated that the incidence of high triglycerides increased progressively from the lean to the obese PCOS women, and the incidence of low HDL was three times higher in the overweight than in the lean PCOS subgroup [183].
HDL-C has several functions: inhibition of LDL-C oxidation, transport of cholesterol from peripheral cells to the liver, anti-apoptotic effects, and antithrombotic and antioxidant effects. For this reason, low HDL-C is considered an independent cardiovascular risk factor: thus, women with PCOS may have a higher cardiovascular risk than normal women at the same BMI level [184].
Furthermore, these lipid disorders are exacerbated among those women who develop glucose intolerance in association with PCOS; in fact, 88 % of women with PCOS and IGT or type II diabetes have an abnormal lipid profile, compared with 58 % of women with PCOS and normal glucose tolerance [177].
Even PCOS adolescents have less favorable blood lipid profiles, with higher LDL-C and lower levels of HDL-C, and they appear to be more insulin resistant than their peer control with higher fasting C peptide levels [185].
3.2.3.3 NAFLD (Nonalcoholic Fatty Liver Disease)
NAFLD represents a disease spectrum ranging from steatosis hepatitis (SH or NAFL) to nonalcoholic steato-hepatitis (NASH), characterized by hepatocyte injury, inflammation, and fibrosis, which can progress to cirrhosis in 25 % of cases, with its long-term complications, such as portal hypertension, liver failure, and hepatocellular carcinoma [186].
The hepatic steatosis is histopathologically characterized by the accumulation of triglycerides, both in the form of macro- and microvesicles, in more than 5 % of hepatocytes. These “fatty hepatocytes” are usually peri-venular located, and they are mainly present at the level of the “portal areas.” The pool of fatty acids available for the synthesis of triglycerides is related to the balance between their formation and utilization. The deposition of triglycerides into the hepatocytes depends on both of these reactions: thus, the development of a fatty liver is the consequence of a dysfunction in different metabolic pathways.
There are important relationships between peripheral insulin resistance and hepatic fat deposition. High intake of calories in sedentary individuals who are genetically susceptible induces a state of IR, which involves an increased lipolysis, as a result of free fatty acid and TNF-α circulating levels and of lower levels of adiponectin: this increases both insulin resistance and circulating levels of free fatty acids. The raised fat deposition in the liver induces insulin resistance by itself, activating abnormal insulin intracellular signals. Both these processes lead to an increased hepatic insulin resistance and deposition of fatty acids.
These events, in turn, cause a dysregulation of some sterol regulatory proteins (SREBP-1C) and, probably, of ghrelin. This pathogenic mechanism is responsible for inducing a de novo lipogenesis in the liver.
Thus, it is well evident that NAFLD is a complex and multifactorial disease, and it is currently the most common cause of liver disease and high enzyme levels in clinical practice. Probably a state of IR plays critical part in both the development and progression of the liver disease. It seems that an important role is assumed by oxidative stress and some adipokines, such as TNF-α and adiponectin. Moreover, the nature of the epidemic NAFLD, as well as its clear association with obesity and metabolic syndrome, makes the altered lifestyle and a sedentary lifestyle conditioning factors in the development of this disease even in teenagers [187].
Even if liver biopsy is the “gold standard” for distinguishing between simple steatosis and NASH, and for disease severity assessment, the diagnosis of fatty liver is clinically performed by ultrasound:
1.
Absent: the echogenicity of the liver parenchyma is greater than or equal to that of the cortex of the kidney; there is a clear view of the intrahepatic venous system.
2.
Mild fatty liver: slight increase of fine echoes in the liver parenchyma with normal visualization of the intrahepatic venous circulation.
3.
Moderate hepatic steatosis: moderate and widespread increase of fine echoes in the liver parenchyma with impaired visualization of intrahepatic venous system.
4.
Severe fatty liver: marked increased echogenicity of the liver parenchyma with deficiency or absence of visualization of intrahepatic venous circulation [187].
