Patient positioning is extremely important in preparation for an LP. Children may lie in the lateral recumbent position with their hips and knees flexed, or they may sit upright with their hips flexed. While the interspinous space may be maximized in the sitting position (7), either position may be used according to clinician preference to maximize the success of the procedure. Use of local anesthetic may improve the likelihood of success in the absence of sedation (8). The needle should be introduced in the L3-4, L4-5, or L5-S1 space (6,9), with the bevel parallel to the longitudinal fibers of the dura.
Once CSF flow is obtained, an opening pressure (OP) should be measured on all patients with a standard manometer. For an accurate measurement, children should lie in the lateral recumbent position with legs either flexed or extended (10); it is not helpful to measure an OP if the patient is sitting upright. Pressures may be elevated in certain infections (such as Cryptococcus) and low in others (such as a spinal epidural abscess [EA] with spinal block). The commonly accepted normal range of CSF OP in adults is 10 to 25 cm H2O (11). Normal values in children are poorly established. A recent prospective study of 197 children between 1 and 18 years old suggests that a range of 11.5 to 28 is probably normal (12).
After measuring the OP, a minimum of 4 tubes of CSF should be collected: the first and last for cell count, and the others for chemistries and Gram stain/bacterial culture. Additional tubes may be sent for analysis according to the specific testing indicated for each case. It is imperative to obtain two tubes to measure cell counts so elevated red blood cell (RBC) count in a traumatic LP may be distinguished from an intracranial hemorrhage. Withdrawing the needle with the stylet in place may reduce the risk of CSF leak and headache later (13). Common but treatable transient side effects following LP include back pain in 40% (6,14) and spinal headache in up to 60% (3,6).
Interpretation of the LP varies by age (Table 16.2), but basic cell counts, chemistries, and OPs can help significantly narrow a differential diagnosis (Table 16.3). Several general rules of thumb in interpreting LP results are as follows:
-
Color: Normal CSF should be clear and colorless; turbidity may be due to high white blood cell (WBC) or RBC. CSF may be grossly bloody with just 6,000 RBC (15). Xanthochromia refers to a yellow/pink discoloration of the CSF, due to the presence of bilirubin (related to blood breakdown products), elevated protein, or systemic hyperbilirubinemia. The presence of xanthochromia with RBCs suggests blood has been present in the CSF for at least 2 hours. Xanthochromia due to hemorrhage may persist for 2 to 4 weeks (16).
-
WBCs: In general, infants have a higher normal CSF WBC than older children, with a higher percentage of polymorphonuclear cells (17,18). A WBC differential composed primarily of lymphocytes or monocytes is suggestive of, but not specific for, a viral process.
-
RBCs: For LPs with gross blood, the WBC count may be corrected by decreasing 1 for every 700 RBC (19), when RBCs are below 10,000. Above 10,000, this rule of thumb may not be reliable. Grossly hemorrhagic LPs may be seen with a traumatic LP or intracranial (subarachnoid or intraparenchymal) hemorrhage. Hemorrhagic LPs can cause falsely negative herpes simplex virus (HSV) polymerase chain reaction (PCR) or falsely positive venereal disease research laboratory (VDRL) test for syphilis or Epstein-Barr virus (EBV) PCR. In addition, breakdown of the blood brain barrier (BBB) with hemorrhage may result in organisms or antibodies causing a peripheral/non-CNS infection to be found in the CSF, or may introduce an infection into the CNS.
-
Protein: Normal protein concentration varies with age (Table 16.2), and may increase by 1 mg/dL per 1,000 RBC in older children and 2 mg/dL per 1,000 RBC in neonates in a hemorrhagic LP (20). Protein levels that are depressed are rarely pathologic, but proteins that are elevated may be due to anything that disrupts the BBB (21). For proteins over 100 to 200 mg/dL, spinal block should be considered and diagnostic spinal cord imaging should be performed. One may see elevated protein with a normal WBC (albuminocytologic dissociation) in acute inflammatory demyelinating polyneuropathies, such as Guillain-Barré syndrome (GBS).
-
Glucose: Normal concentration ranges for CSF glucose are based on serum glucose measured within 1 hour prior to LP. A ratio of 0.6 CSF to serum or greater is normal (22,23). Hypoglycorrhachia may be seen with bacterial meningitis, tuberculous meningitis, some fungal meningitides, neurosarcoidosis, or tumors (21), but CSF glucose concentrations less than 18 mg/dL are strongly suggestive of bacterial meningitis (24). Glucose concentration in lymphocytic pleocytosis can greatly help narrow a differential diagnosis (Table 16.3)
-
Specific organism testing: varies by pathogen. Please reference the Encephalitis/Myelitis and Immunocompromised Hosts sections of this chapter for specific recommendations.
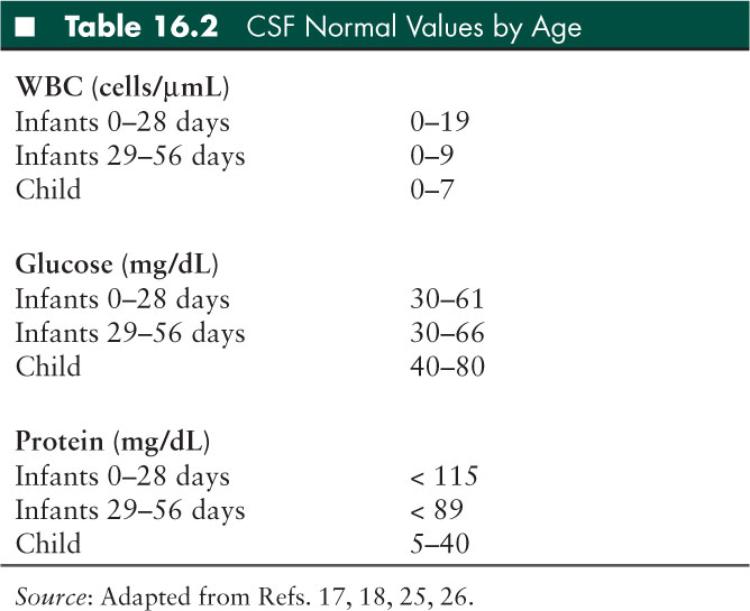
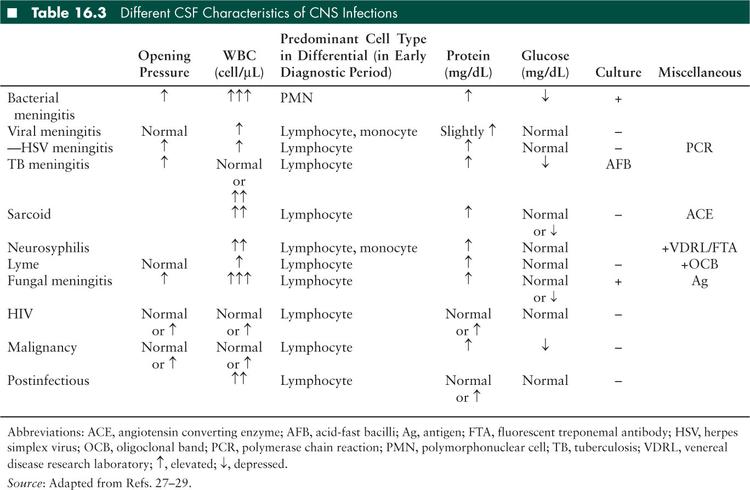
Neuroimaging
CNS imaging is typically obtained in the child with focal neurologic deficits, obtundation, or seizures. A variety of imaging modalities exist, each with different advantages and disadvantages. Neuroimaging for specific disorders will be discussed in the following sections.
Computed tomography (CT) is a fast and effective study to help exclude acute problems that require interventional measures such as hemorrhage, large mass, obstructive hydrocephalus, or large vascular territory ischemic stroke. CT of the head is frequently used prior to the decision to perform an LP when there is concern for possible elevated ICP. Spinal CT with contrast is superior to a plain x-ray for detection of a paraspinal infection, but is not sensitive enough to exclude the presence of an early discitis or epidural abscess (EA), and does not assess the integrity of the spinal cord itself. Disadvantages of performing a CT include radiation exposure, the possibility of an acute allergic reaction to the radiodense, iodinating contrast agents, and the risk for acute kidney injury in subjects with underlying renal disease.
Magnetic resonance imaging (MRI) is more sensitive than CT for small structural lesions and inflammation. Diffusion-weighted imaging (DWI) is particularly sensitive to ischemia, and may also be helpful distinguishing an abscess or empyema from a necrotic tumor. Susceptibility-weighted imaging can be used to identify very small areas of hemorrhage which may not otherwise be seen on CT. MRI of the brain is also more likely than CT to show meningeal enhancement in uncomplicated meningitis. MRI with DWI is superior to CT in identification of ischemia, cerebritis, abscess, empyema, and ventriculitis. MRI does not involve radiation. However, the lengthy image acquisition time necessitates sedation for many children. In addition, any ferromagnetic materials in the imaging suite are incompatible with the strong magnetic field of the MRI. Therefore, children with myringotomy tubes, braces, metallic pacemakers, orthopedic implants, or metallic neurostimulators may be unable to safely undergo an MRI exam. Finally, gadolinium enhancement may be contraindicated in patients with renal disease given the small risk of nephrogenic systemic fibrosis or nephrogenic fibrosing dermopathy.
■ MENINGITIS
Meningitis refers to inflammation of the tissues surrounding the brain and spinal cord, usually caused by an infection of the CSF. Presentations range from acute and imminently life-threatening with acute bacterial meningitis, to chronic and smoldering with recurrent types of meningitis.
Acute Bacterial Meningitis
Epidemiology and Pathophysiology
Acute bacterial meningitis is one of the top 10 causes of infection-related death worldwide (30), with an annual incidence of about 2,000 pediatric cases in the United States per year (31), and accounting for about 5% of all cases of meningitis in developed countries (32). Bacterial meningitis is a medical emergency. Mortality in treated cases ranges from 3% to 40% (27,31,33), and approaches 100% without appropriate treatment. Even following optimal treatment, neurologic sequelae including hydrocephalus, visual and hearing loss, developmental delays, and cognitive deficits are common among survivors, given the rapid progression of infection and subsequent parenchymal damage.
Bacterial meningitis occurs when invading pathogens overcome host defenses to reach the subarachnoid space. In neonates, pathogens are typically acquired from maternal genital secretions. In children who suffer head trauma leading to skull defects causing CNS leaks, or with congenital dural defects, the CNS may be directly inoculated with an environmental pathogen (27). In most infants and children though, the route of pathogen entry is through the nasopharynx. In individuals with immature humoral immunity, or in the context of organisms with a particularly virulent capsule, colonizing bacteria may invade the nasopharyngeal epithelium (34). During subsequent bacteremia, a robust bacterial capsule again resists normal host defenses of complement mediated bactericidal activity, neutrophil phagocytosis, and circulating antibodies (35), allowing the organism to invade the CNS via one of several routes of entry: through the choroid plexus epithelium, through the dural venous sinus system, or through the cribriform plate. The CNS physiologically stocks lower concentrations of immunoglobulins and fewer complement factors than the systemic circulation, so once a pathogen reaches the subarachnoid space, bacterial seeding of the meninges proceeds relatively unhindered. Bacterial replication and autolysis then induces the release of proinflammatory cytokines, chemokines, and cellular toxins, causing inflammation and leukocyte recruitment into the subarachnoid space. This inflammatory response results in increased BBB permeability, increased CSF outflow resistance, and loss of autoregulation with changes in cerebral blood flow. Vasogenic edema, hydrocephalus with interstitial edema, and cytotoxic edema may ensue, all of which may culminate in increased ICP. Ventriculitis may also lead to pial vein, cortical arteriole, and venous sinus thrombosis, and subdural effusions (34).
Causative organisms vary depending on the route of pathogen entry and host factors affecting the pathophysiology of infection. Before 2000, Haemophilus influenzae type b (Hib) was the major cause of bacterial meningitis in children. However, following the introduction of a Hib vaccine to the infant vaccine schedule in the early 1990s, the overall incidence of bacterial meningitis in children less than 5 years of age was significantly reduced. The introduction of the Streptococcus pneumoniae (pneumococcus) conjugate vaccine to the infant vaccine schedule in 2000 reduced the incidence of pneumococcal meningitis in children in the United States by 55% to 60%. However, S pneumoniae remains the most common cause of bacterial meningitis in children (31) because the standard pneumococcal vaccine includes only 13 of more than 90 serotypes. Host factors that may predispose a child to developing bacterial meningitis include lack of opsonizing antibodies, surgical or functional asplenia, complement deficiency, glucocorticoid excess, HIV infection, bacteremia, basilar skull fracture, or other traumatic or inflammatory breach of the BBB. Individuals with CSF shunts are particularly prone to staphylococcal infections with both coagulase-negative staphylococcal species and Staphylococcus aureus, in which the infection begins as ventriculitis but may spread to the meninges. Children in the early postneurosurgical period are particularly prone to S aureus meningitis, although this is rare. Finally, a child’s age predisposes them to various infections, based on their environmental exposures and the maturation of their immune system (Tables 16.4 and 16.5).
