Cardiovascular Physiology of Pregnancy and Clinical Implications
Jessica Spiegelman, Marie-Louise Meng, Jennifer Haythe, and Dena Goffman
•Pregnancy can expose undiagnosed cardiac pathology, or it can cause cardiac pathology in otherwise healthy people
•Heart rate, blood volume, and cardiac output all increase, while systemic vascular resistance and blood pressure decrease
•Heart rate >100 beats per minute in pregnancy should be regarded as abnormal until proven otherwise
•Many of the symptoms of normal pregnancy are similar to the symptoms of cardiac disease
•A systematic approach is required to evaluate any pregnant or postpartum patient with suspected cardiovascular disease in order to differentiate physiologic change from a pathologic process
Introduction
Pregnancy is unique as the only non-pathologic state that causes dramatic alterations in physiology. The integral components of pregnancy physiology that promote fetal growth and development are cardiovascular adaptations [1]. The maternal heart undergoes significant structural and functional changes beginning soon after conception that evolve as the pregnancy progresses to serve the changing demands of the developing fetus and in anticipation of blood loss at delivery [2]. Disruptions in this physiologic system may lead to both maternal and fetal morbidity at all stages of pregnancy. In the intrapartum period, increased perfusion of the uterus, pain-related tachycardia, and fluid shifts lead to additional hemodynamic variation. Most of these cardiovascular adaptations of pregnancy return to baseline in the postpartum period; however, some may persist for up to 6 months after delivery, emphasizing the need for maternal care in the “fourth trimester.”
Because many of the cardiovascular adaptations of pregnancy are marked departures from the nonpregnant physiology, pregnancy may unmask previously unrecognized cardiac disease such as rheumatic mitral stenosis that can lead to significant morbidity and mortality. Not surprisingly, cardiovascular disease remains the leading cause of maternal mortality in the United States [3]. Normal pregnancy symptoms may overlap with those of cardiovascular disease. An understanding of the maternal adaptations that may elicit or exacerbate cardiovascular disease is essential for any obstetric provider. Appropriate triage and follow-up is essential in women with cardiovascular disease during pregnancy and the postpartum period.
Cardiovascular Physiologic Adaptations
The hemodynamic parameters that adapt during pregnancy include heart rate (HR), systemic vascular resistance (SVR), blood volume, cardiac output (CO), and blood pressure (BP) (Box 3.1). Each parameter is reviewed and the interplay between them is demonstrated in Figure 3.1. Most changes begin in the first trimester, peak in the late second or early third trimester, and then plateau for the remainder of pregnancy with a return to pre-pregnancy values during the postpartum period [4].
Box 3.1 The Hemodynamics of Uncomplicated Pregnancies: Antepartum, Intrapartum, and Postpartum Changes
| Heart Rate | Systemic Vascular Resistance | Plasma Volume | RBC Volume | Cardiac Output | Blood Pressure |
1st trimester | Increase | Decrease | Increase | Increase | Sharp increase | Decrease |
2nd trimester | Steady increase | Rapid decrease | Rapid increase | Steady increase | Slower increase | Continued decrease, nadir |
3rd trimester | Steady increase | Slight increase after 32 weeks | Slower increase after 28 weeks | Steady increase | Plateau | Increase |
Intrapartum | Continues to increase | No significant change | Decrease due to blood loss | Decrease due to blood loss | Increase | Increase |
Postpartum | Return to pre-pregnancy within 6–48 hours | Return to pre-pregnancy values soon after delivery | Vaginal delivery: decrease over 10 days Cesarean delivery: no change | Vaginal delivery: decrease over 10 days Cesarean delivery: no change | Rapid decrease in first 2 weeks, slower decrease for up to 6 months | Return to pre-pregnancy, unknown time frame |
Earliest change | 5 weeks | 5 weeks | 6 weeks | 8–10 weeks | Before 8 weeks | 7 weeks |
Peak/nadir above/below pre-pregnancy values | +20%–25% | −30% | +45% | +20%–30% (with iron), +15%–20% (no iron) | +30%–50% | −5–10 mmHg (systolic); up to −15 mmHg (diastolic) |
GA at peak/nadir | Intrapartum | 32 weeks | 32 weeks | Term | 25–35 weeks | 20–24 weeks |
Heart Rate
Maternal HR increases steadily throughout pregnancy and has been shown in some studies to be the first hemodynamic parameter to change after conception as early as 5 weeks of gestation [5,6]. Unlike most other hemodynamic parameters, which change at rapid rates until a plateau point in the second trimester, HR increases steadily throughout pregnancy [2]. Peak increase in HR is generally between 10–20 beats per minute (bpm), or 20%–25%, above pre-pregnancy values [7]. The upper limit of normal heart rate in pregnancy is generally no higher than 95 bpm, and therefore heart rates >100 bpm should be regarded as abnormal until proven otherwise [2]. Later in pregnancy, this increase in HR is the primary contributor to the rise in CO. Maternal position may cause variations in HR, with slightly lower rates noted in the left lateral position than in the supine position, most likely due to alterations in preload with position changes [8]. HR typically returns to pre-pregnancy values in the immediate postpartum period, that is, within 6 hours postpartum [9].
