Competitive immunoassay. The Y-axis is the % of Bound/Bound (B/B) at 0% concentration of unlabeled antigen, while the X-axis is the concentration of unlabeled antigen. From Abnova (Taiwan) Corporation at www.abnova.com.
The immunometric assay is characterized by having two or more antibodies, which are directed to different epitopes (antigenic sites) on the target antigen. One of these antibodies is coupled to a solid matrix, while the other antibody is labeled with a signal system, such as an enzyme, fluorescent tag, or chemiluminescent compound. These two antibodies form a “sandwich” with the target antigen caught between the two antibodies. The signal, which is generated by the labeled antibody, can then be detected by the appropriate detector, whether that is radioactivity or luminescence [12]. In this type of assay, there is a direct relationship between the amount of signal and target antigen in the sample (Figure 17.2).
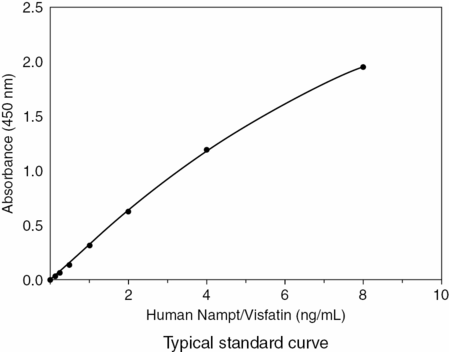
Standard curve for an immunometric (sandwich) assay. From Creative Diagnostics at www.creative-diagnostics.com.
Mass spectrometry
Mass spectrometry (MS) is a detector system for chromatographic systems which allows one to further evaluate a molecule in terms of its molecular weight and structure, and consequently is able to specifically detect a molecule based on its molecular structure [13]. Gas chromatography–mass spectrometry (GC-MS) is considered the gold standard for evaluating and determining the structure of steroids and their metabolites. However, GC-MS requires prolonged run times and is a complex process, making it difficult to adapt to high throughput systems, such as those needed in clinical laboratories. A large amount of effort has been put into improving MS over the past years to make it more user friendly for the clinical laboratory. Recent refinements in the use of high-pressure liquid chromatography–tandem mass spectrometry (HPLC-MS/MS) have improved the clinical applicability of this technology, by maintaining the sensitivity and specificity of MS with the high throughput needed by the clinical laboratory. HPLC is primarily used to purify and separate the compound of interest from interfering substances. For testosterone, usually a reverse-phase C18 and C8 column are used with water as the polar phase and methanol or acetonitrile as the organic phases. Another method to enhance sensitivity of the assay, especially if there is a small volume, is to use a derivatization procedure to improve ionization and fragmentation. Hydroxylamine is often used as the derivatization agent for testosterone, producing testosterone oxime. Since MS works in vacuum conditions, once the sample is chromatographed the solvent must be removed with ionization of the analyte. Ionization occurs by a process of atmospheric pressure ionization, which generates ions that maintain the structure of the molecule. This ionization process facilitates separation by the MS technique of the steps of ionization from fragmentation of the molecule of interest, which allows for a specific determination of molecular weight and structure of the compound.
Bioassays: in vitro
Bioassays are unique in that they can determine the hormonal activity within a sample, regardless of the structure of the compounds within that sample. Because these assays measure the total activity within a sample, one can also measure anti-hormonal activity within the sample. These assays are indispensable in the search for environmental agents with hormonal activity as well as the biological activity of new “doping agents.” These assays may play a future role in the clinical evaluation of male hypogonadism as well as in women and children with signs and symptoms of hyperandrogenism.
These assays use cells or cell lines, often genetically modified, as the primary basis for the assay technique. They are used as a screening tool to evaluate a test sample for biological activity and are improved over in vivo bioassays, with better sensitivity, specificity, higher cost effectiveness, and the potential for a higher volume of samples. However, they do have a number of limitations: they are unable to monitor the in vivo metabolism of a substance because some of the cells used in the assay may metabolize the target substance, altering its biological activity, and the cells used may have different co-regulators than the cells of interest, resulting in variable responses.