The extent of steatosis is related to the degree of insulin resistance [188].
Elevated liver enzymes have been used as a noninvasive surrogate marker of NAFLD, provided that other potential causes of liver disease (chronic viral hepatitis, alcohol-induced liver disease, etc.) have been excluded.
The typical pattern of abnormal liver biochemical profile includes increased serum aminotransferases, with a predominant increase of alanine aminotransferase (ALT), relative to aspartate aminotransferase (AST), accompanied by elevated γ-glutamyl transpeptidase levels (γ-GT) [189, 190].
Elevated ALT, above the level of 35 U/l, has been detected in 30 % of overweight/obese PCOS women [191, 192].
Both adult and pediatric patients with NASH are commonly asymptomatic. Rarely, patients may present with persistent right upper quadrant pain or chronic pain in the umbilical region. On physical examination, more than 90 % of patients with NASH are found to be obese, and acanthosis nigricans has been reported in 36–49 % of patients [193].
A number of studies have demonstrated a high risk of hepatic steatosis in women with PCOS [187, 192, 194–196].
A recent study has shown that women with hyperandrogenic PCOS have evidence of increased liver fat, compared with PCOS women with normal androgens or with healthy controls [197].
In one of our studies, an elevated percentage of NAFLD both in lean and obese women, with a low rate of hepatomegaly (8.3 %) and 15 % of elevated liver enzymes, was found. In fact, liver enzyme impairment is not always associated to the presence of NAFLD, and vice versa [187].
Moreover, in contrast to what happens in other population subgroup where only a minority of patients who suffer from NAFLD progress to NASH, the prevalence of advanced liver disease (NASH with fibrosis) in women with PCOS is higher [192, 198] even in the adolescent population [199].
For these reasons, from a clinical point of view, it seems advisable to closely follow women with PCOS and insulin resistance, particularly in the presence of body weight alterations.
3.2.3.4 Hypertension
Hyperinsulinemia may contribute to the hypertension (which is part of the metabolic syndrome) by several mechanisms:
The prevalence of hypertension in PCOS women increases with BMI as independent factor [206].
Women with PCOS were found to have an increased left atrial size and left ventricular mass index, with a reduced left ventricular ejection fraction [207], which is directly related to the degree of insulin resistance; this finding may represent early remodeling as a prelude to overt cardiac dysfunction [208].
The results of many studies are controversial: in a few of them, both systolic and diastolic blood pressures are normal [124, 209–212], while in other studies, mean arterial pressures and ambulatory systolic pressures are elevated in women with PCOS compared with non-PCOS controls [212].
PCOS patients appear to be at increased risk for developing hypertension, at least later in life if it doesn’t occur during the reproductive age.
For menopausal or climacteric women with a previous history of PCOS, this prevalence varies from 28.1 to 39 % [209, 213], while for patients who are in their third or fourth decades of life, the prevalence varies from 3.8 to 22 % [213–215].
This difference of prevalence according to the age range is probably a consequence of aging itself.
3.2.3.5 Diabetes
PCOS women are at high risk of progression to impaired glucose metabolism and type II diabetes; a family history of type II diabetes is present in a large percentage of women affected by PCOS, suggesting the important role of the genetic pattern in the development of the syndrome.
Generally, glucose levels remain normal in PCOS despite insulin resistance, because of compensatory pancreatic β-cell insulin production resulting in hyperinsulinemia. However, some patients have a genetic susceptibility to pancreatic β-cell failure and, over time, develop elevations in glucose when pancreatic β-cell insulin production can no longer overcome the insulin resistance [216].
A study reported that 35 % of patients with PCOS had impaired glucose tolerance (IGT) and 10 % had type II diabetes (T2DM) by the age of 40 [217].
A very recent study showed that the conversion rate from IGT to T2DM in women with PCOS was higher than that in the general population of women with IGT: 2–10.75 % vs 1–7 % per year [218].