Systemic Vascular Resistance
The decrease in SVR occurs as early as 5 weeks of gestation and prior to full development of the placenta [4]. In the first trimester, SVR decreases by about 10%, primarily due to the vasodilatory effects of progesterone, estrogen, prostaglandins, and relaxin [10]. Increased nitric oxide production in pregnancy likely also plays a role in the decrease in SVR [11]. As the placenta develops, it further decreases the SVR by adding a high-flow, low-resistance component to the maternal circulation. Decreased SVR leads to a decrease in BP, more specifically diastolic BP, and a widening of pulse pressure [12].
Decreased SVR in the renal vasculature activates the renin-angiotensin-aldosterone (RAA) system. Increased angiotensin leads to salt and water retention at the level of the kidneys and thus to an increase in blood volume and maintenance of blood pressure [13]. Decreased SVR is therefore at least partially responsible for an increase in blood volume and stroke volume. The combination of decreased SVR and increased HR leads to the increased cardiac output (Figure 3.1), with a larger contribution from HR in later pregnancy. SVR nadirs in the second trimester at about 30% lower than the baseline, and then plateaus until about 32 weeks, after which time it increases slightly [14]. At term, SVR remains up to 21% lower than pre-pregnancy values [15]. The pattern of SVR change during pregnancy is generally inverse to that of cardiac output, leading to a minimal change in the mean arterial pressure [14].
Blood Volume
Increased blood volume in pregnancy is evident from studies dating as far back as the 1930s [16]. Both plasma and red blood cell (RBC) expansion contribute to the total blood volume increase of pregnancy. Plasma volume expansion is primarily mediated by the upregulation of the RAA system, which begins as early as 6 weeks’ gestation and continues to increase rapidly throughout pregnancy, with the steepest increase in the second trimester [17]. After 28 weeks, the rate of plasma volume increase slows; plasma volume reaches its peak at approximately 32 weeks’ gestation [18]. To quantify plasma volume expansion in pregnancy, a 2017 meta-analysis of 30 studies found that plasma volume rose by 180 mL in the first trimester, by 570 mL in the second trimester, and by 1090 mL by 35 weeks. By 41 weeks’ gestation, the total plasma volume expansion was 1130 mL above pre-pregnancy values [19].
RBC expansion is in part the result of a progesterone-driven increase in erythropoietin and helps support the increased oxygen demand of pregnancy [20,21]. RBC mass increases beginning at 8–10 weeks’ gestation and steadily rises, reaching a 20%–30% increase by the end of pregnancy in women taking iron supplementation, or a 15%–20% increase in women not taking supplemental iron [22].
Although both plasma and red blood cell volume increase during pregnancy, plasma volume increases more rapidly than erythrocyte volume, leading to the dilutional anemia of pregnancy [23]. In one study, normal reference ranges for maternal hemoglobin were shown to be from 11.6–13.9 g/dL in the first trimester, 9.7–14.8 g/dL in the second trimester, and 9.5–15 g/dL in the third trimester; with 12–15.8 g/dL used as a reference range for nonpregnant females [24]. While some degree of “anemia” is considered normal in pregnancy, maternal hemoglobin levels below these values may result from insufficient iron intake to meet the demands of increased erythropoiesis. Pregnancy-related anemia responds well to iron supplementation. Other causes of anemia should be ruled out [25].