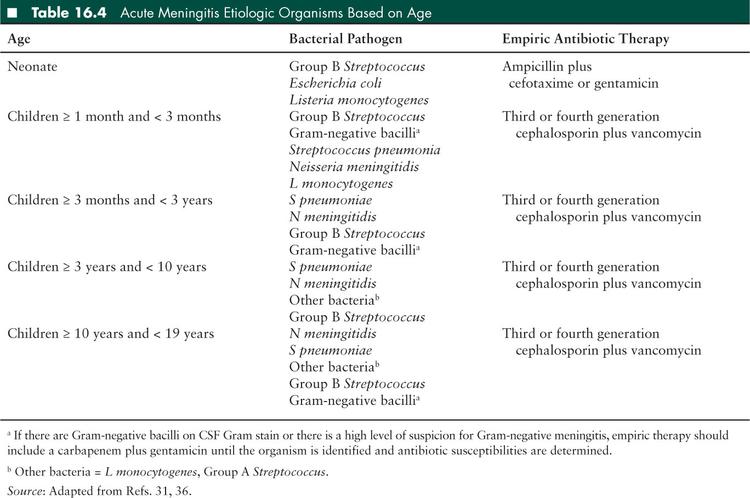
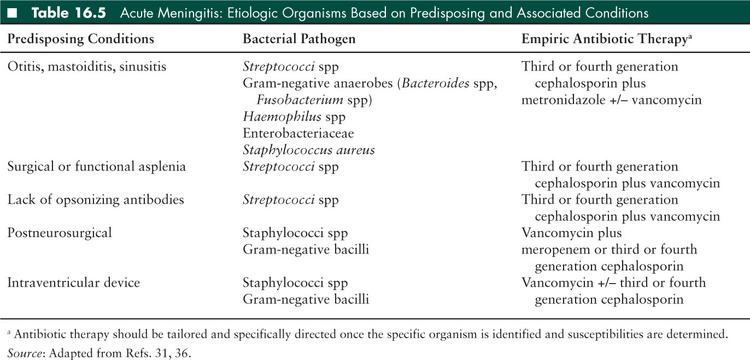
Clinical Presentation
Acute bacterial meningitis may present progressively over several days, or fulminantly over several hours, in which case it is more likely to be associated with significant brain edema. Signs and symptoms of meningitis may be preceded by an upper respiratory infection or otitis media. Most children initially present with fever and signs of meningeal inflammation (meningismus), including altered mental status (irritability or lethargy), headache, nuchal rigidity, emesis, and/or photophobia (36); however not all children have a classic presentation. For example, 6% to 20% of children may be afebrile (37,38) and up to 16% may not have meningeal signs. Nuchal rigidity is observed in less than 50% of children with meningitis (27,36) and may be absent in early infection (38). Nuchal rigidity is commonly assessed using Kernig and Brudzinski signs. A Kernig sign is present if the supine patient, with hips and knees flexed at 90°, has significant pain with extension of the knee less than 135°. A Brudzinski sign is present if the supine patient flexes the lower extremities during attempted passive flexion of the neck. Infants typically have a more nondescript presentation of bacterial meningitis, involving fever or hypothermia, altered mental status (irritability, restlessness, lethargy), poor feeding, respiratory distress, emesis or diarrhea, and/or a bulging fontanel. In all children, petechiae and purpura may be a sign of Neisseria meningitidis (meningococcal) infection. The current immunization for meningococcus does not include serogroup B, which predominantly affects younger children and is responsible for one-third of the cases of meningococcal meningitis in the United States (27).
Neurologic findings in acute bacterial meningitis are variable. Altered level of consciousness, manifested primarily by irritability or lethargy is common, and children who are less responsive have a poorer prognosis. Increased ICP may result in a headache, bulging fontanel, or palsies of the third, fourth, or sixth cranial nerve (sixth nerve palsy is most common). Papilledema typically takes several days to develop, making it less helpful in the acute diagnostic setting. Up to 16% of patients, and 34% of those with pneumococcal meningitis, may have focal neurologic findings related to parenchymal extension of their meningitis (meningoencephalitis), increased ICP, or stroke, commonly related to an inflammatory vasculitis. Focal neurologic signs also correlate with increased morbidity and mortality. Finally, 20% to 30% of children with bacterial meningitis may have seizures, typically at the time of presentation. While most seizures are generalized and within the first 48 hours of admission, focal seizures may occur later in the course, and typically indicate a focal parenchymal injury. It is extremely unusual for bacterial meningitis to present with fever and a single brief generalized seizure alone. A study of 506 children aged 2 months to 15 years at two hospitals over a 20-year period found no cases of acute bacterial meningitis presenting solely with a simple febrile seizure (39). Another recent study examined 704 children aged 6 to 18 months presenting to a large tertiary care emergency department with a first time simple febrile seizure: none of these children had bacterial meningitis (40).
Diagnosis
As with all neurologic infections, a thorough history should be taken in all children with suspected bacterial meningitis, with particular attention to the course of illness, presence of predisposing immunologic factors, concurrent infections or head trauma, recent travel to areas with endemic meningococcal disease, and exposure to an individual with bacterial meningitis. Special attention should be given to immunization history. Complete physical and neurologic examinations should be performed. The constellation of systemic hypertension, bradycardia, and respiratory depression (Cushing triad) is a late sign of increased ICP. Children with bacterial meningitis may have concomitant bacterial infections at other sites (e.g., arthritis with N meningitidis, pneumonia or endocarditis with S pneumoniae).
Initial blood tests should include a complete blood count with differential, serum electrolytes, blood urea nitrogen, creatinine, glucose, and aerobic blood cultures. Blood cultures are positive in up to 80% of patients with previously untreated bacterial meningitis (41). Coagulation studies should be included if petechial or purpuric lesions are present. An LP should be performed in any child with suspected bacterial meningitis, unless specific contraindications are present (see above). Evaluation should include cell counts from two tubes, glucose, protein, Gram stain, and culture. Cell counts should be examined within 90 minutes of the LP to avoid WBC disintegration (41). Although several theoretical methods exist to differentiate between a traumatic LP versus a hemorrhagic LP, none have been validated (42), and clinicians should err on the side of treating conservatively while awaiting confirmatory results. In bacterial meningitis, CSF-to-serum glucose ratios are typically decreased to ≤ 0.6 in neonates, and ≤ 0.4 in children over 2 months of age (27). Specific organism testing may be performed as indicated (see Encephalitis and Myelitis section of this chapter, Table 16.21). Early in the course of disease, a culture may be positive in the absence of a pleocytosis (43), and about 10% of cases with bacterial infection will have an initial lymphocytic predominance (44), making clinical differential diagnosis slightly more challenging. However, a high OP, pleocytosis with neutrophil predominance, elevated protein, and depressed glucose are more commonly observed (Table 16.3). Bacterial culture results in the definitive diagnosis, however growth from culture typically takes at least 24 hours (45). The sensitivity of CSF Gram stain exceeds 70%. Despite a high specificity of 80% to 92%, the positive predictive value of a Gram stain without culture is low (approximately 50%) given the very low prevalence of bacterial meningitis in the population at large (45–47). Reasons for falsely positive CSF Gram stains include observer misinterpretation, reagent contamination, and use of an occluded spinal needle, the latter of which leads to skin contamination. If CSF parameters are consistent with bacterial meningitis but culture does not yield bacterial growth, an empiric course of antibiotics should be considered.
Neuroimaging is typically normal in the early stages of bacterial meningitis, and is not necessary in uncomplicated disease. Neuroimaging in bacterial meningitis is appropriate when focal neurologic signs are present (raising concern for the presence of other pathologic conditions), if there is concern for a parameningeal infection focus (which is rare, and mostly seen in partially treated disease) or other anatomic condition predisposing a patient to the infection, or to look for obvious radiographic signs of elevated ICP prior to LP. Within several days of symptom onset, leptomeningeal enhancement and widened basal cisterns may be observed on CT. Occasionally, subdural effusions may be present later in the disease process, and less commonly, diffuse cerebral edema or abscess formation. Head ultrasound may be used for the examination of infants.
Complications
Acute and late neurologic complications of bacterial meningitis occur in 16% to 50% of survivors (48,49), and are related to the age and baseline health of the patient, causative organism, and the severity and duration of illness.
Early neurologic complications in children are varied. Increased ICP may occur within the first 48 hours in up to 50% of children who require intensive care monitoring (44). This elevation is typically associated with Cushing triad and progressive obtundation. Cranial nerve palsies (particularly third, fourth, sixth, and eighth) may occur secondary to elevated ICP, or as primary complications. Seizures occur in about 30% of children with bacterial meningitis early in the course of illness and often before presentation to medical care (2). In some cases seizures may be due to parenchymal spread of infection, in others they are thought to be due to cortical irritation resulting from associated inflammation, fever, and electrolyte changes. The majority of seizures resolve without directed intervention over several days. However, seizures that are prolonged or difficult to control may be associated with later neurologic sequelae (43,50). Subdural effusions occur in up to 50% of Hib meningitis, and in up to 30% with other infectious etiologies (27). They are typically sterile and self-resolve, however, if a child has protracted or focal symptoms, or partially treated meningitis with a prolonged course, subdural effusions may become superinfected and subdural drainage should be considered. Ventriculitis occurs most commonly in infants and presents with persistent fever. This complication requires prolonged antibiotics. Cerebritis and infarction result from direct spread of infection to the parenchyma of the brain, or due to inflammatory changes of the blood vessels related to the inflammatory cytokine milieu. Both are associated with abscess formation and poor prognosis.
Transient or permanent sensorineural deafness can result from inflammatory or bacterial damage to the eighth cranial nerve, cochlea, or labyrinth, or from the use of ototoxic antibiotics. Permanent hearing loss may occur in up to 11% of cases, and is more likely in children with a longer duration of symptoms, lack of petechiae (in the case of meningococcal disease), a low CSF glucose, and ataxia. In addition, it is significantly more common in Hib and pneumococcal infections than in meningococcal infections (51). All patients with bacterial meningitis need a hearing test prior to hospital discharge, and should be followed closely as outpatients.
Later neurologic complications include cognitive and behavioral deficits, seizures, and motor deficits. Less common complications of bacterial meningitis include transverse myelitis, infarction, permanent hydrocephalus, aneurysm formation of intracranial vessels, and cortical visual impairment.
Treatment
Treatment of bacterial meningitis is meant to inhibit bacterial replication, prevent cerebral edema, and prevent the secondary effects of proinflammatory cytokines in the subarachnoid space. Suspected meningitis should be treated immediately with empiric intravenous antibiotics; treatment must not be delayed if there is a contraindication to or a delay in ability to perform LP (36). In such situations, blood cultures should be obtained, and empiric antibiotics administered as soon as possible. Antibiotics typically sterilize a CSF culture and Gram stain within 2 to 48 hours, depending on the organism (52); earlier sterilization appears to occur with N meningitidis compared with other pathogens. CSF glucose begins to normalize over 12 hours following treatment (53). However, the CSF WBC and protein elevations frequently persist for up to 7 days (44). Once organism identification is made, and antibiotic susceptibilities are available, antibiotic selection can be appropriately directed. A repeat LP for test-of-cure in neonates with Gram-negative meningitis is recommended and may alter the duration of therapy, but is not routine for other clinical scenarios in which a child is recovering within the expected time course (2).
Choice of empiric antibiotics varies with age, and care must be taken to use antibiotics with appropriate CNS penetration. However in general, coverage should include a third generation cephalosporin, in addition to further coverage based on specific risk factors (Tables 16.4 and 16.5). In most cases of bacterial meningitis, a 10- to 14-day course of treatment is necessary, although some require longer durations of treatment. General recommendations for organism-specific antibiotics and duration of treatment are listed in Table 16.6, but individual treatment should always be discussed with an infectious disease specialist. Acyclovir should be added to an empiric regimen until HSV meningitis is definitively excluded.
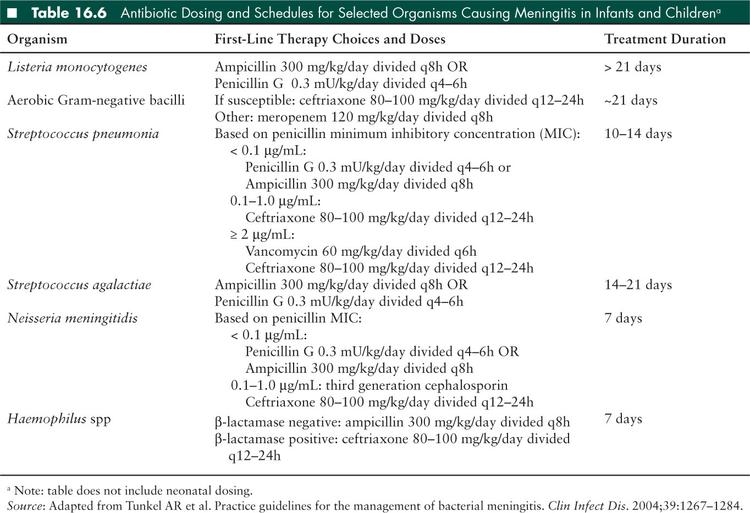
Use of adjunctive corticosteroid therapy has been closely examined in bacterial meningitis with both prospective and retrospective randomized trials and meta-analyses in infants and children. When antibiotics are administered, the resultant bacterial lytic products, including endotoxin, increase the host inflammatory response in the subarachnoid space, resulting in further cellular damage. In general, glucocorticoids are thought to suppress proinflammatory cytokines in the subarachnoid space, and therefore decrease eighth cranial nerve damage and subsequent hearing loss, the clinical duration of fever, parenchymal damage and later neurologic deficits, and perhaps mortality (54). In animal studies, it has also reduced cerebral edema, elevated ICP, and CSF outflow obstruction (27). However, it does not appear to reverse existing CNS damage.
The only proven indication for adjunctive glucocorticoid treatment of meningitis is in the setting of Hib infection. In this case, dexamethasone 0.4 mg/kg IV every 12 hours for 2 days is recommended. It is most beneficial when administered with, or just before parenteral antibiotics (33). The American Academy of Pediatrics recommends using dexamethasone only if it can be given within 1 hour of the first dose of antimicrobial therapy. Use in pneumococcal infections or as empiric therapy is more controversial. Several recent studies suggest no benefit to children for mortality (55) or hearing loss (56). The role for dexamethasone in the treatment of neonates is less clear (27).
Acute complications of meningitis require intensive care. Subdural empyemas (SDEs), intraparenchymal abscesses, and communicating hydrocephalus must be addressed surgically. The syndrome of inappropriate antidiuretic hormone (SIADH) may be treated with fluid restriction if the patient is hemodynamically stable; this frequently self-resolves with meningitis recovery. Patients who are stuporous or comatose may require ICP monitoring. Treatment for increased ICP include head of the bed elevation to 30°, hyperventilation to maintain PCO2 at 25 to 30 cm H2O, use of hyperosmolar agents such as hypertonic saline or mannitol, or neurosurgical decompression. Seizures are managed routinely, depending on frequency and type.