The in vitro bioassays can be divided into three major categories: receptor binding assays, cell proliferation assays, and reporter gene assays. Receptor binding assays have been developed to measure compounds that specifically bind to a receptor of interest, which in androgen assays is the androgen receptor [14]. These assays involve combining the sample of interest, labeled receptor ligand, and the receptor. The amount of bound, labeled ligand displaced by the unknown sample corresponds with the receptor binding activity of the unknown.
In the cell proliferation assay, one measures the degree of cellular proliferation that results from addition of an unknown sample to the cell culture. This has been best developed for screening of estrogenic activity in a substance using MCF-7 cells, also known as the E-SCREEN assay. Similar types of assay have been developed for androgens, one a prostate cell line and another MCF-7 cell line transfected with the human androgen receptor. These assays are relatively simple and highly sensitive, but there is a good deal of variation between batches [15].
Reporter gene assays are designed to measure hormonal activity in an unknown sample by measuring the response of a hormone-dependent reporter gene. These types of assay have been developed for measuring serum androgen activity, and are accomplished by transfecting a mammalian cell line or yeast strain with the hormonal receptor of interest as well as a reporter gene. The reporter gene is composed of a promoter, which contains hormone response elements for the hormone receptor of interest coupled to the reporter gene. When an unknown with the hormone of interest is added to this mixture, it binds to the hormone receptor, the ligand–receptor complex then binds to the hormone response elements and activates the reporter gene. With activation, the reporter gene activates transcription and translation of an enzyme such as luciferase or chloramphenicol acetyl transferase. This enzyme then converts the substrate in the mixture into a signal, which can be measured by luminometry, spectrophotometry, or fluorimetry [16]. Reporter gene assays using mammalian cells are becoming more common and are being developed for use with steroids, specifically androgens.
Androgen assays
There are a number of significant challenges in measuring testosterone levels, including the wide range of concentrations between prepubertal children, women, and adult men. Another important aspect is that testosterone secretion follows a circadian rhythm, with highest concentration in the morning and decreasing throughout the day. When measuring testosterone, it is important to consider that only 1–2% of this steroid circulates in a free, biologically active form. There are also other circulating steroids that closely resemble testosterone and may interfere with the assay. Furthermore, there is no universally accepted testosterone standard and validated normal ranges are wanting.
Total testosterone
The gold standard for measuring total testosterone is GC-MS. However, GC-MS is a time-consuming, labor-intensive method limiting its applicability in a high-volume clinical setting. Initial immunoassays involved extraction and chromatography prior to the assay. These initial steps improved accuracy and sensitivity by removing interfering proteins, separating out cross-reacting steroids, and allowing for the processing of a large sample to increase sensitivity. Once again, these initial steps of extraction and chromatography were labor-intensive, cumbersome, and time-consuming, which limits their clinical applicability. To meet the need for high-throughput and speed of turnaround, many direct immunoassays have been developed for measuring total testosterone. While these assays meet the clinical demand for a rapid, high-throughput assay, they have limited accuracy, especially for lower testosterone levels (<300 ng/dL). This is a severe limitation, since the need for a sensitive and accurate assay is to assist in differentiating between pathology and physiology, which for testosterone is differentiating between normal and low levels of the analyte [17,18].
The College of American Pathologists (CAP) has a Quality Control program where they distribute a blinded sample to clinical laboratories, which allows clinical laboratories to compare the accuracy of their assays to other laboratories using the same technology. Previous studies have demonstrated unacceptable variation with large coefficients of variation (c.v.) for lower concentrations, with improvement in variability with increasing testosterone levels. For normal female androgen levels, the c.v. ranged between 13% and 32%, while for the hypogonadal male or androgenized female, there was improvement, but still significant variability in the assays. This large variability significantly limits the use of total testosterone measurement in hypogonadal males and females. These assays had the best reproducibility in measuring ranges of testosterone in eugonadal males. In studies comparing MS to immunoassay of testosterone, most immunoassays overestimated the testosterone level [19,20].
Free testosterone
The gold standard for measuring free testosterone is with equilibrium dialysis. In this method, labeled 3H-testosterone is added to the unknown sample, equilibrium is allowed to occur across a semipermeable membrane, the bound and free 3H-testosterone are separated, and the free portion measured. The total testosterone level is then multiplied by the free fraction of 3H-testosterone to obtain the free testosterone level. Another similar method is ultrafiltration of the sample. These methods are relatively cumbersome, expensive, can be influenced by absorption of analyte to the membrane or by temperature, and are highly dependent on the measurement of total testosterone [21].