Clinical Implications
1.Anemia may lead to adverse obstetric outcomes including low birth weight, therefore attention to hemoglobin levels in pregnancy is prudent for prevention of maternal and fetal morbidity [18,26].
2.Conversely, the absence of physiologic anemia may be detrimental. Hemoglobin levels above 14.6 g/dL in early pregnancy are associated with an increased risk of intrauterine fetal demise [27].
Increasing blood volume traversing through the heart leads to an increased atrial natriuretic peptide (ANP), released in response to atrial stretch. ANP acts as a peripheral vasodilator and a diuretic, thus exerting a check on volume expansion and contributing to a further decrease in SVR in order to compensate for the increase in blood volume [28]. Increased total blood volume during pregnancy serves several purposes: (i) To meet the increased metabolic demands of the gravid uterus and its enlarged vasculature with up to 750 mL/min directed to the uterus at term gestation [29]. (ii) It prepares maternal blood volume for the blood loss experienced at delivery. (iii) Increased blood flow to the placenta provides the fetus with the oxygen and nutrients necessary for normal development.
Clinical Implications
1.The degree of blood volume expansion is proportional to birth weight in multiple studies, and diminished plasma volume increase has been associated with preeclampsia and growth restriction [30].
2.Fetal growth restriction has been shown to be preceded by inadequate volume expansion even as early as 8 weeks of pregnancy [31].
Cardiac Output
CO is the product of stroke volume and HR that reflects the capacity of the heart to respond to the perfusion requirement of the body. CO has been the focus of many studies assessing the hemodynamics of pregnancy that have universally shown a rise during pregnancy. Measurement of CO can be either invasive or noninvasive. Invasive methods using thermodilution technique remain the gold standard for calculating cardiac output. Many studies have understandably focused on identifying noninvasive techniques that can best replicate the measurements obtained invasively, such as 2D or Doppler echocardiography [32–34]. Due to differences in measurement techniques and feasibility of obtaining measurements, the precise degree of the CO increase and the mechanisms behind it have not been universally agreed upon [35]. Studies of normal maternal physiology in relation to these changes in CO remain an important area for future research.
Typically, the sharpest rise in CO occurs by 8 weeks, with continued increase into the second trimester and a peak between 25–35 weeks [36]. In some studies, CO has been shown to be increased by 50% during pregnancy; however, there is no consensus in the literature regarding the precise magnitude and pattern of change in CO. A 2016 meta-analysis looking at 39 studies assessing CO data in uncomplicated, singleton pregnancies showed that CO increased by 1.5 L/min, or 31%, above nonpregnant values [14]. This increase was steady, though non-linear, until its peak at 31 weeks, with a subsequent plateau. In early pregnancy, stroke volume was the major contributor to the increase in CO, and in late pregnancy, HR became increasingly influential [14,35].
Maternal position affects CO, which has implications during obstetric procedures, imaging studies, and during labor and delivery. In the supine position, the gravid uterus compresses the inferior vena cava, decreasing venous return to the heart and causing a significant decrease in CO [8]. The magnitude of decrease correlates with the size of the uterus, as is evident from twin studies [37]. Evidence has shown that in twin gestations, CO is 20% higher than in singleton pregnancies, and that compression of the vena cava by the uterus is even more pronounced [38]. CO is directly related to weight and height, with an increase in CO noted as weight increases in women above 160 cm in height. CO is inversely related to age, with higher CO in women under the age of 25 [39].
The need to augment CO in pregnancy and delivery is a major contributing factor to the potential decompensation of women with cardiac disease if this augmentation is compromised due to the underlying cardiac structure and function.