Chemoprophylaxis is recommended for close contacts or in outbreak situations for meningococcal disease and Hib. High-risk contacts include household members, incompletely immunized or immunocompromised contacts, and, in the case of meningococcal disease, those who had direct exposure to the index patient’s respiratory secretions at any time within 7 days prior to onset of illness. Contact of patients with Hib meningitis should receive rifampin, and contacts of patients with meningococcal meningitis should receive rifampin, ceftriaxone, ciprofloxacin, or azithromycin. Chemoprophylaxis should be addressed for all contacts immediately following diagnosis.
Acute Viral Meningitis
Viral meningitis and encephalitis have similar epidemiologies, causative organisms, pathophysiologies, and treatments. For a complete review, please see Encephalitis and Myelitis section of this chapter. Briefly, the most common causes of viral meningitis are enteroviruses (EVs), followed by parechoviruses, herpesviruses, arboviruses, and influenza. The clinical presentation of viral meningitis is similar to acute bacterial meningitis, although symptoms are typically less severe and more indolent. However, anyone presenting with acute meningitis should be treated empirically as acute bacterial meningitis with immediate antibiotics until an alternate diagnosis becomes clear (32). LP is the diagnostic procedure of choice. Viral meningitides classically demonstrate a mildly elevated WBC, elevated protein, and normal glucose (Table 16.3). The pleocytosis is typically lymphocytic, although may be neutrophil predominant in the early stages of infection. In general, viral meningitis is much more common than acute bacterial meningitis; 95% of all children with pleocytosis are ultimately diagnosed with viral meningitis (47).
Subacute and Chronic Meningitis
Epidemiology and Pathophysiology
Subacute and chronic meningitis account for about 10% of all cases of meningitis (57), with an increased prevalence in the immunocompromised population (58). The pathophysiology is similar to acute bacterial meningitis, however subacute meningitis evolves over days to weeks, and chronic meningitis persists for over 4 weeks (2,58,59). Clinically, the distinction between subacute and chronic meningitis is not important because both conditions are similar in presentation and etiology. In addition, patients rarely present after 4 weeks of meningeal symptoms, making the distinction unhelpful at the bedside. Therefore, subacute and chronic meningitis will be discussed here together. In broad terms, etiologies can be broken down into infectious versus noninfectious causes, however no etiology is found in 15% to 25% of cases (60). Noninfectious causes include autoimmune inflammatory disorders (Behçet, systemic lupus erythematosis, Wegener granulomatosis, or Vogt-Koyanagi-Harada syndrome), collagen vascular diseases, leptomeningeal neoplastic metastases, chronic subarachnoid bleeding, and chemical meningitides.
Infectious causes of subacute and chronic meningitis are diverse. Tuberculous (TB) meningitis (see Tuberculosis and Other Granulomatous CNS Infections section) and fungal meningitides (particularly cryptococcal disease; see Immunocompromised Hosts section) are the most common infectious causes. Other infectious etiologies include neurosyphilis (see Tuberculosis and Other Granulomatous CNS Infections section), neuroborreliosis, neurobrucellosis, rickettsial infections, Listeria monocytogenes, HIV (see Encephalitis and Myelitis and Immunocompromised Hosts sections), and parasitic diseases (see Protozoal and Helminthic infection section). Chronic viral meningitides outside of immunocompromised patients are rare.
Clinical Presentation
Most chronic meningitides present similarly, though more subacutely, to acute bacterial meningitis. Meningeal signs including headache and neck stiffness, lethargy, confusion, weight loss, and intermittent emesis predominate (58). If present, low-grade fevers are usually below 39°C (61). Less commonly, patients describe focal neurologic deficits, cranial nerve palsies, seizures, and radicular pain or paresthesias (58). Specific presentations of several etiologic organisms are as follows:
-
Borrelia burgdorferi meningitis typically presents approximately 4 weeks after the erythema migrans rash of Lyme disease appears (57), and may be associated with persistent erythema migrans and/or arthritis. The course is subtle and fluctuant (61) and may be accompanied by a facial palsy or painful neuritis (58), most commonly optic neuritis.
-
Systemic brucellosis presents with undulant fever, lymphadenopathy, and hepatosplenomegaly (61) following exposure to unpasteurized milk products or farm animals (60). Neurologic brucellosis occurs in less than 5% of cases of systemic disease (57). In the early septicemic phase an isolated leptomeningitis may occur, however patients may also develop a meningoencephalitis 2 months to 2 years following the initial episode (60), which may include cranial nerve palsies (61), radiculopathy, and peripheral neuropathy (57).
-
Rickettsial chronic meningitis (particularly Ehrlichia chaffeensis, Anaplasma phagocytophilum, and Babesia spp) occurs in about 10% of rickettsial infections, and is frequently not identified due to low diagnostic baseline suspicion and lack of specific testing.
-
Listeria causes basilar meningitis mimicking tubercular meningitis.
-
Other etiologic considerations for clinical presentation of chronic meningitis include syphilis and neurocysticercosis (see Tuberculosis and Other Granulomatous CNS Infections section, and Protozoal and Helminthic infection section).
Diagnosis
Because several of the causes of chronic meninigitis are zoonoses, thorough travel and animal exposure histories are important in diagnosis, as is a thorough physical exam to investigate noninfectious etiologies. LP is the diagnostic test of choice for chronic meningitis, following a good examination and history. However, isolation of a causative organism from the CSF is often difficult because the pathogen load in chronic meningitis tends to be much lower than in acute bacterial meningitis (58). In addition, many pathologic organisms are fastidious and difficult to isolate in standard culture (such as B burgdorferi, Rickettsia, and Ehrlichia), or quickly degenerate without proper CSF handling (such as Naegleria fowleri). Blood and CSF serologies, PCR, pathogen-specific antigen tests, and other serum markers of systemic inflammation may be helpful (see Encephalitis and Myelitis section Table 16.21).
Most cases of chronic meningitis have nonspecific CSF findings. Typically, there is a moderate lymphocytic pleocytosis (< 500 WBC/mL), mildly elevated protein, and normal glucose (57). Persistent neutrophilic meningitides are rare, but can be associated with fungal infections, TB, or brucellosis. Noninfectious cases of chronic neutrophilic meningitis may be due to neoplasm, systemic lupus erythematosus, or chemical meningitis (58).
Neuroimaging may be helpful in determining an etiology of chronic meningitis; MRI with gadolinium enhancement is the best modality to do so. Table 16.7 reviews MRI findings suggestive of specific pathogens and processes. Vascular imaging by conventional angiography or magnetic resonance (MR) angiography may be helpful to look for evidence of vasculitis or aneurysm; however, vascular imaging is not 100% sensitive. Meningeal biopsy may be considered for focal areas of meningeal enhancement if the etiology is unclear; meningeal biopsy has the highest yield if underlying brain parenchyma is examined in tandem (58).
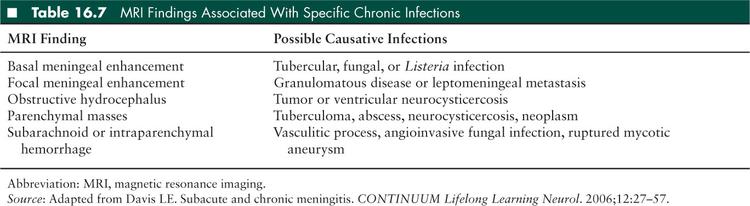
Treatment
Treatment for chronic meningitis is dependent on the etiologic agent (please see Acute Bacterial Meningitis and Tuberculosis and Other Granulomatous CNS Infections sections for organism specific treatment); however, as discussed above, a causative organism is frequently not found.
Recurrent Meningitis
Recurrent meningitis is defined by repeated episodes of appropriately treated acute meningitis, followed by periods of absent meningeal signs and normal CSF parameters (57). Recurrent meningitis may be bacterial, fungal, or aseptic.
Recurrent bacterial meningitis in children occurs with 1.3% to 5% of community acquired bacterial meningitis episodes (62). Most cases of recurrent bacterial meningitis are due to traumatic or congenital skull-based anatomic defects, parameningeal infectious foci, or immunodeficiencies (59). Following skull fracture, recurrent cases of S pneumoniae, Hib, and meningococcal meningitis have been described. Parameningeal foci include brain abscess, chronic osteomyelitis of a skull bone, or mastoiditis. Acute diagnostic testing and treatment are similar for recurrent bacterial meningitis as for chronic meningitis. Neuroimaging should be used to look for an anatomic source. If possible, anatomic defects should be repaired (63), and immune reconstitution should be implemented. Prophylactic antibiotic use in cases of known skull base defects is controversial, and not routinely recommended (62).
Recurrent aseptic meningitis may be caused by waxing and waning chronic meningitis (particularly Cryptococcus), although in these cases the CSF is persistently abnormal between acute episodes. Mollaret meningitis is a specific syndrome of benign recurrent aseptic meningitis characterized by sudden short bouts of fever, meningismus, and CSF profiles suggestive of viral infection. Mollaret meningitis primarily affects young adults, but has been reported in children as young as 5 years old (63). Typical episodes last 1 to 5 days, occurring at weekly, monthly, or yearly intervals, and often persist over many years. While some cases of Mollaret meningitis have been associated with HSV-2 CNS infection (57,63), many cases remain idiopathic. Patients with known HSV-2 CNS infection should be treated with antiviral therapy, although no controlled trials have formally evaluated this recommendation. The typical course of Mollaret meningitis is benign (63).
Latent infections such as HSV, EBV, and toxoplasmosis (59), chronic inflammatory diseases, and chemical meningitides may also cause a recurrent aseptic meningitis. Recurrent chemical meningitides may be due to intermittent discharge from epidermoid cysts or craniopharyngiomas, and neuroimaging may again be helpful in identifying these structural etiologies. Drug-induced recurrent aseptic meningitis is rare, but has been reported with nonsteroidal antiinflammatory drugs (NSAIDs), sulfa based antibiotics, and intravenous immunoglobulin (IVIg) (63). Vascular inflammatory disorders that are typically classified as chronic meningitis (such as Behçet) may also present with more intermittent symptoms and be clinically classified as recurrent cases.
Noninfectious Causes of Meningitis
There are multiple noninfectious causes for meningitis (Table 16.8).
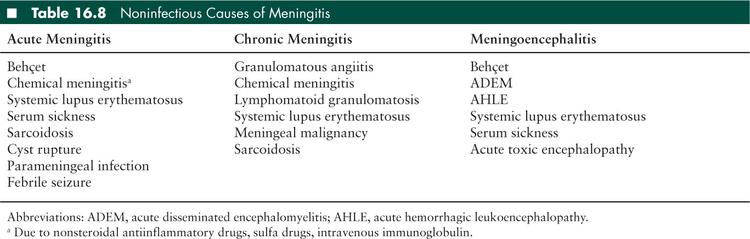
■ FOCAL SUPPURATIVE INFECTIONS
Brain Abscess
One thousand five hundred to 2,500 brain abscesses are diagnosed annually in the United States, and the incidence appears to be decreasing (64). While previously uniformly fatal, the advent of new neurosurgical techniques, antimicrobial therapy, and CT scanning (65) has improved morbidity and mortality with early and aggressive diagnostic and therapeutic strategies. However, brain abscesses continue to be a serious and difficult to treat suppurative intracranial infection, with a mortality rate in children of 4% to 12% (66).
Epidemiology and Pathophysiology
Brain abscess is defined as a focal intracerebral collection of pus surrounded by a well-vascularized capsule (67). Abscesses develop over the course of 2 weeks or more, beginning with a cerebritis stage, which is an ill-defined inflammatory infiltrate. The lesion progresses to become more organized and ultimately an encapsulated collection of necrotic tissue surrounded by a thick-walled capsule. A brain abscess can be staged based on clinical, histologic, and radiographic findings (Table 16.9).
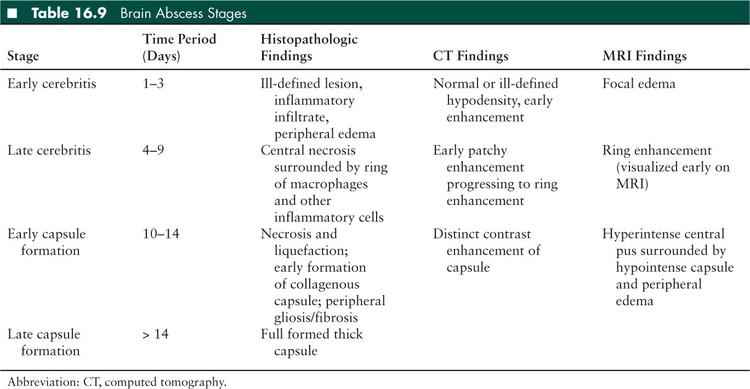
In general, the brain is well protected from microbial invasion by an impermeable BBB. The BBB is composed of CNS endothelial cells joined by tight junctions (67) surrounded by a basal lamina. Astrocyte foot processes then ensheath these vessels on the parenchymal side. Interactions between these cells and immune cells regulate the permeability of the BBB, therefore modulating the inflammatory and microorganism milieu allowed entrance to the brain parenchyma. States of inflammation such as meningitis or disrupted blood flow following embolic events increase the permeability of the BBB. Physical disruptions, such as penetration by a foreign body in the case of surgery or trauma also upset this baseline homeostasis.
Bacteria reach the brain parenchyma via several different routes including direct extension from a cranial source of infection, hematogenous dissemination from a nearby or distant organ infection, or exogenous introduction. Direct extension is most common, typically from a paranasal sinusitis or otitis media; these sources may also reach the brain parenchyma via a valveless venous drainage system in the crania mucosa that communicates with the venous plexus of the dura mater (66,68) (Figure 16.1). Dental infections may also extend into parenchymal space (Tables 16.10 and 16.11).