Moreover, it was clearly shown that the risk for IGT or T2DM in women with PCOS was amplified (fourfold increase) in the presence of obesity, highlighting the role of patient weight in the development of glucose metabolism disorders [218].
A Korean study found that nonobese patients with PCOS presented a higher prevalence of elevated glycated hemoglobin than nonobese controls [219].
Both IGT and T2DM are very significant cardiovascular risk factors in women. Once the diagnosis of diabetes is made, the relative risk of cardiovascular disease in women increases fourfold to sevenfold, with a greater risk of cardiovascular disease and heart failure compared to men with diabetes [220].
With regard to mortality rates, diabetes may be a more prominent contributing cause of death in women with PCOS compared with the general population [221].
3.2.3.6 Obstructive Sleep Apnea
Obstructive sleep apnea (OSA) is a known cardiovascular risk factor and is one of the major causes of chronic sleep disruption. It is characterized by episodic partial or complete upper airway obstruction during sleep, leading to intermittent hypoxia, sleep fragmentation, and a reduction in the quantity of deep non-rapid eye movement (NREM) sleep or “slow wave sleep” (SWS). This alteration has been associated with cortisol levels rising [222].
OSA has been independently related to glucose intolerance and insulin resistance even after adjustments for obesity and age [223–227], and PCOS women are 5–30 times more likely to have this disorder compared to controls [167, 176, 228–231].
The mechanism by which PCOS increases the risk of OSA remains unclear.
The high prevalence of this disorder cannot be fully attributed to excess adiposity, as reported in some studies: in two studies the severity of OSA did not correlate with BMI [229–231], and in another one, even after controlling for BMI, women with PCOS were 30 times more likely to have breathing disorders during sleep and 9 times more likely to have daytime sleepiness than the control women [230].
On the other hand, according to a recent study, nonobese women with PCOS do not seem to be at increased risk of OSA: this raised risk is only present among the obese women [232]. Other studies have also indicated that obese women with PCOS who have OSA are more insulin resistant compared to obese women with PCOS who do not have OSA [231, 233, 234].
Insulin resistance, in fact, seems to be a stronger predictor of OSA, more than age, BMI, or circulating testosterone concentration [229]: the role of androgen elevation in the pathogenesis of OSA in women with PCOS remains controversial [229, 230].
Another potential pathogenic mechanism is the “low progesterone theory”: it has been estimated that the upper airway resistance is lower during the luteal phase, when usually progesterone is higher, compared with follicular phase when progesterone is low [235]. Progesterone promotes its effects through direct stimulation of respiratory drive [236] and enhancement of the upper airway dilator muscle activity [237] by which it reduces airway resistance. Because women with PCOS have usually anovulatory cycles, and so circulating progesterone concentrations reflect the constantly lower levels of the follicular phase, this may contribute to the high prevalence of OSA in PCOS [238].
3.2.3.7 Plasma Viscosity and Pro-thrombotic State
In healthy individuals, there is equilibrium between the hemostatic coagulation and fibrinolytic systems: thrombosis results from an imbalance between these complex systems [239]. The hemostatic system plays an important role in cardiovascular disease: for example, acute events often precipitate by thrombosis developing on a ruptured arterial plaque.
Plasma viscosity is an important hemorheologic variable, and it is mainly determined by several macromolecules, such as fibrinogen, immunoglobulins, and lipoproteins [240].
Plasma viscosity is an indicator of blood flow in the network of small blood vessels that constitute microcirculation. An elevated plasma viscosity indicates increased resistance to blood flow in most tissues of the body [241].
Chronic hyperviscosity is able to impair microcirculation and promote target organ damage [242], and it is considered an independent predictor of cardiac events and mortality [243–245].