Blood Pressure
Despite marked blood volume expansion, pregnant women usually remain normotensive throughout pregnancy, and have been traditionally thought to have an overall decrease in BP [40]. Data from longitudinal studies have demonstrated that, like SVR, arterial BP begins to decrease in the first trimester, as early as 7 weeks [41]. BP nadirs in the second trimester, but the majority of the decrease in BP occurs at 6–8 weeks. It is therefore important, if possible, to compare pregnancy BPs to true preconception BPs, as BPs in early pregnancy are likely already displaying the effects of pregnancy [2]. Systolic blood pressure, diastolic blood pressure, and mean arterial pressure all decrease, but diastolic blood pressure and mean arterial pressure decrease more than systolic blood pressure, leading to a widened pulse pressure [12]. In the third trimester, BP begins to increase, and it returns to pre-pregnancy values in the postpartum period, though the precise timeline of BP normalization is not clear.
Some recent studies have challenged the traditional thinking that BP is lower in pregnancy than in the nonpregnant state. A study of third trimester BP from 2016 showed significantly higher systolic and diastolic BPs in pregnant compared to nonpregnant women, by 18 and 12 mmHg, respectively [42]. In 2002, a retrospective study of 1599 women showed that women with a high initial BP had a fall in BP during pregnancy, while those with low initial BP had either a much smaller fall or a rise in BP as pregnancy progressed [43]. In addition, a 2011 study demonstrated a progressive increase in blood pressure throughout pregnancy [44]. The Generation R study, meanwhile, demonstrated that women with lower education levels did not experience a mid-pregnancy fall in diastolic blood pressure, and this difference was not explained by body mass index or weight change during pregnancy [45]. These factors should be taken into consideration when assessing maternal blood pressure.
Correct patient positioning, timing, and cuff size are all extremely important in the measurement of blood pressure.
•Blood pressure should be taken after the patient has rested, ideally for at least 10 minutes.
•The patient should be seated with her legs uncrossed and her back supported, and her arm should be positioned at the level of her heart.
•If blood pressure must be taken in a recumbent position, which is often the case in the intrapartum setting, the patient should be placed in a left lateral decubitus position and the cuff should be at the level of the right atrium.
•An appropriately sized cuff has a length that is 1.5 times the upper arm circumference, or a bladder that encircles at least 80% of the arm and a width of at least 40% of the arm circumference [46].
•BPs are generally higher in the sitting position than in the recumbent position, because compression of the vena cava by the gravid uterus in the supine position leads to a decrease in venous return and therefore cardiac output. Normally, there is a compensatory increase in peripheral vascular resistance that helps maintain the maternal BP; however, occasionally supine position may lead to an increase in HR and decrease in BP which may result in significant dizziness, lightheadedness, nausea, or syncope [8]]. These hemodynamic changes in response to positioning must be kept in mind when evaluating a pregnant patient.
Cardiac Structure
Pregnancy and exercise are the two non-pathological states known to cause cardiac hypertrophy [48]. In pathologic cardiac hypertrophy, pressure and volume overload lead to either concentric or eccentric changes in ventricular geometry. Concentric hypertrophy is an increase in ventricular wall thickness with decrease in ventricular cavity size that may lead to diastolic heart dysfunction, and eccentric hypertrophy is a thinning of the ventricular wall leading to a dilation of the ventricular cavity with potential for subsequent systolic cardiac dysfunction. These states often lead to decreased cardiac function and heart failure over time. In contrast, exercise and pregnancy lead to a proportional increase in both wall thickness and chamber size [49]. Cardiac hypertrophy in pregnancy is mediated both by the increase in blood volume and circulating levels of sex steroid hormones [50]. Progesterone in particular tends to increase protein synthesis and thereby induce hypertrophy in cardiac muscle cells [51]. There is an increase in left ventricular wall thickness of up to 28% by the end of pregnancy, and an up to 52% increase in left ventricular wall mass [41,52]. Right ventricle similarly has been shown to undergo an increase in wall mass of up to 40% [5].
The effects of pregnancy on left ventricular function are not well understood. Both normal and decreased ventricular systolic function has been reported in the literature [53]. Mild LV diastolic dysfunction has also been reported during normal, otherwise uncomplicated pregnancies [54]. However, pregnancy-induced cardiac hypertrophy is similar to exercise-induced hypertrophy and differs from pathological hypertrophy in that it is reversible, with studies showing a normalization of heart size starting from 7–14 days up to 1 year postpartum [55].