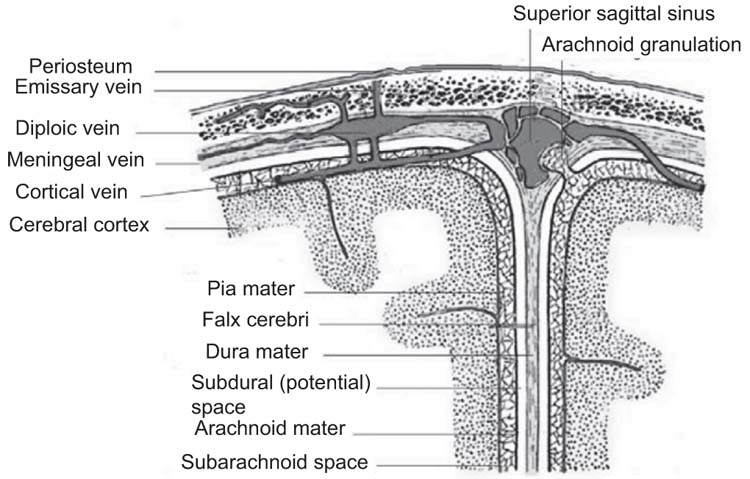
FIGURE 16.1 Anatomy of the meninges and vascular anatomy of sinuses. Adapted from Gray’s Anatomy of the Human Body, 1918 (public domain).
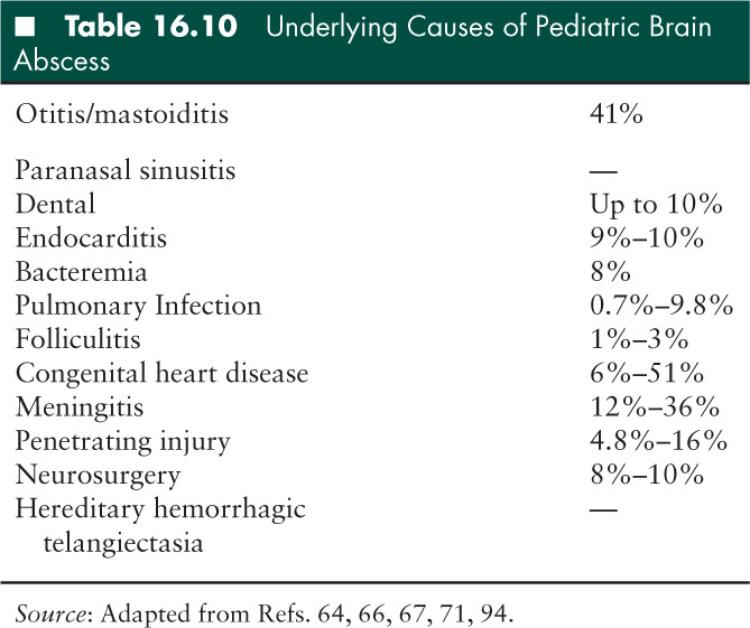
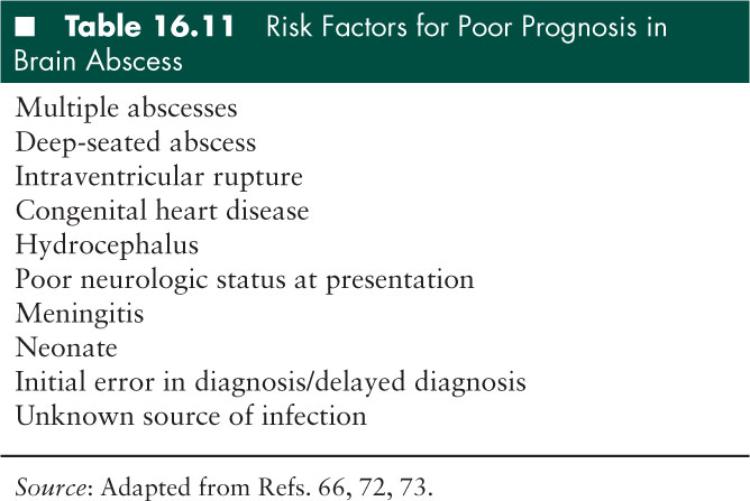
Several known predisposing factors lead to brain abscesses in children, although 15% to 30% of cases remain cryptogenic (66) (Table 16.11). Some organisms implicated in meningitis, specifically enteric Gram-negative bacilli, are more likely to cause abscesses (64), and infants and toddlers are most susceptible to parenchymal abscess formation following bacterial meningitis. Congenital heart disease, particularly with pronounced right-to-left shunting, is a risk factor for brain abscess: a patent foramen ovale or other atrioventricular septal defect may contribute to brain abscesses of unclear etiology (67). Intracranial trauma and surgery are risk factors for brain abscess with direct introduction of organisms to the sterile intracranial space. Trauma in which a foreign body or bone fragment is found in the brain parenchyma, or surgery which incorporates a foreign material into the intracranial space such as a ventriculoperitoneal shunt provide a nidus for infection. Children more frequently sustain penetrating orbital or otic trauma from sharp objects such as pencils, sticks, or lawn darts (66,69,70). Intracranial hemorrhage, ischemia, and tumors are additional risk factors for parenchymal abscess (67). These conditions affect certain pediatric populations, but occur more frequently in adults.
Epidemiologically, there is a male predominance among patients with brain abscesses and approximately 25% of brain abscesses occur in children (64,71). Brain abscesses are most common in children 4 to 7 years of age (66). Immunosuppressed patients and those with congenital heart disease are at a higher risk of developing brain abscess for the reasons discussed above.
Clinical Presentation
Headache is the most common reported presenting symptom of brain abscesses, occurring in up to 56% of patients (72), and is more often chronic and poorly characterized than acute (64,67). A sudden worsening of headache may be indicative of meningeal irritation due to rupture of abscess into the ventricular space. Fever occurs in approximately half of all cases. Papilledema is reported in 41% to 70% (66). Seizures, vomiting, nuchal rigidity, focal neurologic deficits, and altered mental status are less common.
Prognosis is worse in patients who present with significantly altered mental status (65–67,72) and in those in whom abscess ruptures into the intraventricular space, in which case mortality is >80% (67). In pediatric populations, the rate of pervasive neurologic sequelae is approximately 15% with modern diagnostic and therapeutic techniques (73).
Diagnosis
Early descriptions of brain abscesses report diagnoses made based on physical exam; however, prior to the late 19th century, brain abscess was 100% fatal and most diagnoses were made on postmortem examination. Perhaps the most influential factor in decreasing morbidity and mortality associated with brain abscess is the development of CT imaging in 1974. CT scanning allows for early diagnosis, localization for surgical procedures, and follow-up evaluation. Contrast-enhanced head CT is hypodense in the early cerebritis stage, reflecting focal edema. As the abscess organizes and encapsulates, CT will demonstrate a well-formed ring-enhancing lesion (64,67). Since the advent of CT neuroimaging, the rate of multifocal abscesses is recognized to be approximately 50%, which is significantly higher than previously suspected (64).
MRI with gadolinium is the preferred diagnostic study because it is more sensitive for detecting abscesses in the early cerebritis stage, with subtle edema demonstrated by T2 hyperintensity (66). Well-formed abscesses on MRI appear as T1 hypointense, T2 hyperintense, ring-enhancing lesions with surrounding edema. The necrotic core frequently diffusion restricts on DWI, as do some tumors and ischemic insults. MR spectroscopy can be helpful in the differential diagnosis of abscess and tumor based on different spectral profiles. Despite the advantages of modern brain imaging, brain abscess may be hard to distinguish from brain tumor. A surgical procedure is frequently necessary to make the definitive diagnosis.
LP is rarely helpful and potentially dangerous in patients with brain abscesses. If significant mass effect results from the abscess, there is a high risk of brainstem herniation during LP. The procedure is contraindicated in these cases. CSF examination is usually nonspecific, with pleocytosis and elevated protein, although may be normal and cultures are usually negative (67,72). Blood culture is often negative, but when positive, it may be helpful in identifying an organism.
Treatment
Treatment of brain abscess requires a combination of neurosurgical drainage, targeted antimicrobial therapy, and neurologic and intensive care supportive management. Initial stabilization measures are focused on decreasing ICP and controlling seizures. Seizures occur in 25% to 50% of brain abscesses (67) and are treated based on frequency and type. In cases of severe brain edema, mannitol and forced hyperventilation may be adjuncts to surgical care. Surgical stabilization, including decompressive craniotomy, is necessary for those patients with malignant ICP. An external ventricular drainage device may be warranted, especially in cases of intraventricular rupture of abscess (67).
Small lesions less than 2 centimeters may be managed successfully with only parenteral antibiotic therapy (64,67). Conservative therapy may also be appropriate for immature abscesses in the cerebritis stage or when multiple abscesses are present (68,72). However, early and aggressive surgical drainage should be performed for larger accessible lesions in appropriate patients. Several neurosurgical options exist: stereotactic biopsies may be done for deeper lesions, or complete excisions by craniotomy for multiloculated abscesses, those with resistant organisms, or lesions which have recurred despite prior drainage. Neither surgical option is associated with a more optimal outcome (66,72). Surgical management of paranasal sinusitis, otitis media, or other origin of infection must be undertaken in conjunction with abscess drainage.
Empiric antibiotic therapy is chosen based on the suspected origin of the infection. Brain abscesses are frequently polymicrobial due to the colonizing flora of the infection sources. The most common organisms are viridans group streptococci, Streptococcus milleri, and anaerobic bacteria (67,72). Abscesses of otic origin may include Gram-negative bacilli such as Pseudomonas aeruginosa and the Enterobacteriaceae (Table 16.12). Abscesses associated with trauma or surgical procedures are often caused by coagulase-negative staphylococci and S aureus (66,67).
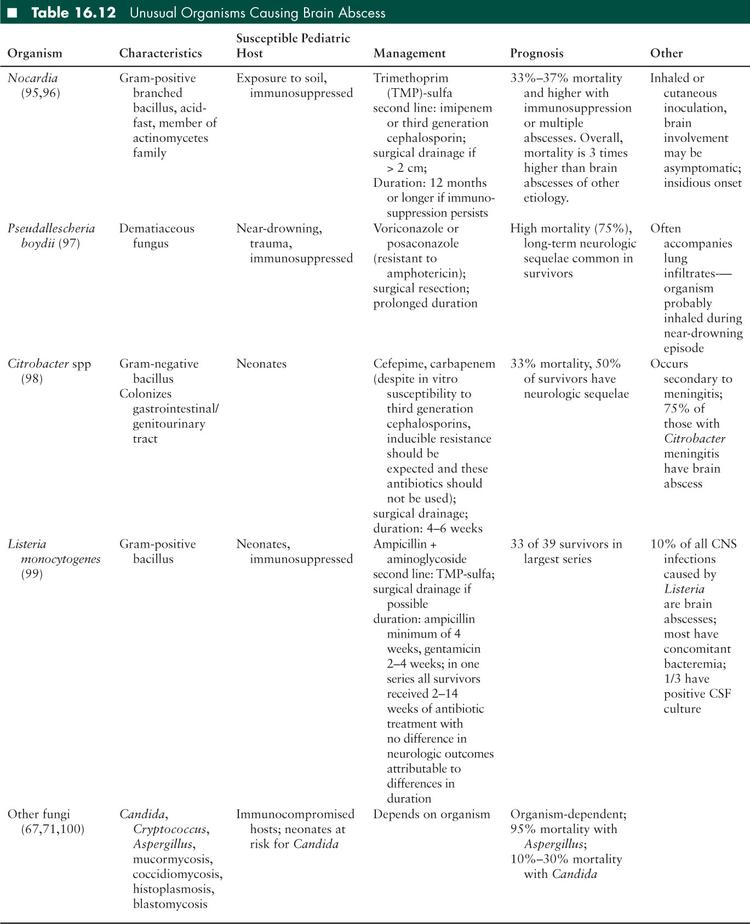
Antibiotics must be both appropriate for the infecting organisms, and also the site of infection. In treating brain abscesses, antibiotics must have appropriate CNS penetration. First generation cephalosporins, clindamycin, and penicillins combined with beta-lactamase inhibitors have very poor CNS penetration. Quinolones and carbapenems have adequate CNS penetration, however, some drugs in these classes can lower seizure thresholds (67). A combination of antibiotics targeted at anaerobes, streptococci, and Gram-negative organisms should be employed for treatment of all brain abscesses. A late generation cephalosporin combined with metronidazole is appropriate empiric therapy. Vancomycin may be added in the case of trauma, postsurgical infection, or other cases in which S aureus is a suspected pathogen. Intraventricular antibiotics may be considered when there is intraventricular rupture of an abscess (66).
The yield of abscess material culture is reported to be 80% to 95% (66,72), and antibiotics may be narrowed to target those organisms identified; however, even with early and fastidious culturing of abscess material, strict anaerobes may be difficult to identify so all regimens should include anaerobic antibiotic therapy.
Corticosteroids have not been shown to cause appreciable difference in outcomes for patients with brain abscess (72) and should be reserved for those situations in which severe brain edema exists. Steroids may inhibit antimicrobial penetration into the CNS and clearance of infection (74). Therefore, corticosteroids should be administered for only short courses, if at all (64,68).
Intravenous antibiotic therapy should be given for approximately 6 to 8 weeks depending on the extent of infection and the organisms involved. Imaging should be repeated every 2 to 3 weeks until resolution of all lesions, and should be repeated immediately in the setting of clinical deterioration (64). Lesions usually resolve after 12 to 16 weeks but complete resolution may not occur for up to 6 months in some cases (66).
Subdural Empyema
SDE is defined as a purulent collection between the dura and the arachnoid meninges. Children with SDE are at high risk of increasing ICP, and therefore rapid neurologic deterioration. Prior to the advent of penicillin in 1944, the mortality rate of SDE was 100%. Currently, the mortality rate for children with SDE is 10% to 20%.
Epidemiology and Pathophysiology
SDE is a life-threatening infection that is primarily associated with otorhinologic infections during the summer months (75). These infections are most common in boys over the age of 7 years, which may be related to rapid growth of paranasal sinuses and related vasculature (76–79). In addition, approximately 2% of infants with meningitis will develop SDE as a complication (77). Less common etiologies of SDE include dental abscesses, superinfection of traumatic hematoma, or infection introduced during neurosurgical manipulation (76). Infants and young children can rarely develop SDE following upper and lower respiratory tract infections (77).