In a recent study, plasma viscosity is not connected to serum androgen levels, but it is correlated with serum fasting insulin and cholesterol levels, which appear to be higher in hirsute women compared to the matched for age and BMI healthy controls [246, 247].
Mild or chronic hyperviscosity is very frequent in older patients with metabolic syndrome (MS) and insulin resistance (IR) [248–250]. A deterioration of plasma viscosity was found to be present even in young, slightly overweight, PCOS women with IR who might be exposed to the same risk factors for cardiovascular diseases as older obese patients with MS. For this reason, plasma viscosity might be useful in the assessment of cardiovascular risk in young women with PCOS, in addition to plasma cholesterol and atherogenic index (triglycerides/HDL-C) [247].
Hyperinsulinemia contributes to the pro-thrombotic state by reducing fibrinolysis and raising the level of PAI-1 (plasminogen activator inhibitor-1). The increase of the latter in PCOS women seems to be independent of BMI: elevated levels, in fact, were observed in lean PCOS women too [137].
The procoagulant state is, in part, due to platelet hyperactivity, which was observed in lean women with PCOS [251] and in type II diabetic patients [252]. Why?
Platelets are involved in acute thrombosis, initiation of atheroma, and modulation of inflammatory responses, and they contribute to endothelial dysfunction [253]. Platelets are able to adhere to intact activated endothelium in the absence of exposed extracellular matrix proteins [254]. These adherent platelets could have a critical role in atherogenesis phenomenon, by secreting chemokines CCL5, CXCL4, and IL-1 [255].
Normally, platelet activation is counterbalanced by inhibitory signaling cascades that are activated by endothelial-derived NO and prostacyclin (PGI2), which modulate excessive activation [256].
Platelet hyperactivity seems to be related to acute hypertriglyceridemia: in fact, high levels of triglycerides might decrease the production of endothelial NO and PGI2, acting as a stimulator of platelet activation [257].
3.2.3.8 Chronic Inflammation, Endothelial Function, and Atherosclerosis
The presence of cardiovascular risk factors such as obesity, insulin resistance, and dyslipidemia may predispose PCOS women to coronary heart disease, but the topic is still controversial [137].
One of the early signs of cardiovascular lesions is the endothelial injury [258]. Several authors have reported precocious anatomical and functional arterial changes in PCOS women [259–261].
A positive correlation was demonstrated between abnormal endothelial function and testosterone levels in hyperandrogenic insulin-resistant women [262], while others have reported no differences for increased cardiovascular risk [263].
Mechanisms involved in the development of endothelial dysfunction could be the following:
Insulin stimulates both endothelin-1 and NO activity in the skeletal muscle circulation: an imbalance between the release of these factors may be involved in the pathophysiology of endothelial dysfunction.
In normal women, aging per se is associated with progressive attenuation of nitric oxide signaling; in PCOS women, these changes are present in early adult life, predisposing polycystic ovarian syndrome patients to premature atherosclerosis; in fact, high levels of plasma ADMA were found: endogenous NO synthase inhibitor NG-NG-dimethyl-L-arginine (ADMA) is a biochemical marker/mediator of endothelial dysfunction [267].