The cardiac valves demonstrate effects of pregnancy as well. In animal studies, all four cardiac valves undergo remodeling throughout gestation, with increased leaflet size and a change in the biochemical composition to include a decrease in cell density and in collagen composition [56]. Valve area may increase dramatically, especially in the aortic and mitral valves, secondary to the increase in blood volume seen by the left ventricle [57]. This increase in valve annulus area with or without mild leaflet elongation can result in mild physiologic valvular insufficiency or regurgitation.
Clinical Implications
1.Pregnancy can expose undiagnosed cardiac pathology or it can cause cardiac pathology in otherwise healthy people.
2.Though the underlying etiology of peripartum cardiomyopathy is still a matter of investigation and is not the focus of this chapter, this condition illustrates the extent to which pregnancy functions as a cardiac stress model.
3.Peripartum cardiomyopathy, or heart failure near the end of pregnancy or within 5 months after delivery without any other identifiable cause, may represent a previously healthy cardiovascular system now unable to respond to the cardiac stress of pregnancy [48]. Alternately, it may reflect a heart with an underlying undiscovered insufficiency or be the result of unbalanced pregnancy hormones [58].
Intrapartum and Postpartum
The cardiovascular changes during labor and delivery are mediated by the autonomic nervous system. These changes occur more rapidly and have more pronounced effects than those that have been evolving gradually throughout pregnancy, and their clinical impact may be significant [59,60]. The blood loss experienced at time of delivery, as well as the contribution of anesthesia and surgical stress in patients who undergo cesarean section, also have profound effects on maternal hemodynamics. Though women with previously recognized cardiac disease require especially close monitoring in labor, even low-risk women can suffer adverse cardiovascular events during this time, and all obstetric providers should be familiar with the possible complications that can occur.
Hemodynamic Changes in Labor
An increase in cardiac output during labor has been long reported in the literature, dating back to the 1950s. In 1956, Hendricks and Quilligan studied changes in CO during late pregnancy, labor, and in the early postpartum period. They concluded that cardiac output increases by an average of 30.9% during contractions, with pain and anxiety contributing to this increase [61]. They additionally described a cumulative 35% increase in cardiac output over the course of prolonged labor. In 1958, Adams and Alexander used a dye-dilution technique to demonstrate that CO increases with each uterine contraction during labor and immediately after the uterus has been emptied, corresponding with an increase in heart rate. They also found that in patients whose heart rate decreased, or who had saddle block anesthesia (mitigation of pain in the sacral region, likely resulting in less increase in HR), CO decreased [62].
Subsequent investigations using various techniques of measuring cardiac output have shown similar findings [63,64]. A study by Robson et al. from 1987 showed an intrapartum increase in cardiac output from a pre-labor value of 6.99–7.88 L/min between contractions. This change was due entirely to an increase in stroke volume, as heart rate did not increase. During contractions, CO increased even more. The rate of increase was incremental as labor progressed, with higher CO demonstrated at more advanced stages of cervical dilation [65]. Autotransfusion of blood into the systemic circulation from the uterine sinuses during contractions may contribute to this increase, as may elevated HR from pain and anxiety [66]. However, even with good neuraxial analgesia and no pain, HR elevates during labor and delivery, indicating that autotransfusion and increase in preload likely contribute most to increased HR [67]. Increased CO also leads to an increase in both systolic and diastolic blood pressure during each contraction, as SVR changes only slightly in the intrapartum period [68].
As in the third trimester, maternal position has an impact on CO in labor, with CO between contractions is higher in the lateral position than supine [69]. Anesthesia also plays a critical role in intrapartum hemodynamics. Women with epidural anesthesia have been shown to have a significantly less dramatic increase in CO between contractions compared to those without regional anesthesia. The peak CO during contractions is not affected by epidural anesthesia. Regional anesthesia also has the potential to cause profound hypotension after placement, and this can in turn affect cardiac output [70].