The subdural space is connected to the sinuses and middle ear by valveless diploic veins. Microbiologic organisms may enter this space via transport along these veins, direct intracranial extension of sinus infections through the posterior wall of the sinuses and the epidural space, or by thrombophlebitis of the interconnected venous system (68,77,79). Direct extension occurs more commonly in middle ear infections with associated mastoiditis (68). In infants with meningitis or respiratory infections, SDE occurs after a bacteremic event with seeding of a preexisting subdural effusion or hematoma (77). An alternate form of SDE occurs in infants following superinfection of meningitis-associated subdural effusions. This is seen less frequently since the initiation of routine immunization for pneumococcus and Hib.
Once pus exists in the subdural space, it can spread widely and rapidly over the entire cerebral hemisphere, into the interhemispheric fissure, and to the contralateral hemisphere due to the lack of septations within this space. SDE in pediatric patients is bilateral in 70% to 80% of cases (80). Most cases of SDE are supratentorial; approximately 0.6% are infratentorial and most of these are of otic origin (75). Although subdural pus begins as liquid, it may become organized as infection progresses, resulting in loculation of advanced infections.
As with brain abscesses, the primary site of infection lends clues to the microbiologic etiology. Polymicrobial infection is common in sinus and otic-derived infections. In general, the most common bacteria found in SDE are streptococci, typically of the microaerophilic or nutritionally-deficient variety. Anaerobes can be isolated in up to two-thirds of cases (68), but fastidious collection techniques necessary for isolating anaerobes is both difficult and uncommon so this is likely an underestimate of the true proportion of anaerobic bacteria involved in SDE (Table 16.13).
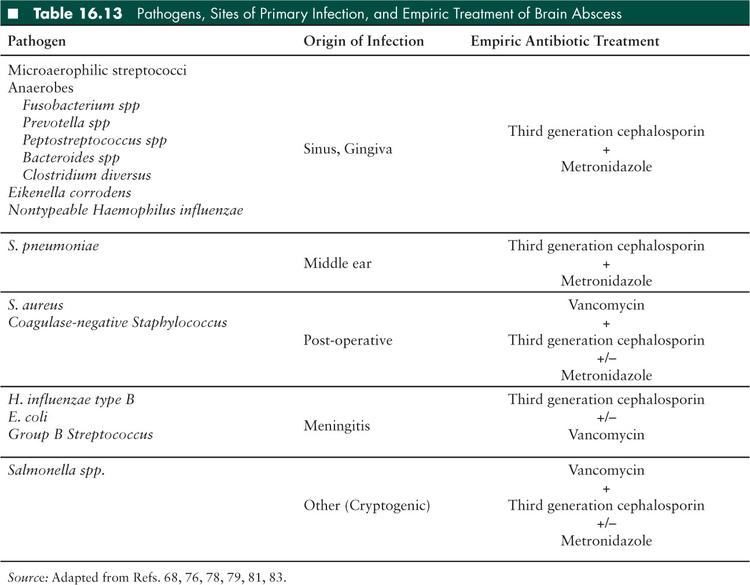
Clinical Presentation
When a microbiologic organism enters the subdural space, it creates an inflammatory response, generating meningeal irritation and purulent exudate, blocking CSF reabsorption at the choroid plexus, resulting in increased ICP. This inflammation and elevated ICP causes headache, emesis, altered level of consciousness, focal neurologic deficits, and may cause seizures (77). New symptoms in a child with a known sinus or ear infection should raise suspicion for intracranial infection. In addition, a relapse of fever, enlarging head circumference, or new neurologic finding in an infant with known bacterial meningitis should prompt evaluation for an SDE. Duration of symptoms preceding diagnosis varies based on primary site of infection. Patients with extension of sinusitis have an average of 14 days of symptoms prior to diagnosis, whereas infants with meningitis and SDE present very acutely (76). Symptoms of primary infection are absent in 25% of patients at time of diagnosis of SDE (81). There may be incisional drainage at the site of postoperative infection.
Diagnosis
Cultures of material collected during surgical intervention are essential in providing guidance for targeted antimicrobial therapy. Blood cultures rarely yield pathogens. LP is generally contraindicated in SDE as ICP is increased. Neuroimaging is imperative in the diagnosis and surgical treatment planning of SDE, and should not be delayed if this diagnosis is suspected. A noncontrast head CT is the most rapid and available form of imaging, and typically demonstrates a hypodense area over the hemisphere or falx; contrast enhancement may help define specific margins. However, CT scanning is reportedly normal in 50% of SDE (76). Gadolinium-enhanced MRI of the brain is the imaging study of choice when available promptly. MRI provides greater morphologic detail and higher sensitivity for fluid collection than CT. For planning and treatment, imaging should include the site of primary infection (paranasal sinuses, mastoids) to aid in surgical planning and to guide empiric antibiotic therapy.
Complications
Complications of SDE include sinus venous thrombus, cerebral edema, herniation, recurrent neurologic deficits, reaccumulation of empyema, and catheter-related complications due to long-term intravenous antibiotics (76–78,80). A 65-case series found sinus venous thrombus as the only statistically significant risk factor for mortality (82). Another series of 38 pediatric patients with SDE reported neurologic deficit and cerebral herniation at presentation as significantly associated with poor outcome (83).
Treatment
SDE is a neurosurgical emergency. Nearly all patients with SDE require at least one neurosurgical procedure; only very rare cases are managed successfully without surgery, and only in cases of well-localized, small collections of pus in minimally symptomatic patients (76,78,83). There remains some controversy surrounding the appropriate initial neurosurgical procedure to drain SDE. Craniotomy appears to have more success and requires fewer repeat operations, although there is a slightly higher risk of intraoperative bleeding and infection (75,82). In patients with significant cerebral edema, decompressive craniectomy may be required. If skull bones are infected, a bone flap removal is necessary. Burr holes are appropriate for drainage of localized collections, early infection when pus is still very liquid, or for patients too unstable to be taken to the operating room. In several large series however, the use of burr holes as initial surgical technique was a risk factor for repeated operation (76,80). In one large series of pediatric patients with SDE, 26% required reoperation, 83% of whom had primary burr hole placement (82). Surgical intervention for the origin of infection should be performed simultaneously with drainage of subdural collections. Early sinus drainage and mastoidectomy contribute to lower mortality and decreased incidence of reoperation in cases of rhinosinogenic SDE (77,79).
Cultures of pus should be obtained during surgical intervention. Cultures are sterile in 7% to 53% of cases (77,78,82) likely due to exposure to antibiotics and difficulty isolating anaerobic bacteria. Generally, empiric antibiotic therapy should be broad and include Gram-positive, Gram-negative, and anaerobic bacteria. Empiric therapy may be tailored to the site of originating infection if it is known at diagnosis. S aureus is a rare pathogen in sinusitis-related intracranial infection, occurring in only 4% to 8% of cases (77), so vancomycin need not be included in empiric therapy unless there are specific concerns for S aureus infection (Table 16.13).
Antibiotics should be tailored to pathogens grown in cultures of subdural pus. Although no randomized studies exist regarding the duration of antibiotics, few treatment failures are observed when intravenous antibiotics are given for 3 weeks following operative drainage (80). Patients with SDE who are managed conservatively without surgical intervention should receive approximately 6 to 8 weeks of intravenous antibiotics (78). Metronidazole is enterally bioavailable and adequately penetrates the BBB, so may be given orally.
Intensive care management should include anticonvulsant therapy either to treat or prevent seizures in cases of severe SDE. Any patient with severe neurologic compromise (Glasgow Coma Score < 8) should be mechanically ventilated for airway protection and monitored with continuous electroencephalogram (EEG) to detect subclinical seizures. Management of intracranial hypertension may require hyperventilation, mannitol, and external ventricular device placement. Corticosteroids should be reserved for only the most refractory cases of increased ICP, as the immunosuppressive effects of steroids are deleterious in treating intracranial infections.
Epidural Abscess
EA is a collection of pus in the potential space between the calvarium and the dura mater. Its origin is similar to those of SDE, however EA-related mortality is low and good neurologic outcomes are expected. EA is an uncommon entity in the developed world due to the early recognition and treatment of otorhinologic infection.
Epidemiology and Pathophysiology
EA is the most common complication of acute bacterial sinusitis. Sixty percent to 90% of epidural space infections occurring in children are a secondary complication of sinusitis or middle ear infections (84). Like SDE, EA occurs more frequently in males, and in patients 7 to 20 years of age (85), probably for similar reasons. Pathophysiologically, a network of valveless bidirectional veins passes from sinuses and mastoid air cells through the epidural space and into the subdural space, thereby creating a passageway for bacteria to travel from extracranial to intracranial structures (Figure 16.1) (84). Less frequently, sinus and mastoid infections extend directly through the skull wall into the epidural space.
The dura mater is tightly adhered to the skull and forms a space only when penetrated. This precludes epidural infections from spreading rapidly and protects the patient from focal neurologic symptoms in most cases. Because of the proximity of this space to the skull, osteomyelitis may be comorbid in EA. Pott puffy tumor is a specific predisposing factor for EA in children and adolescents. Described by Percival Pott in 1760 (76), the “tumor” describes localized swelling of the forehead indicating osteomyelitis of the frontal bone with subperiosteal abscess secondary to frontal sinusitis. Although not always included in this constellation of findings, EA is a common comorbidity.
Children may also develop EA following superinfection of a traumatic head injury. Blood is excellent culture media, so patients with traumatic epidural effusions may develop superinfection of the hematoma therefore creating EA. In cases of superinfected epidural effusions, the hematomas are likely seeded by bacteremic events, which may be transient and asymptomatic, or pathologic. In these patients, staphylococci, streptococci, and Gram-negative bacilli must be considered. Most commonly, EAs are polymicrobial infections. Streptococci are found in a disproportionately high number of cases, and anaerobes are often present in cases of sinogenic origin (Table 16.13) (86).
Clinical Presentation
EA presents more subtly than SDE, often with several days of fever, headache, and neck pain. Mental status change and focal neurologic deficits occur less frequently than in SDE, although seizures may occur (84,85). Extension of sinus disease into the epidural space usually occurs in the frontal region, so frontal headaches may be present. Patients with prior trauma or surgery may suddenly develop fever or erythema and dehiscence at the skin incision.
Diagnosis
The diagnostic evaluation of EA is very similar to that of SDE. Blood cultures should be obtained, as well as abscess culture if surgical intervention occurs. CT scanning is often the first imaging modality used in acute bacterial sinusitis, and may detect a low-attenuation extraaxial collection consistent with EA. MRI with gadolinium is the imaging modality of choice, with 90% to 95% sensitivity and at least 90% specificity in detection. It is better able to clearly delineate abscess from normal tissue, and effectively shows thickening of the dura, which differentiates a purulent collection from a benign effusion (84).
Treatment
Although EA does not pose the same neurosurgical emergency as SDE, surgical management in combination with intravenous antibiotic therapy ensures the best outcomes (80). Craniotomy is preferred as burr holes are associated with abscess recurrence. Small EA less than 2 cm in patients without neurologic compromise may be treated successfully with antibiotics alone (84). Surgical management of osteomyelitis, sinusitis, and mastoiditis should occur concurrently with drainage of EA.
Empiric therapy should include coverage of streptococci, Gram-negative bacilli, and anaerobic bacteria. Staphylococci are infrequently found in EA originating from otorhinologic infection, although should be considered in posttraumatic or postoperative cases of EA. Empiric antibiotics should include a third-generation cephalosporin and metronidazole, and vancomycin if staphylococci are suspected pathogens. In EA originating in the sinuses, narrowing antibiotic coverage based on culture data is not recommended because anaerobic bacteria are difficult to grow in culture and may not be recovered in microbiology specimens (85).
A 3-week course of intravenous antibiotics is appropriate following surgical drainage of EA. In patients managed medically without surgical intervention or those with comorbid osteomyelitis, a 6- to 8-week course of antibiotics is necessary (80,84).
Spinal EA
Spinal location accounts for a small proportion of all epidural infections; in one large pediatric series only 8.6% of 70 patients with extraaxial CNS infections occurred in the spine (76). When present, spinal EA may threaten the spinal cord or cauda equina by either compression, or vascular supply compromise. Spinal EA may occur secondary to spinal manipulation, hematogenous spread from distant sites of infection, superinfected traumatic hematoma, or local extension of vertebral osteomyelitis. Patients typically present with backache and lower-limb weakness (76). Spinal SDE is exceedingly rare.
Spinal EA is often medically managed with success. Surgical intervention is high-risk and reserved for those patients with progressive or concerning neurologic deficits indicative of spinal cord compression (87). Empiric antibiotic treatment is aimed at the organisms responsible for the primary site of infection; because spinal EA is often associated with bacteremic events, S aureus is the most common pathogen, occurring in 60% to 90% of infections, and anaerobes are rarely found. Gram-negative bacilli must be considered (76,86). Vancomycin and a third or fourth generation cephalosporin are appropriate empiric coverage.
Suppurative Jugular Thrombophlebitis
Also known as Lemierre syndrome, suppurative jugular thrombophlebitis describes a well-known syndrome of pharyngitis with septic thrombosis of the internal jugular veins, bacteremia, and possible distant septic metastasis. This entity was first described by Dr Andre Lemierre (88) in 1936 and is commonly caused by Fusobacterium necrophorum, an anaerobic resident of the oral flora.
Epidemiology and Pathophysiology
Oral bacteria cause pharyngitis in superinfection of a viral upper respiratory infection. This pharyngitis rarely extends to the carotid sheath, which encloses both the carotid artery and the internal jugular vein. However when it does, infection and inflammation of those vessels may create a local thrombophlebitis, which can extend both intracranially (to cause cavernous sinus thrombophlebitis), and to distant sites as septic thromboemboli. Metastatic disease most frequently affects the lungs and causes cavitary pneumonia but also may affect musculoskeletal sites, and other distant organs (89).