Direct immunoassay measurements of free testosterone are attractive in the clinical setting, because of their speed, ease of use, and adaptability to high throughput; however, they have been found to be clinically unreliable with a high variability, poor accuracy, and poor sensitivity. These assays have not been found to be helpful in clinical or research settings [22].
SHBG testosterone can be precipitated by ammonium sulfate, allowing one to measure bioavailable testosterone (free and albumin bound). This assay is performed by adding 3H-testosterone to the unknown sample, precipitating SHGB-testosterone, and measuring the free 3H-testosterone. One can then calculate the bioavailable testosterone by multiplying total testosterone by the non-precipitated 3H-testosterone. This assay is relatively simple for the laboratory, but can be influenced by the degree of precipitation and impurities in the tracer [23].
Other methods for estimating free testosterone include calculations based on the concentration of total testosterone and SHBG, the law of mass action, or empirical equations. The free androgen index (FAI) is calculated by multiplying the quotient testosterone/SHBG by the total testosterone level. This calculation is highly dependent on the accuracy and sensitivity of the assays used to determine total testosterone and SHBG. The FAI has good correlation with equilibrium dialysis measurements of free testosterone in women, but correlates poorly with the same measurements in males. Other calculations for determining free testosterone include algorithms based on the law of mass action and empirical equations using computer modeling [24].
Mass spectrometry
MS after extraction and chromatography allows for the highly accurate measurement of testosterone. The initial extraction and separation phases of GC-MS have increased the time and cost, and made it a labor-intensive assay. However, improvements in HPLC-MS/MS have improved the clinical applicability of this technique, including ease and high throughput. A number of recent studies have focused on using this technology to develop standard ranges for various populations.
Clinical use
The clinical use of testosterone assays depends on a highly accurate, sensitive assay that is reproducible. Before diagnosing a male as hypogonadal it is also important to obtain multiple samples, at least three, between 8 a.m. and 10 a.m. due to the circadian rhythm of testosterone secretion.
In adult males, testosterone measurement is used to differentiate between eugonadism and hypogonadism. Most clinical assays are accurate and sensitive enough to differentiate between these two states in the male. If a level is close to the lower limit of normal, then a calculated free level may be helpful in evaluating the patient.
In adult females, most assays are able to differentiate between the normal range and hyperandrogenism. However, these same assays are not accurate enough to quantify the degree of hyperandrogenism. Free testosterone levels, including the FAI, correlate well with the degree of hyperandrogenism. Current assays usually are not informative in detecting hypoandrogenism in adult females.
When measuring total testosterone in children, both boys and girls, it is vital to have sufficiently sensitive assays with appropriate normal ranges. Currently, these assays are of limited value in children.
Immunoassay interference
There are a number of factors that can interfere with an assay, including exogenous issues, which are not inherent to the specimen, and endogenous factors. Exogenous factors include pre-analytical factors and the assay formulation, while endogenous factors include those inherent to the specimen and can vary between patients and over time (Table 17.1).
Exogenous factors | Endogenous factors |
---|---|
Time Age Circadian rhythm Menstrual cycle Pregnancy | Protein bound Affinity alterations (familial dysalbuminemia) Lower concentrations |
Physiological state Infection Hematologic status Immune status Fasting Exercise, stress, and posture | Matrix of assay pH Ionic strength Protein concentration |
Drugs In vivo Assay | Cross-reacting substances Endogenous Exogenous (drugs) |
Type of specimen Blood Plasma Serum Saliva Urine | Antibodies Heterophile antibodies Human anti-animal antibodies Autoantibodies Rheumatoid factor |
Test tube Glass or plastic Serum separator test tube | High-dose hook effect |
Specimen collection Hemolysis Lipemia |
Exogenous factors
Pre-analytical factors can have an impact on analyte levels, as well as their interpretation. By strict adherence to patient preparation as well as specimen collection and handling, one can maximize clinical interpretation.