Despite a multitude of evidence in older studies demonstrating an intrapartum increase in CO, newer data using more modern technologies have not consistently produced the same results. The LiDCOplus monitor calculates beat-to-beat stroke volume from the arterial pressure waveform obtained from an arterial line, and calibrates it using thermodilution; it can thus continuously calculate CO [71]. This method has been shown to be comparable to pulmonary artery catheter measurements in certain patient populations [72]. A 2017 study by Kuhn et al. used this technique for continuous monitoring of maternal hemodynamics in 20 healthy women with conflicting results in the first stage of labor. In the second stage, there was a decrease in CO and SVR and an increase in the remaining metrics for all patients [73].
Changes during the Second Stage
Bearing down to push during the second stage of labor has many similarities to the Valsalva maneuver, which is technically defined as expiration against a closed glottis after full inspiration. The Valsalva maneuver is commonly used in the assessment of patients with cardiovascular disorders. It can be divided into four phases:
•Phase I: Initial straining leads to increased intrathoracic pressure, followed by a transient increase in systemic blood pressure. HR remains stable.
•Phase II: A decrease in venous return which leads to a gradual decrease in right and left ventricular volumes, mean arterial pressure, pulse pressure, and stroke volume as well as reflex tachycardia.
•Phase III: Release of straining leads to an abrupt decrease in intrathoracic pressure and pooling of blood in the expanded pulmonary bed, causing decreased blood pressure. HR remains stable.
•Phase IV: A systemic overshoot of blood pressure over baseline volume, likely due to return of left ventricular preload and reflex sympathetic activity in response to the hypotension of phase III [74].
In the second stage, bearing down while pushing causes changes to BP and HR that are comparable to those seen in the four phases of the Valsalva maneuver above. Active pushing has been associated with a 30% increase in HR, and 50% increase in CO [75]. Pushing in the left lateral position blunts these responses [76]. It follows that in women with known or previously unrecognized cardiac disease, these fluctuations in hemodynamics could overwhelm the cardiovascular system. Good-quality evidence proving this theory is lacking; for example, in a 2016 study that assessed the length of the second stage in 73 women with cardiac disease, 34% of women pushed longer than recommended, with no adverse cardiac events reported in the entire cohort [77]. Recommendations on whether a patient should be allowed to bear down or should have an assisted second stage must be individualized, and more evidence is needed before definitive guidelines can be put in place [75].
Clinical Implications
There are two main concerns with the cardiovascular changes during Valsalva maneuver.
1.Increase in arterial shear stress
2.Fluctuations in pre-load
The rapid rate of blood pressure increase from stage III to stage IV causes a great increase in momentary arterial shear stress which can be detrimental in patients with arterial pathology. Fluctuations in ventricular preload during Valsalva are especially worrisome in women with preload-dependent lesions.
Delivery and the Postpartum Period
The most marked hemodynamic changes occur at the moment of delivery itself and in the immediate postpartum period. CO increases up to 80% at delivery, then begins to decline to pre-labor values within approximately 1 hour following delivery [41]. Increased CO may be secondary to the return of blood supply from the uterus to the systemic circulation that leads to an increase in stroke volume [78]. Other factors that may contribute to this increase include increased venous return after relief of the weight of the gravid uterus and auto transfusion from uterine involution. An increase in CO may serve to combat the blood loss experienced at delivery. CO rapidly decreases for the first 2 weeks following delivery, and over the course of the postpartum period returns to pre-pregnancy values. Complete normalization of cardiac output can take up to 6 months postpartum [41].
Heart rate has been shown to decrease in the first 48 hours postpartum [9]. By 2 weeks after delivery, heart rate reaches its nadir and does not decrease further. In the 48 hours after delivery, stroke volume increases by 10% and then decreases steadily until up to 6 months postpartum. Blood pressure tends to remain stable throughout this period, except in those patients who have lost a clinically significant amount of blood [79].