Lemierre syndrome is rare in the antibiotic era, especially with attention paid by primary care providers to prompt treatment of bacterial pharyngitis in an effort to prevent rheumatic heart disease. One Scandinavian retrospective review estimated a current incidence of 0.8 cases per million people (90). Lemierre is seen most frequently in adolescents and young adults, and has a male-to-female incidence of 2:1 (91). The mortality rate has been reported as 0% to 8% in the postpenicillin era (89,91).
F necrophorum, a Gram-negative anaerobe and oral commensal organism causes 80% of Lemierre syndrome cases (92). However, many other organisms have been described in association with this syndrome including S aureus, Pneumococcus, Streptococcus pyogenes and other streptococcal species, and other Gram-negative bacilli such as Proteus mirabilis (91,93). These organisms enter the pharyngeal space from odontogenic sites via erosions or breaks in the pharyngeal mucosa.
Clinical Presentation
Lemierre syndrome classically presents acutely with fever, chills, shock, and cardiovascular instability in the context of a recent history of resolved pharyngitis. Patients may have pain at the mandibular angle or the sternocleidomastoid muscle, and may have concurrent focal edema. Patients with intracranial extension of septic thrombophlebitis may have cranial nerve palsies.
Diagnosis
Lemierre syndrome is diagnosed based on clinical symptomatology as well as the presence of bacteremia. A thorough history and physical exam, with focus on the head and neck, as well as blood and pharyngeal cultures should be done. In children without a clear source, a thrombophilia evaluation should be considered.
All children with Lemierre syndrome should be evaluated radiographically. Ultrasound is a safe, sensitive, available, and noninvasive modality to visualize a thrombus of the internal jugular vein, however, it is limited in elucidating intracranial extension. The diagnosis of intracranial venous thrombosis is best established with more advanced imaging. Sinus venous thrombosis is best seen on MRI with MR venography, but contrast-enhanced CT is also very sensitive and specific, and is often more readily available. Contrast-enhanced CT typically demonstrates a low density intraluminal thrombus, a sharply defined bright vessel wall, soft tissue swelling surrounding the internal jugular (IJ) vein, and a distended IJ vein proximal to the thrombus. In addition, CT provides information regarding parameningeal foci of infection such as subdural or epidural collections (93).
Treatment
The mainstay of therapy for jugular thrombophlebitis is antibiotic treatment. Most patients do not require ligation of the vessel or other surgical intervention if prompt and appropriate antibiotic therapy is initiated at diagnosis. Empiric antibiotic therapy should include coverage for Fusobacterium spp, as well as other anaerobes (Bacteroides, Peptostreptococcus), all streptococcal species, and S aureus. Metronidazole plus a third or fourth generation cephalosporin plus vancomycin should be initiated upon diagnosis. Antibiotic therapy can be narrowed based on blood culture results, but clinicians should remember that up to one-third of Lemierre syndrome cases are caused by polymicrobial infections (91). Antibiotics should be continued for a minimal duration of 3 to 6 weeks, and patients with intracranial thrombophlebitis and multiple distant metastatic sites should receive longer courses (93).
Patients with Lemierre syndrome often present with septic shock and may require cardiorespiratory support with mechanical ventilation and vasopressors. Given this risk, patients with a clear diagnosis of jugular thrombophlebitis who are not yet in extremis should be admitted to an intensive care unit (ICU) for close observation during the initiation of treatment.
The role of anticoagulation in jugular thrombophlebitis is controversial and is reserved for patients with a large intracranial clot burden, progressive thrombosis despite adequate antibiotic therapy, and no clear contraindications (93).
■ TUBERCULOUS AND OTHER GRANULOMATOUS CNS INFECTIONS
Tuberculous Meningitis
Mycobacterium tuberculosis represents a complex of seven nearly genetically identical species which are slow-growing, fastidious, aerobic bacteria most notable for causing granulomatous disease of the lungs. M tuberculosis also causes an array CNS diseases including meningitis, tuberculoma, and spondylitis.
Epidemiology and Pathophysiology
The most devastating CNS disease caused by M tuberculosis is meningitis. The pathogenesis of tuberculous meningitis begins during primary infection, which is most often transmitted by inhaling organism into the pulmonary tree. From there, the bacillus is hematogenously disseminated and may form small granulomas throughout multiple organs, including the meninges. In time, CNS granulomas, called “rich foci,” form central caseation and eventually rupture, spilling organism into the CSF and causing meningeal inflammation and exudates. The time course of this rupture depends on the host’s immune status, but often occurs 2 to 6 months after initial infection (101).
Three processes lead to the intracranial destruction incurred by M tuberculosis. First, M tuberculosis has a propensity to form gelatinous exudates within the CSF, particularly in the basal portion of the brain including the sylvian fissures, basal cisterns, brainstem, and cerebellum (102). This exudate can coat cranial nerves resulting in focal cranial neuropathy, and/or often creates a significant obstruction to CSF flow resulting in hydrocephalus. The degree of hydrocephalus present at initial diagnosis portends important prognostic factors. Second, obstruction and inflammation of meningeal blood vessels causes vasculitis and infarcts. This may be an early finding or may occur after treatment has been initiated. Third, small tuberculomas may coalesce or expand to form larger lesions that cause mass effect on surrounding tissue.
General epidemiologic risk factors for TB include poverty, crowded living spaces, and living in endemic areas. The highest disease burden of TB exists in Southeast Asia and Africa. In 2008, the five countries with the highest incidence of TB reported were Nigeria, India, China, South Africa, and Indonesia (103).
Tuberculous meningitis is three times more common in children than adults, and children often have more severe disease manifestations than adults (104). Approximately 4% of children with TB develop tuberculous meningitis (105). Children are thought to be at risk due to relative immunologic immaturity. In addition, patients who are immunosuppressed by HIV/AIDS, malnutrition, or immunosuppressive therapy are at a high risk for severe disease caused by TB including tuberculous meningitis.
Clinical Presentation
Patients with tuberculous meningitis present with a spectrum of clinical manifestations, most commonly irritability and focal neurologic deficits. In 1947, the British Medical Research Council described three disease stages of increasing severity (Table 16.14); more severe disease at presentation is directly related to poorer outcomes (106). Stage I involves nonspecific and often subtle neurologic signs without focal deficits. This stage lasts for several weeks and the diagnosis of tuberculous meningitis is usually not made during this time. Stage II is more acute and typical of bacterial meningitis. The hallmark of this stage is focal neurologic findings. Stage III represents late and severe disease, including stupor and coma.
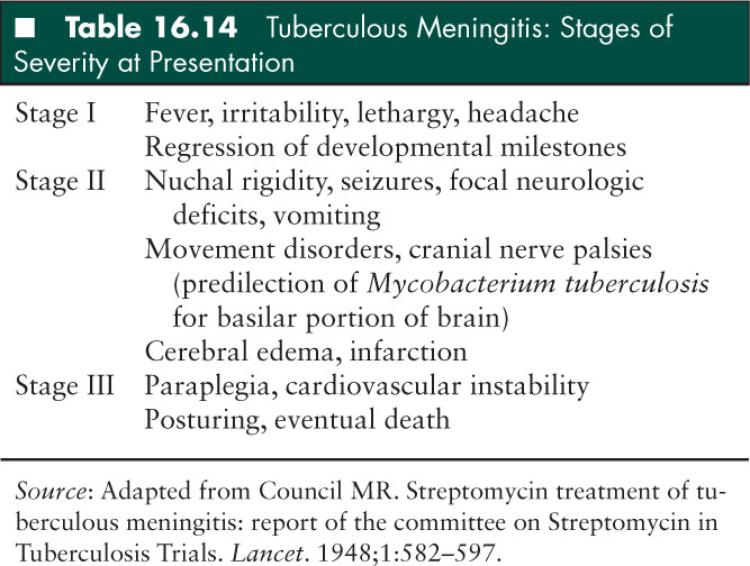
Diagnosis
The differential diagnosis of tuberculous meningitis includes typical bacterial meningitis, viral meningoencephalitis, and other CNS vasculitides such as Behçet disease and sarcoidosis.
The diagnosis of tuberculous meningitis is made difficult by the slow growth rate of M tuberculosis and therefore long time period required for culture positivity of CSF samples. A high level of suspicion must be maintained in order to not delay treatment. Several clinical findings help to distinguish tuberculous meningitis from other causes of CNS infection: (1) illness greater than 7 days at presentation, (2) optic atrophy on fundoscopic exam, (3) focal neurologic deficit, (4) movement disorders, and (5) lymphocyte predominance of CSF WBC (107).
An LP is required for diagnosis, although because patients are at significant risk for cerebral edema, imaging prior to LP should be considered (see Diagnostic Procedures section). OP is usually elevated. CSF sampling yields a lymphocyte-predominant pleocytosis, although neutrophils may be the predominant cell early in the disease process. WBC is generally elevated, but may be normal. Low or normal WBC count is associated with poor prognosis (102). An elevated protein level in the CSF and hypoglycorrhachia are consistent findings (Table 16.3). CT and MRI findings include hydrocephalus, infarcts, and basal meningeal enhancement.
Additional supportive laboratory findings include hyponatremia secondary to metabolic derangement such as cerebral salt wasting or SIADH, and central diabetes insipidus (107). Chest radiography may be normal, or may show signs of active or resolved pulmonary TB including hilar lymphadenopathy, pleural effusion, or calcification. Less than half of patients diagnosed with tuberculous meningitis have an abnormal chest x-ray (105), although this number may be somewhat higher in pediatric patients (104). Tuberculin skin testing is unreliable in diagnosing tuberculous meningitis, especially in early stages of disease. Even in later stage disease, tuberculin skin testing is positive in only approximately half of patients (108,109). Again, this number may be greater in children though, where tuberculin skin testing has been reported to be positive in up to 85% to 90% of children with tuberculous meningitis. Children who are vaccinated with bacilli Calmette-Guerin will have positive tuberculin skin testing transiently following vaccination. The tuberculin reactivity rapidly wanes at 6 to 12 months following vaccination, and reactivity to tuberculin skin testing thereafter is no different between vaccine recipients and those who are unvaccinated (110,111).
Microbiologic diagnosis is traditionally made by large volume CSF culture. A large amount is required due to the paucity of organisms in CSF. Acid-fast stains of CSF are positive in only 5% to 30% of samples (112), but M tuberculosis can be expected to grow in culture within 3 to 4 weeks, and occasionally sooner if a large burden of organisms exists. Alternate body fluid sources such as sputum or gastric aspirate may be positive in cases of suspected tuberculous meningitis and should be examined both to support the diagnosis and for infection control purposes. Nucleic acid amplification testing is now routinely used in the United States and assays are available for CSF. Nucleic acid amplification testing is > 95% specific, but depending on the burden of organisms, may have sensitivity ranging from 44% to 95% (113). Nucleic acid amplification testing is not superior to bacteriologic diagnosis, but may remain positive following empiric treatment for a longer period of time than traditional culture (102).
Complications
Approximately 80% of children diagnosed with tuberculous meningitis will either die or be significantly neurologically impaired (112). However, prognosis is largely determined by stage of disease at presentation. In one retrospective description, all patients who presented in stage I disease had a normal outcome (114). Patients who present in stage II or III disease have a high incidence of mortality, and those who survive suffer significant neurologic sequelae. Permanent neurologic impairment includes movement disorders, vision and hearing impairment, mental retardation, and cranial nerve palsies (105).
Treatment
Any patient with suspected tuberculous meningitis should be immediately empirically treated to prevent disease progression and worsened outcome; treatment should not be delayed until diagnosis is confirmed if a high clinical suspicion for TB meningitis exists. Empiric therapy for tuberculous meningitis in the United States consists of a four-drug regimen of isoniazid (INH), rifampin, ethambutol, and pyrazinamide, given for at least the first 2 months of therapy. Thereafter, patient-specific mycobacterial isolates and accompanying drug susceptibility testing may help narrow the chemotherapeutic regimen. In pan-sensitive isolates, chemotherapy would be narrowed to INH and rifampin. Because M tuberculosis grows slowly in culture, an isolate from the source case to which a child was exposed is frequently identified prior to the growth of that child’s culture, and may be used to guide the child’s therapy until testing of their own isolate is available. Antituberculous therapy should be given for a total of 12 months (107). Multidrug resistant TB may be suspected based on the source case exposure history, or travel to regions with high prevalence of multidrug resistant TB. In this case, empiric therapy may be adjusted to account for the probability of INH or other drug-resistant TB and therapy may be extended for up to 24 months (107).
Adjunctive corticosteroids are recommended in all cases of tuberculous meningitis because they decrease the risk of serious neurologic morbidity and mortality. Dexamethasone or prednisone is typically given for 2 to 8 weeks including dosage taper. If neurologic disability remains significant, duration of corticosteroid treatment may be extended.
If hydrocephalus is significant, patients may require external ventriculostomy or ventriculoperitoneal shunting. Shunts are frequently complicated by obstruction, which is often due to elevated protein and subsequent increased viscosity of CSF in tuberculous meningitis.
Following initiation of treatment, patients are often noted to have worsening hydrocephalus, and new or larger ring-enhancing lesions on brain imaging. Patients may also have increased CSF pleocytosis. This represents a “paradoxic expansion” of disease, which does not require a change in antituberculous therapy or further neurosurgical intervention (104) although some experts recommend continuation of steroid treatment should this occur (112).
TB and HIV Coinfection
Coinfection with HIV and TB is common in the third world. HIV infection increases the risk of the development of TB. Patients with HIV have a high incidence of abnormal chest radiographs and CT scans of the brain, and are prone to falsely negative tuberculin skin testing, making the diagnosis of TB in such patients quite difficult (115). However, small studies have shown that the clinical presentation of TB meningitis is not different in patients with and without HIV infection (116). Treatment for patients with HIV and TB meningitis remains the same as for those without HIV. Immune reconstitution should be instituted when possible, although care must be taken to monitor closely for immune reconstitution inflammatory syndrome (IRIS), which can be fatal in patients with HIV undergoing treatment for TB.