Examples of potential immunoassay interference by exogenous factors include the time of day the specimen was drawn (cortisol), the age of the patient (DHEA-S), the phase of the menstrual cycle (progesterone), and whether the patient is pregnant (human chorionic gonadotropin [hCG]). One example commonly seen in reproductive endocrinology, which illustrates the importance of patient preparation, is measurement of 17-hydroxyprogesterone. 17-Hydroxyprogesterone is often drawn in the patient suspected of having polycystic ovary syndrome to exclude the diagnosis of late-onset congenital adrenal hyperplasia due to 21-hydroxylase deficiency. If the sample is inadvertently obtained in the luteal phase, it will be elevated along with progesterone due to production by the normal corpus luteum. This example highlights the importance of interpreting results based on the physiology of the endocrine gland of interest [25].
The physiological state of the patient at the time the sample was obtained can have a significant impact upon the results. Some conditions which could influence the analyte of interest include an ongoing infection, hematologic and immunologic status, posture, fasting versus fed state, exercise, and stress. By appropriately preparing the patient prior to obtaining the sample, one can minimize this type of interference [26].
Drugs can affect an assay by having in vivo effects on the patient as well as in vitro effects upon the assay itself. Numerous drugs can alter the in vivo environment and affect the results of an assay. A common example of an in vivo effect is that of oral contraceptive pills, which increase the production of a number of circulating binding proteins. This increase in binding proteins can raise the total concentration of an analyte, but not change the free concentration of the hormone (like thyroxine). Drugs can also interact directly with the assay and influence the result; this is especially important where drugs can cross-react in steroid assays [27,28].
The type of specimen collected and prepared for use is also an important pre-analytical aspect of the assay, given that many assays are designed for a specific type of sample, including whole blood, plasma, serum, urine, or saliva. Whole blood is rarely used in immunoassays, owing to the presence of cellular enzymes. These enzymes from whole blood may rapidly destroy the analyte of interest, with one pertinent example being ACTH. For this reason, when planning to measure these unstable analytes, the sample must be collected with special precautions, such as the addition of antiproteolytic agents, rapid centrifugation, and freezing of the sample. Plasma is another type of sample that is often collected and used in the clinical laboratory. When collecting a sample as plasma, it is important to fill the test tube to the manufacturer’s predetermined level, otherwise there can be leftover chelating agent, which may interfere. The chelating agent may interfere with the antigen–antibody reaction of the immunoassay, resulting in spurious results. Serum is the matrix of choice for many immunoassays, as it is stable and easily stored. One important exception to the rule for serum is parathyroid hormone, which is rapidly destroyed in serum. Parathyroid hormone needs to be collected in an EDTA test tube and frozen within 30 minutes of collection [29,30].
The type of test tube in which the sample is collected can also influence the results of the assay. Gel serum separator tubes can interfere with numerous peptide and protein assays. For steroid assays, progesterone is reduced by 50% when stored on a gel serum separator test tube for 6 days. In the past, tris(2-butoxyethyl)phosphate was used as a plasticizer but it can inhibit the binding of certain drugs to proteins. Another important example is ACTH, which will bind tightly to glass test tubes resulting in spurious results [31].
While collecting a sample, hemolysis can have a large impact upon certain assays. With hemolysis, there is a release of cellular enzymes that can metabolize a number of peptide hormones and alter their measurement. If one is measuring a substance which has a higher concentration within the cell, then hemolysis will release the analyte of interest and artificially increase levels. An example of hemolysis falsely elevating levels is folate and potassium. A lipemic sample can also interfere with a number of assays, either by directly interfering with a turbidimetric endpoint or displacing a ligand from its binding site [32].