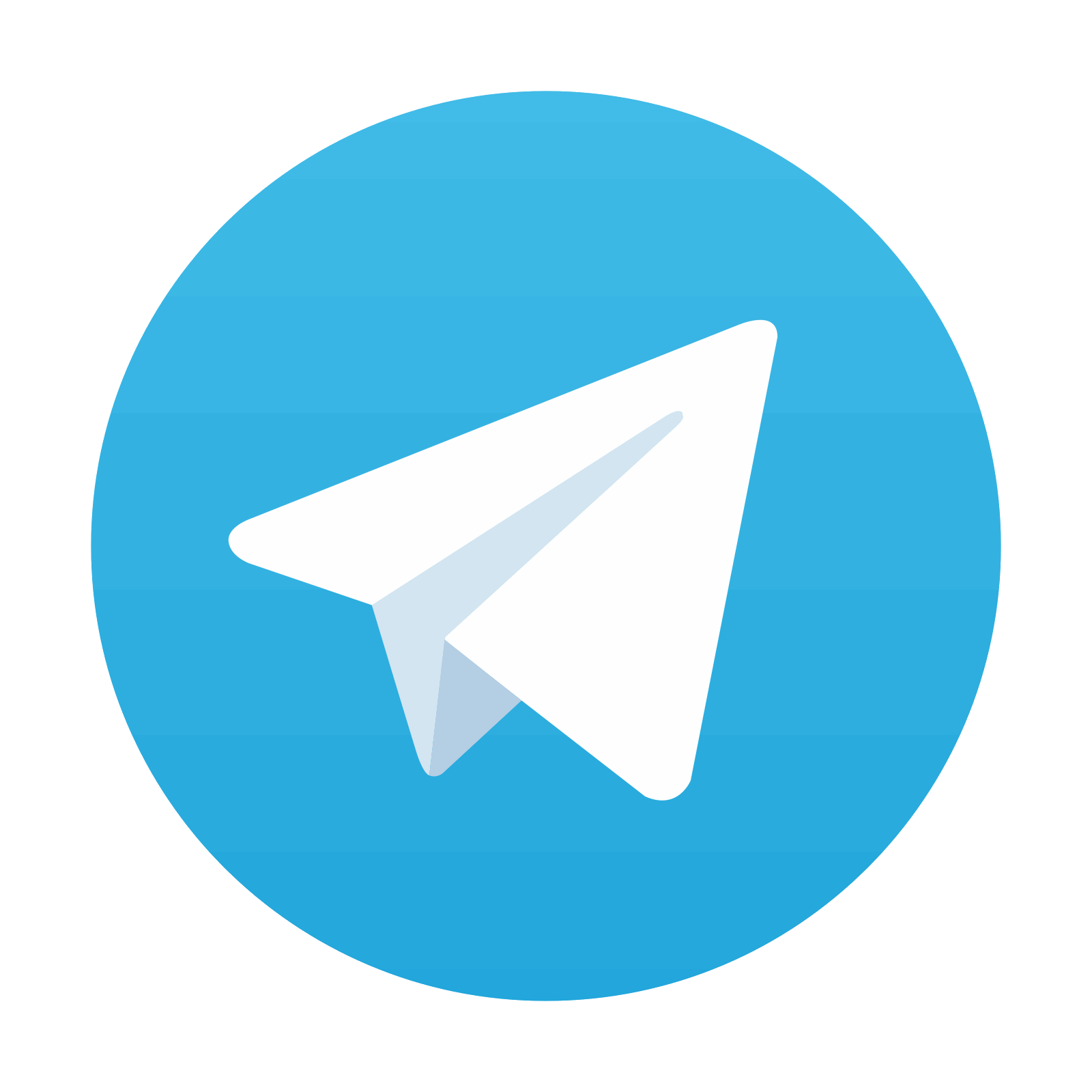
Stay updated, free articles. Join our Telegram channel

Full access? Get Clinical Tree
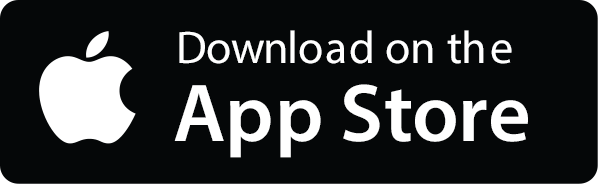
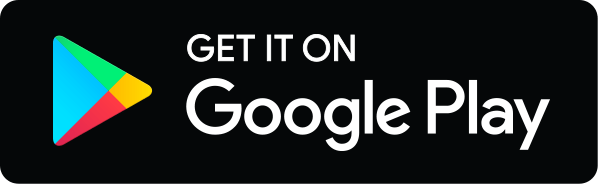