Infection Control
An investigation into the source case should be undertaken for all children with tuberculous meningitis both to identify an isolate for drug susceptibility testing, as well as for infection control purposes. Fifty-one percent to 69% of children diagnosed with tuberculous meningitis have an identifiable close contact with active TB (114). Family members and close contact should undergo tuberculin skin testing and chest radiography as indicated. If any patient contact has active pulmonary TB, he or she should be excluded from visiting the hospitalized child and should obtain an appropriate medical evaluation. Children with tuberculous meningitis who do not have active pulmonary disease are considered noninfectious and do not need to be isolated or placed in a negative pressure environment.
Tuberculoma
Intracranial tuberculomas are rare, large, caseating granulomas that present as mass lesions. The most common clinical manifestation is focal seizures (105), occurring in around 44% of patients (117). Focal neurologic deficits may also be present, depending on the size and location of individual lesions. Tuberculomas may be single or multiple (113).
Contrast-enhanced CT or MRI of the brain reveal ring-enhancing lesions with surrounding edema. The differential diagnosis includes malignancy, brain abscess, sarcoidosis, neurocysticercosis, and toxoplasmosis. Antituberculous therapy should be initiated for any presumed diagnosis of tuberculoma. Surgical biopsy for pathologic examination is often required for definitive diagnosis, although excision of the tuberculoma is not required for successful treatment. The neurosurgical staff should be alerted to the presumed diagnosis of TB because this will require specific preparation of the operating room and sterilization procedures. Chemotherapeutic regimens for tuberculoma are the same as those for tuberculous meningitis (118). Although no trials involving corticosteroid treatment for tuberculoma without meningitis have been done, most experts extrapolate from data regarding treatment of tuberculous meningitis and use corticosteroids in the initial treatment of tuberculoma.
Tuberculous Spondylitis
Vertebral TB, first described by Percival Pott in 1779 who coined the eponym Pott disease, is a primary bone infection affecting vertebral bodies and intervertebral joints. Pott disease is frequently complicated by neurologic symptoms due to close proximity to CNS structures. It represents one-third to one-half of all cases of skeletal TB (113,119). The clinical presentation of vertebral TB is typically more indolent and less severe than primary CNS TB. Vertebral TB, an infection which begins in the bone, must be distinguished from spinal tuberculoma, which arises within the spinal cord, sometimes causing symptoms similar to that of bony disease (102).
Pathophysiology/Epidemiology
The thoracic spine is the most common spinal region to be affected by tuberculous spondylitis due to the spread of mycobacteria from the pulmonary lymphatic system to spinal lymphatics. Other routes of spread include hematogenous dissemination during initial infection and bacteremia, or contiguous spread from other tissue sites.
TB begins at the anteroinferior part of the vertebral body, the destruction of which develops a “wedge” deformity. Vertebral bodies are preferentially affected due to their significant vascularity. Once in the vertebral body, mycobacterial spread occurs along the anterior spinal ligament and may involve contiguous discs and vertebral bodies (119). The posterior portion of the spine is often spared. Paraspinal abscesses occur in approximately 50% of cases and may dissect along tissue planes for a great distance, occasionally affecting distant vertebral bodies (113). Other contiguous sites of abscess reported include epidural, psoas, retropharynx, and gluteal muscles depending on the vertebral level affected (120–122). Intradural manifestations are caused by compression of the cord or nerve roots by edema or abscess.
The development of TB of the spine indicates a prolonged infection with M tuberculosis. In underdeveloped parts of the world and endemic areas, spinal TB is predominantly a disease of adolescents and young adults (113). In the United States and other developed countries, spinal TB affects older patients.
Clinical Presentation
Nonspecific back pain and limb weakness are the most common presenting symptoms. Spinal TB is not often accompanied by systemic symptoms such as fever, night sweats, and weight loss. Focal neurologic deficits such as urinary/fecal incontinence, paraplegia, and sensory loss indicate advanced disease (119).
On physical exam, spinal tenderness and edema may be present. Kyphoscoliosis and other spinal deformities are common in children and may persist or progress despite appropriate antituberculous treatment.
Diagnosis
The diagnosis of tuberculous spondylitis is frequently delayed due to vague, nonspecific symptoms; delay in diagnosis from time of initial presentation may range from 3 weeks to several years (123). Spinal radiographs are typically normal in early stages of the disease; plain radiographs of advanced disease show destruction and collapse of anterior vertebrae and resulting wedge-shape. CT and MRI are more sensitive and reveal abnormalities earlier in the disease course.
When possible, a biopsy of vertebral lesion should be examined for histologic changes and microbiologic evidence of TB including acid-fast smear and culture, and antibiotic susceptibility profile. Pathology specimens will show granuloma formation.
Tuberculin skin test may be positive in spinal TB, but is not diagnostic. Chest x-ray and sputum culture should be examined if the patient has symptoms consistent with pulmonary TB.
Treatment
Treatment for vertebral TB begins with the same 8-week regimen as described for tuberculous meningitis. This should be followed by a period of INH and rifampin, with a total treatment duration of 6 to 9 months. Corticosteroids are not recommended for the treatment of tubercular spondylitis unless is occurs concurrent with tuberculous meningitis.
The role of surgery in the treatment of vertebral TB is largely supportive and probably not required for successful treatment, although a paucity of appropriate trials exists regarding this matter. Surgical management is indicated for spinal stabilization, abscess drainage, and decompression of neurologic structures (119).
Syphilis
Syphilis, one of medicine’s “great masqueraders,” is caused by the spirochete Treponema pallidum. In the pre-penicillin era, syphilis was on nearly every neurologic differential diagnosis because the population prevalence was very high, and it was known to cause a vast spectrum of neurologic disease. However, since the advent of penicillin and more sophisticated diagnostic tools, the incidence and prevalence of syphilis has markedly decreased. Due to these epidemiologic shifts, and the fact that neurologic effects of syphilis occur only after many years or decades of infection, neurosyphilis is rare among children. The important manifestations, diagnostic, and therapeutic tools are described in this section.
Pathophysiology/Epidemiology
As of 2007, 12 million new cases of syphilis were diagnosed each year, 100,000 of which were in North America (124). Children may be infected by syphilis via vertical transmission (congenital syphilis) or as an acquired sexually transmitted infection. The prevalence of congenitally acquired syphilis mirrors the pattern of prevalence of infected women (125). The incidence and prevalence of syphilis dramatically decreased with the availability of penicillin in 1942, although both have again been increasing in the United States over the last 11 years (126), particularly in men who have sex with men (127). Reasons for this are not entirely clear, although it may be related to an increase in unprotected sex among HIV positive individuals (who are now surviving longer with HIV infection, and less afraid of transmitting HIV infection through unprotected sex), and trading drugs for sex.
Spirochetes invade many organs, including the CNS, early in the course of infection when a high burden of spirochetemia exists (128). Seventy percent to 80% of patients clear spirochetes from the CNS early in disease, regardless of treatment, or after a brief meningitis (125,126). However, those who do not clear their initial CNS infection progress to persistent meningitis, and further CNS manifestations. Neurosyphilis may be divided into 2 categories based on time to manifestation. Early neurosyphilis is a meningitis caused by obliterative endarteritis and infarction of small vessels of the CNS, known as meningovascular syphilis (127). This is the primary manifestation seen in pediatrics. Late neurosyphilis irreversibly affects the CNS parenchyma in the form of atrophy, gummas, and progressive vascular disease, and manifests decades after primary infection occurs (125).
Clinical Presentation
Syphilitic meningitis may be symptomatic or asymptomatic. Given the variability in clinical presentation, CSF evaluation should be done when a high index of suspicion exists. Typical symptoms include fever, headache, photophobia, and neck stiffness. Meningovascular syphilis presents with focal neurologic deficits dependent on the specific location of ischemia. Cranial nerve palsies, seizures, and altered mental status are common (129). Additionally, patients may have independent otic or ophthalmologic involvement.
Neurologic manifestations of congenitally acquired syphilis occur either early in life as aseptic meningitis or, more commonly, at age 2 to 3 years as deafness, cranial neuropathies, seizures, or other symptoms consistent with meningovascular syphilis.
Diagnosis
Systemic syphilis is diagnosed by a sensitive but nonspecific screening serologic test (Venereal Disease Research Laboratory [VDRL] or rapid plasma reagin [RPR]); followed by a confirmatory treponemal test such as fluorescent treponemal antibody-absorption. CSF examination is indicated for any patient with a serologic diagnosis of syphilis and neurologic symptoms or for patients who do not respond with an appropriate decrease in RPR titer after treatment (fourfold or greater decline 6 to 12 months after therapy) regardless of neurologic symptoms. CSF testing often demonstrates lymphocytic pleocytosis ranging from 5 to 2,000 cells/mL depending on the stage of infection (Table 16.3), but in general, CSF testing is insensitive. CSF may be normal early in the course of infection, and CSF VDRL is negative in up to 70% of patients with neurosyphilis (130). Centers for Disease Control (CDC) diagnostic criteria are shown in Table 16.15 (126). CSF should be reexamined every 6 months until abnormalities have resolved (126).
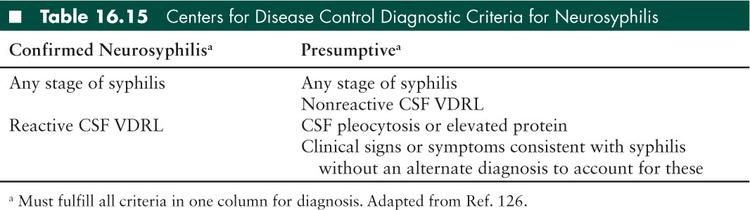
All infants being evaluated for congenital syphilis based on clinical suspicion or maternal history should undergo LP (125). CSF findings are similar to those of older children and adults including pleocytosis and elevated protein, although these abnormalities may not be present if LP is done early in the course of infection (128).
Treatment
Penicillin is the standard of care for patients with neurosyphilis, however small studies have suggested that intravenous ceftriaxone 2 grams daily may be equally efficacious (130). Benzathene penicillin, recommended for nonneurologic syphilis, does not provide spirocheticidal levels of antibiotic in the CSF (129), so the recommended agent to treat neurosyphilis is aqueous crystalline penicillin G 2 to 4 million units every 4 hours intravenously for 10 to 14 days. An alternate regimen of penicillin G procaine 2.4 million units per day intramuscularly for 10 to 14 days is approved and should be given with oral probenecid 500 mg 4 times daily. The treatment for congenital syphilis is aqueous crystalline penicillin G 50,000 units/kg every 12 hours for 1 week, then every 8 hours for 3 weeks, then every 4 to 6 hours for 10 to 14 days (128).
■ ENCEPHALITIS AND MYELITIS
Inflammation of the brain or spinal cord parenchyma on histopathologic examination associated with clinical neurologic dysfunction formally defines encephalitis or myelitis, respectively. However the diagnosis of these entities in practice rests primarily on clinical presentation and history, since tissue is rarely obtained for pathologic examination. For the purposes of this section, encephalitis will be defined as acute CNS dysfunction (seizure, focal neurologic deficits, or altered mental status) in the context of laboratory or radiographic evidence of brain parenchymal inflammation. Myelitis will be similarly defined with respect to the spinal cord. Both encephalitis and myelitis may be caused by a host of inflammatory etiologies, including infectious, neoplastic, toxic/metabolic, vascular, or autoimmune disorders.
There is some inherent overlap in the clinical presentation of primary encephalitis and myelitis with meningitis, since inflammation of the brain or spinal cord frequently involves the meninges, and primary inflammation of the meninges frequently spreads to involve adjacent brain parenchyma (131). However, in this section, we will consider these separate entities, and aim to broadly discuss the epidemiology, diagnosis, and treatment of primary infectious encephalitis and myelitis based on various clinical presentations. Finally, a brief discussion of non-infection-related primary encephalitis and myelitis will follow.
Primary Infectious Encephalitis
There are at least two different forms of infection-related encephalitis/myelitis: primary infectious encephalitis/myelitis, and postinfectious (or immunologically mediated) encephalitis/myelitis (i.e., acute disseminated encephalomyelitis [ADEM]) (132). While the clinical presentation of these two entities may be similar, the distinction in pathogenesis is important because clinical course, treatment, and prognosis may differ significantly (133). Primary encephalitis results from direct parenchymal invasion of the offending pathogen and largely affects the gray matter, whereas postinfectious encephalitis is immunologically mediated and more prominently affects the white matter (132). This section will discuss primary infectious encephalitis and myelitis only. For a discussion of immunologically mediated encephalitis, please see the chapter on “Acquired Demyelinating Syndromes of the Central Nervous System.”
Epidemiology and Pathophysiology
Primary infectious encephalitis causes an estimated 7.3 hospitalizations per 100,000 people in the United States annually, leading to about 1,400 deaths per year, predominantly in children and the elderly (132,133). The true incidence of encephalitis in the United States population in unknown, but probably ranges between 2.6 and 27.7 cases per 100,000 child-years (134,135); the highest rates occur in children less than 1 year of age. Among cohorts of children 1 to 18 years of age, the mean incident age of encephalitis is 6 years (135).
The pathogenesis of primary infectious encephalitis varies broadly by the etiologic organism, but overall is poorly understood. Some organisms enter the CNS from the bloodstream following systemic viremia via infected cells allowed across the BBB (HIV), or via infection of capillary endothelial cells (arboviruses). Other organisms enter the CNS via retrograde transport in infected axons (rabies). Finally, others may enter directly via the olfactory mucosa (N fowleri) or following trauma. Once in the CNS, different organisms have different tissue tropisms, and thus clinical manifestations vary based on the site of significant tissue invasion (132).