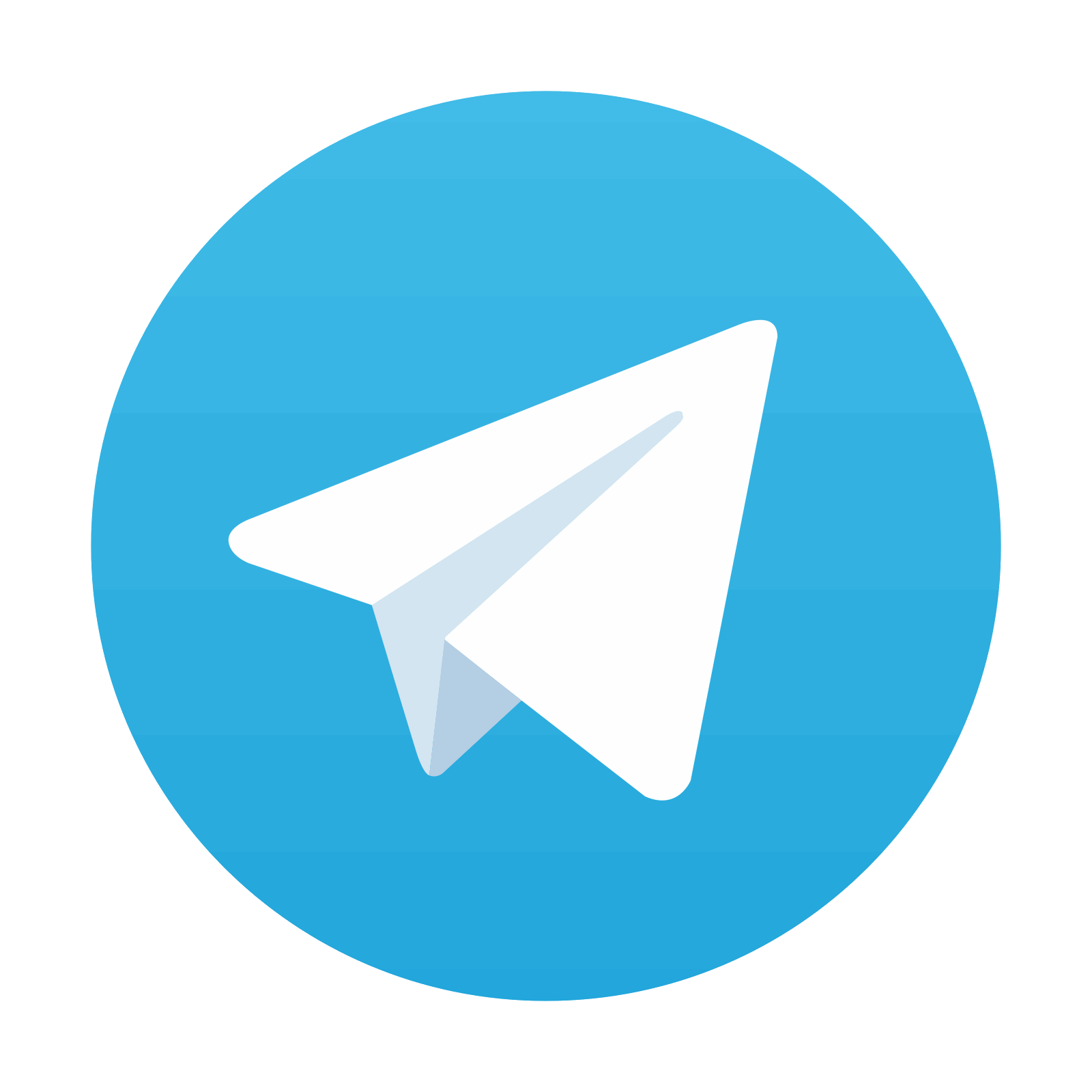
Stay updated, free articles. Join our Telegram channel

Full access? Get Clinical Tree
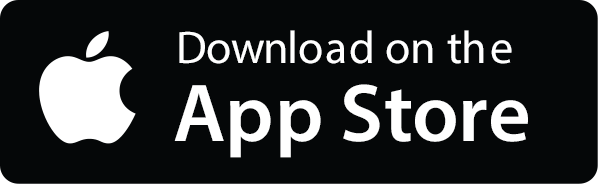
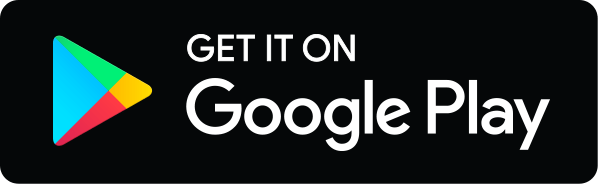