Mode of delivery can have an impact on maternal hemodynamics. For example, blood volume decreases by 10% in the first 1–10 hours postpartum in women who had a vaginal delivery, but by 17%–29% in women who underwent cesarean section. Clinical significance of this difference is unclear as there is no difference in postoperative hematocrits between the two groups. Blood volume continues to decrease over the next 10 days in patients who had a vaginal delivery, but remains stable after the initial blood loss in those who have had a cesarean section. At 3 days postpartum, both groups tend to have an overall 10% lower blood volume than pre-delivery [69,80,81].
In more recent literature, CO was found to increase throughout cesarean section, peak within 2 minutes after delivery of newborn and placenta, and reach levels lower than baseline at 24–36 hours postoperatively [82]. This is likely secondary to the rapid increase in venous return and preload after delivery of the fetus and placenta. SVR and mean arterial pressures reach their lowest point immediately after delivery and return to their baseline levels by the time of fascial closure [83].
Analgesia (mild sensory block to prevent pain) and anesthesia (dense sensory and motor block to permit surgical stimulation) have known, relatively predictable influences on maternal hemodynamics. Spinal anesthesia for cesarean section, which creates a sensory and motor block to the level of T4, will lead to profound hypotension due to a decrease in SVR and a compensatory increase in CO; however, anesthesiologists routinely augment SVR with vasopressors (most commonly phenylephrine) to reverse this sympathetic block. Women who undergo cesarean section with an epidural already in place generally remain hemodynamically stable, with a slight decrease in BP that can be counteracted with phenylephrine; HR and CO generally remain unchanged [84]. It should be noted that slow neuraxial anesthesia via an epidural catheter allows time for the anesthesiologist to augment SVR with vasopressors so as to prevent significant maternal hemodynamic alterations due to anesthesia.
Distinguishing Physiology from Pathology