More than 100 different pathogens have been associated with primary infectious encephalitis (134,136), and common culprits have evolved over time as vaccine-preventable etiologies diminish (measles, mumps, rubella), newer pathogens emerge (West Nile virus, Nipah virus), and new laboratory methods are developed for pathogen detection (132). In general, viruses, particularly herpesviruses and arthopod-borne viruses (arboviruses), are the most common identified etiologies of encephalitis. However, bacteria (M tuberculosis, L monocytogenes), fungi, parasites, and other noninfectious agents may also be responsible (Table 16.16) (132). The frequency and distribution of etiologic agents varies with geographic region and climate, epidemics, arthropod presence, and variations in immunization programs (136). In a prospective study of 1,570 cases referred over 7 years to the California Encephalitis Project (CEP), only 16% of encephalitis cases had a confirmed or probable infectious etiology (69% viral, 20% bacterial, 7% prion, 2% parasitic, 1% fungal); 13% had a possible infectious etiology, and 8% had a noninfectious etiology (such as neoplasm, vasculitis, metabolic disorders, etc.) (137). Perhaps most striking however, is that no etiology was found in 63% of cases (137). Another prospectively observed cohort of 253 subjects in France (10% of whom were pediatric), who were hospitalized for at least 5 days, found an infectious etiology in only 52% (138). Of these, 69% were viral, and 30% were bacterial (10% Listeria, 15% M tuberculosis). An epidemiologic summary of encephalitis is organized by infectious etiology in Table 16.16 by specific historical clues or exposure in Table 16.17.
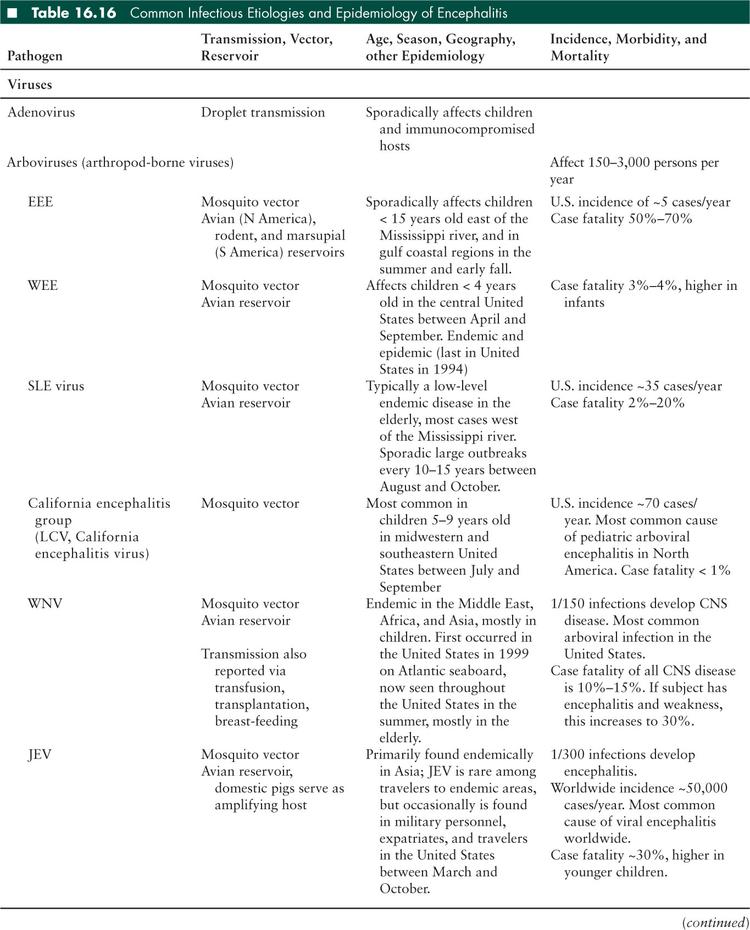
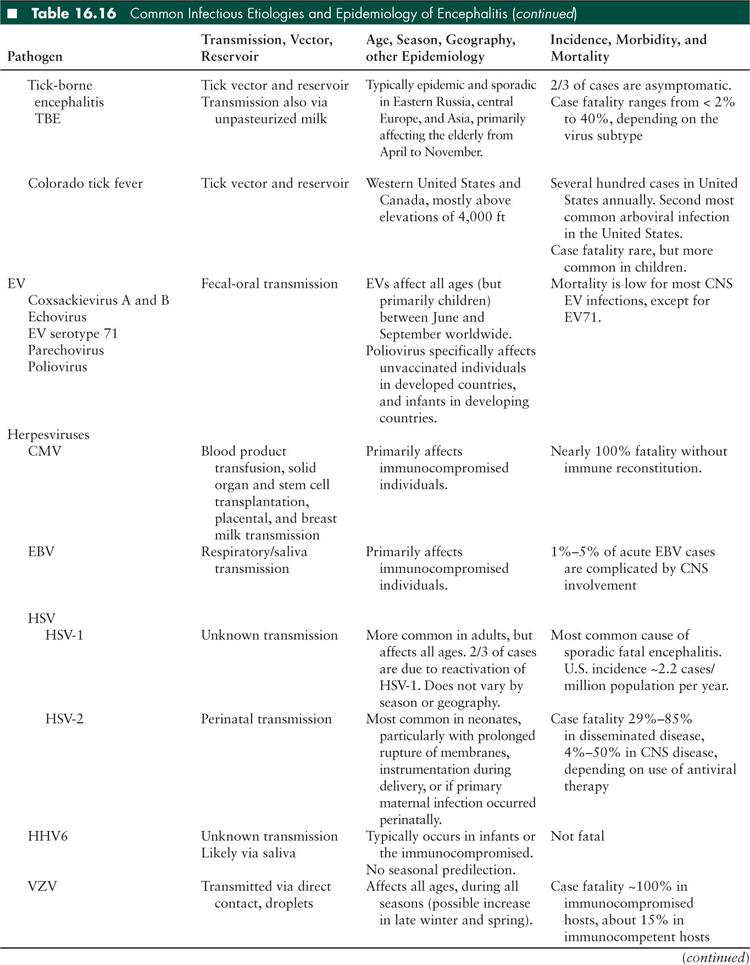
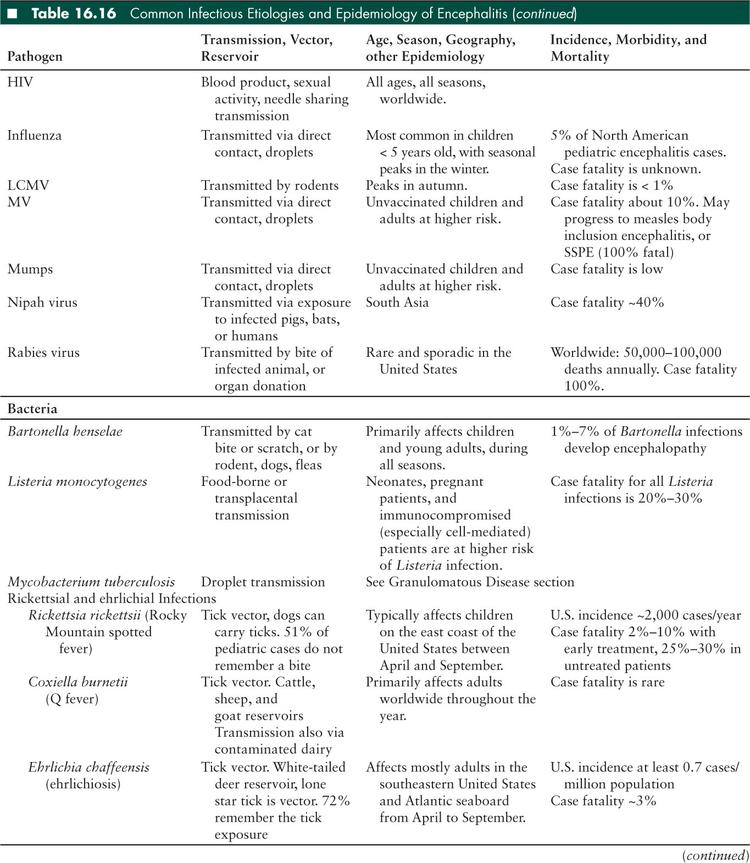
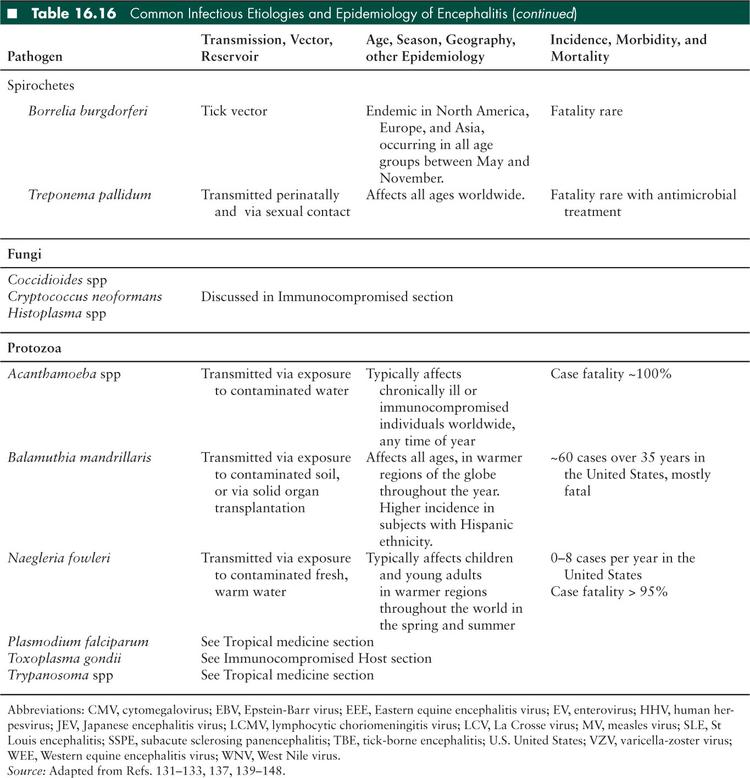
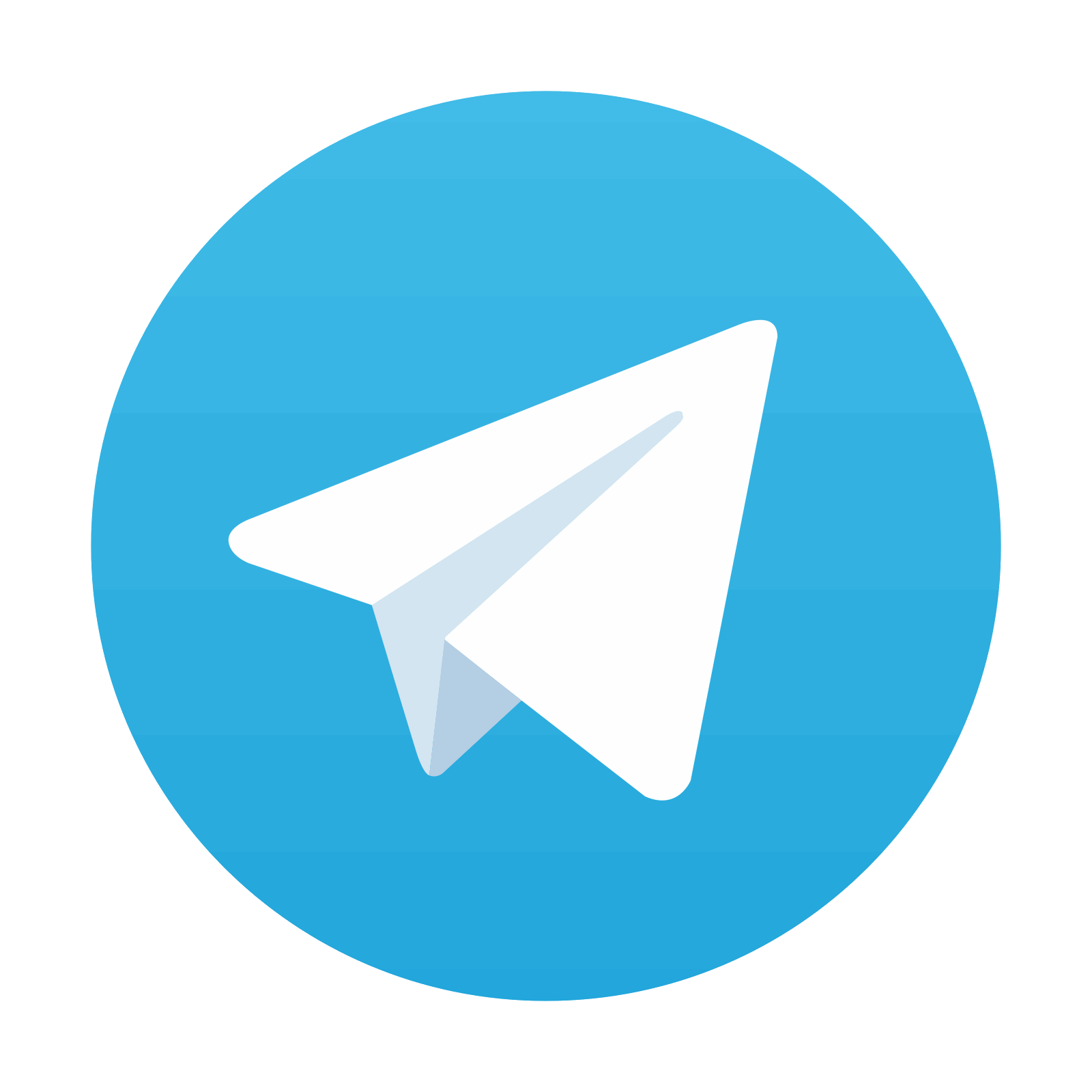
Stay updated, free articles. Join our Telegram channel

Full access? Get Clinical Tree
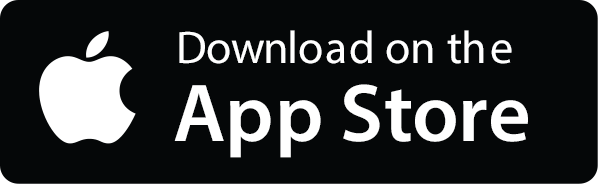
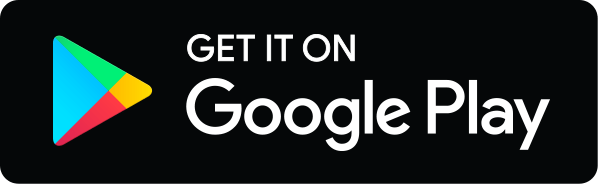