Many of the symptoms of normal pregnancy can mimic those of cardiovascular disease. A systematic approach to any pregnant or postpartum woman noting cardiac symptoms is essential so that symptoms of concern prompt appropriate workup, and normal findings do not lead to a barrage of unnecessary tests and procedures.
Symptoms
The common symptoms that can present in both normal pregnancy and in pregnancy affected by cardiac disease include decreased exercise tolerance, shortness of breath, fatigue, chest pain, and peripheral edema.
•Shortness of breath: Pregnant women will often complain of breathlessness or dyspnea and decreased exercise tolerance. While traditionally attributed to displacement of the diaphragm and chest wall by the growing uterus, some women experience breathlessness early in pregnancy. Hyperventilation seen in normal pregnancy may be perceived as shortness of breath by some women [85]. Older studies have found that up to 76% of women complain of dyspnea by the 31st week of pregnancy [86]. A low level of fatigue and decreased exercise tolerance are extremely common, though pregnant women should be able to perform their everyday activities and a low level of exercise without issue. Symptoms that should always prompt further investigation include additional symptoms listed in Table 3.1 [87].
Physiologic versus Pathologic Dyspnea | |
Favors Physiologic | Favors Pathologic |
Gradual onset | Acute onset |
No other symptoms | Presence of other symptoms Cough Chest pain Fever Hemoptysis Paroxysmal nocturnal dyspnea Orthopnea Wheezing |
First or second trimester | Third trimester |
Normal vital signs | Vital sign abnormalities |
Clear lungs auscultation | Wheezes or crackles |
Normal labs and imaging studies | Abnormalities in: BMP, LFTs, CBC BNP ECG Echocardiogram Chest x-ray CT angiogram |
Abbreviations: BMP: basic metabolic panel; BNP: brain natriuretic peptide; CBC: complete blood count; CT: computed tomography; ECG: electrocardiogram; LFTs: liver function tests. |
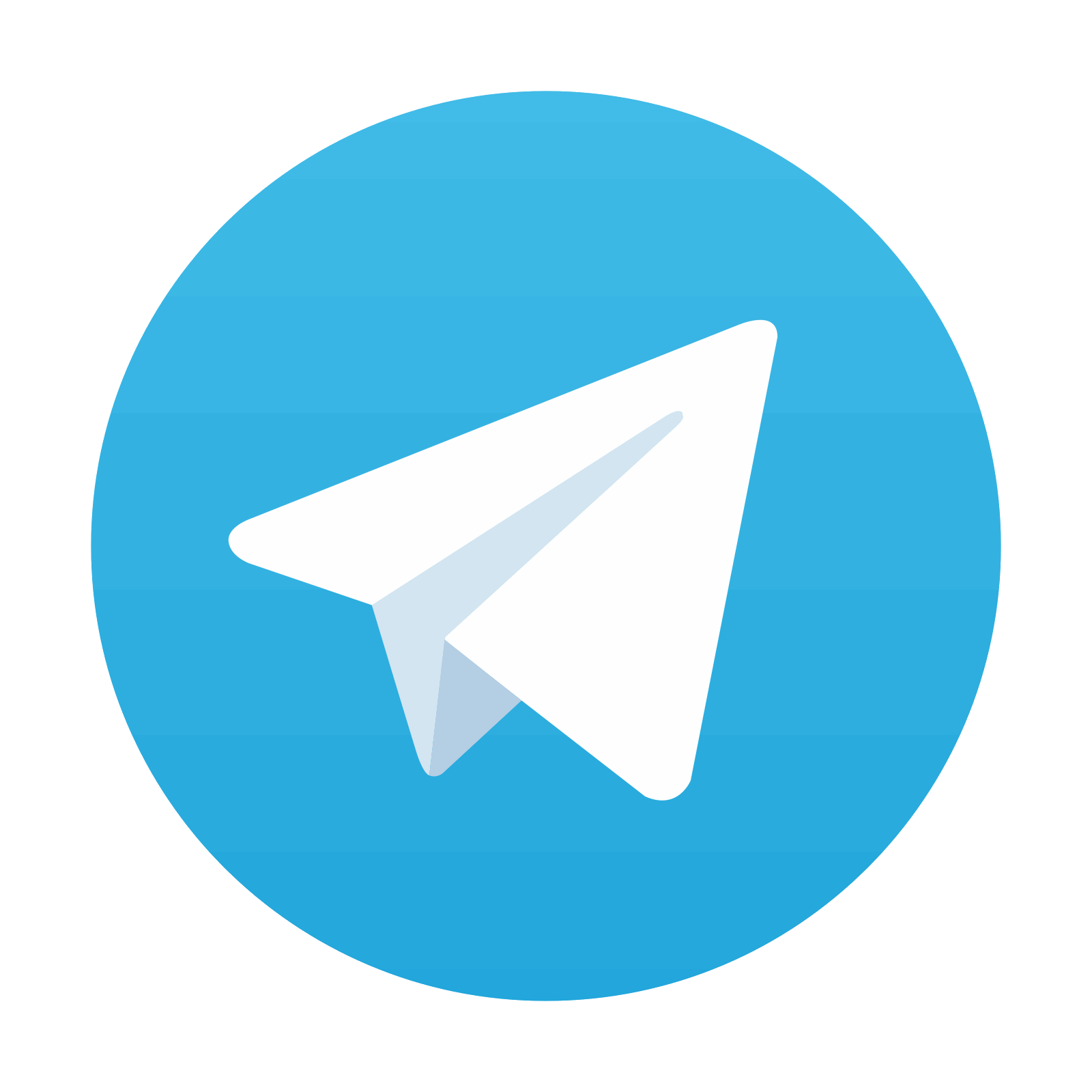
Stay updated, free articles. Join our Telegram channel

Full access? Get Clinical Tree
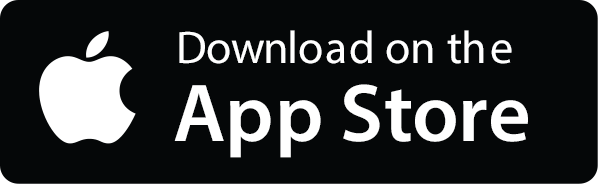
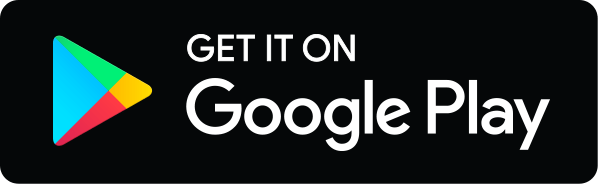